Controlled automated reperfusion of the whole body after cardiac arrest
Introduction
The high incidence of acute circulatory arrest (CA), accompanied by high mortality and neurological sequelae in the few survivors, represents an ongoing challenge in healthcare (1). Regardless of the cause of CA, cardiopulmonary resuscitation (CPR) has been the treatment of choice for decades to counteract the life-threatening loss of circulation and respiratory function. However, during CPR, major challenges are arising, like (I) that the underlying cause of CA cannot be treated on site and (II) the absence of a reestablished spontaneous circulation, despite maximized efforts of emergency medical services. As a consequence of this dilemma and despite extensive effort in research and education of CPR in recent years, the outcome of affected patients in terms of survival and neurological recovery has been unsatisfactory over decades (1-3).
“Targeted CPR” (tCPR) is a relatively new concept, where during the resuscitative efforts specific hemodynamic, respiratory and metabolic targets are defined (4-7). Among others, compression depth of cardiac massage, arterial blood pressure, end-tidal CO2 measurement and the titration of oxygen are core elements of tCPR (4,8). Although tCPR represents a logical step towards a rationale based and controlled therapeutic approach in CPR, due to a significant lack of available appropriate monitoring and related therapeutic interventions, the implementation of tCPR remains rudimentary (9).
At the same time, extracorporeal circulatory life support systems (ECLS) during CPR [extracorporeal CPR (eCPR)] have seen an increased use in recent years.
Here, similar limitations, as with tCPR, apply. Although blood circulation and respiratory function may quickly be replaced by ECLS, the lack in readily available monitoring leads to non-targeted treatment (10). Consequently, despite the additional time for the detection and therapy of treatable causes of CA, survival and neurological recovery of patients treated with eCPR has only improved to a limited extent (10-12). This framework of an insufficient therapeutic approach to a major healthcare problem promotes research and evolvement of new ideas in order to provide better survival and neurological recovery of affected patients. For this reason, the subsequently described concept of “controlled automated reperfusion of the whole body” (CARL) has been developed over the past 15 years.
Methods
Ischemia/reperfusion injury (IRI) has been a topic of a continued research for decades. At its core is the fact, that deprivation of blood flow (ischemia) causes a time-dependent cellular damage mainly related to a lack of required substrates (13). Reestablishing blood flow (reperfusion) to a former ischemic area primarily provides a new supply of nutrients for cellular metabolism. However, the extent of cellular damage is even increasing during the phase of reperfusion (Figure 1). This effect of continued cellular damage is related to a disparity of actual demand and supply of the damaged cells during reperfusion. Moreover, reperfusion injury is triggered within brief periods of seconds or minutes following the reinstitution of blood flow to the ischemic tissue, therefore adding the requirement of a timely intervention of counteractive measures. The comprehension of these mechanisms and the derived therapeutic options limiting IRI has become an established part of therapeutic regimen in cardiac surgery, solid organ transplantation and limb reperfusion (14-17).
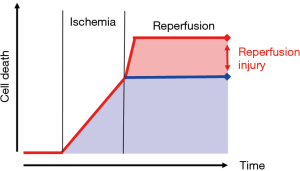
Core elements within the therapeutic approach to limit IRI are (I) gaining control over the physical conditions of reperfusion (i.e., blood pressure, blood flow, pulsatility, body temperature) and (II) the constitution of the reperfusion solution (recirculating blood), i.e., oxygen content, carbon dioxide level, pH, electrolyte content, osmolality (Table 1) (18-26). As mentioned above, readily available and ample monitoring plays a key role in any therapeutic approach in these acute and critical sick patients. Therefore, extent and demand of suitable monitoring had been defined parallel to the conditions of reperfusion and the constitution of the recirculating blood within the CARL therapy. As a consequence of these demands, online and immediate blood gas analysis and arterial blood pressure monitoring have been implemented in the CARL setting, providing the treating team elementary information as baseline for a rationale-based targeted limitation of IRI, rather than an uncontrolled symptomatic therapy of CA.
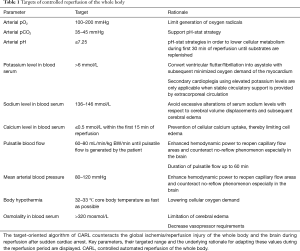
Full table
Since the entity of CA is frequently associated with primarily myocardial pump failure, an extracorporeal circulation was chosen to take over basic circulatory and respiratory function within the therapeutic approach of CARL (Table 2). The treatment of IRI in different organs potentially requires different reperfusion conditions and solutions and may lead to contrary requirements. Exemplary for conflicting priorities in a setting of whole body reperfusion is the element “arterial blood pressure” in myocardial and cerebral reperfusion, with targeted gentle blood pressures during myocardial reperfusion and comparable high blood pressure demand in cerebral reperfusion (26,27). Based on this background and acknowledging such conflicting demands, the concept of CARL has been primarily drafted on established elements of limiting IRI. Moreover, and apart from the focus of current CPR, the severe impact of a generalized IRI of the whole body and the specific demand of the not replaceable brain had been primarily addressed in the concept of CARL. Finally the global concept of CARL and its single elements were tested and further developed in numerous chronic animal experiments over the last 15 years (18-24,28).
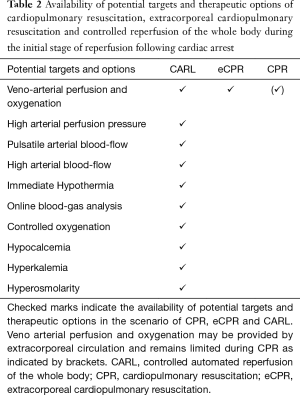
Full table
The unique chronic animal model was established to investigate beneficial components in a setting of global reperfusion after severe ischemia with respect to the endpoints mortality and neurological recovery (21). The animals were exposed to periods of 15 and 20 minutes of unprotected warm ischemia, excluding any resuscitative attempts during this time (18,21,22). The following reperfusion period of 60 minutes was characterized by adapting the reperfusion conditions and the circulating blood according to the continuous and readily available monitoring (i.e., blood gas monitoring, arterial blood pressure, temperature measurement, mixed venous oxygen saturation etc.). Depending on the tested variable, up to 90% of the animals survived the experimental course with up to 90% of them indicating a complete neurological recovery (18,21-24).
In synopsis of the given publications in single organs and the results of the described animal experiments incorporating the global approach of CARL, a set of targets during the initial stage of reperfusion have been identified as beneficial. Continuous blood gas analysis is the starting point for a reasonable adaption of parameters like paO2, PCO2, pH, sodium, potassium and calcium. Beyond that, the presence of a continuous arterial blood pressure monitoring and blood flow allows a targeted adaption according to the individual needs of the patient up to the selection of approved infusion solutions supporting the global concept of CARL.
Medical device for the application of CARL
The described implementation of CARL required a suitable medical device in order to meet the aforementioned requirements of ample and readily available monitoring and a subsequent control of the conditions of reperfusion and the adaption of the recirculating blood. Since such a device was not available, a system configuration “Controlled Integrated Resuscitation Device” (CIRD 1.0; Resuscitec GmbH, Freiburg, Germany) was engineered and approved by the regulatory authorities. CIRD 1.0 is based on an ECLS and contains extended functionality, such as online blood gas monitoring, controlled oxygen supply, high and pulsatile blood flow and an appropriate cooling device for induction of hypothermia (Figure 2). This system configuration has been used to supply CARL therapy patients with acute CA und subsequent prolonged CPR.
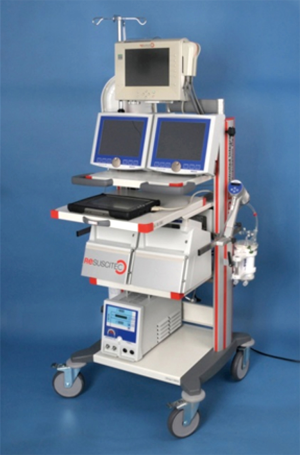
Results
The aforementioned elements of a targeted and rationale-based management of extracorporeal circulation were summarized in a standard operating procedure (SOP) for hospital-based treatment. This document provides guidance for the team members during the highly interactive situation of continued CPR when the demand for extracorporeal support is arising.
The current expertise of patients treated with CARL comprises an uncontrolled and consecutive series of 14 patients with prolonged CPR following witnessed CA (unpublished data). In all cases, the interdisciplinary teams decided in an intention-to-treat situation on the application of extracorporeal circulation using the CIRD 1.0 device. Therefore, all 14 patients received the monitored, rationale based and targeted therapeutic approach of CARL. The results of this approach were similar to those of the preclinical experiments. Despite the extremely prolonged preceding periods of CPR between 51 and 120 minutes, 7 of 14 surviving patients regained full consciousness while 6 of these 7 were assigned to Cerebral Performance Class (CPC) “1”. This complete cerebral recovery also applied to one patient of this series with the most extended preceding period of 120 minutes CPR, who returned to her former work despite a remaining paresis of the legs due to an arteria spinalis anterior syndrome (29).
Conclusions
For decades, CA followed by CPR has been associated with very high mortality and frequent neurological sequelae in the few survivors. The current method of circulatory support in these situations dates back to the early 1960s, when external cardiac massage and defibrillation was invented. It presents a symptomatic therapy that aims towards a jump start of the just failing heart, so as to provide circulation. CPR supported by extracorporeal circulation (eCPR) has become more important and more widely accepted in recent years, as it might allow a better survival and neurological recovery by temporary takeover of circulation and gas exchange. However, the results of eCPR are still controversial. Limited improvements in survival and frequent neurological impairment of resuscitated patients are still reported.
Under these premises, there is growing evidence, that a severe and generalized IRI occurs during and after CPR and eCPR, causing severe harm in affected patients and leading to an unsatisfying morbidity and mortality (30-32). For this reason, 15 years back we focused our research onto the limitation of this generalized IRI of the whole body and the brain. The therapeutic CARL approach represents an evolved method that aims towards a limitation of a generalized IRI and follows the current highly demanded concept of tCPR (4,18,22,26). CARL allows for a rationale-based, personalized and comprehensive therapy based on a readily available set of monitoring data and diagnostic findings.
Our demand regarding a suitable medical device for the implementation of CARL was solved by assembling a system configuration, which allowed the application of CARL in 14 patients with prolonged CA. First experiences in these patients showed promising results with superior neurological recovery in the 50% survivors. Yet, these results can only contribute a little piece of evidence to the wide field of understanding and counteracting IRI following CA.
Acknowledgments
Christina Süßlin and Gabriele Lechner for all effort and support towards the preparation of this manuscript.
Footnote
Conflicts of Interest: G Trummer, C Benk and F Beyersdorf are shareholder of Resuscitec GmbH. G Trummer and C Benk received salaries for part-time employment.
Ethical Statement: This study was approved by the Ethics Committee of Albert-Ludwig University Freiburg/Germany, No. 86/14 (§23b MPG) on May 15th, 2014. Written informed consent was obtained from the patient for publication and any accompanying images.
References
- Soar J, Nolan JP, Böttiger BW, et al. European Resuscitation Council Guidelines for Resuscitation 2015: Section 3. Adult advanced life support. Resuscitation 2015;95:100-47. [Crossref] [PubMed]
- Kim YJ, Ahn S, Sohn CH, et al. Long-term neurological outcomes in patients after out-of-hospital cardiac arrest. Resuscitation 2016;101:1-5. [Crossref] [PubMed]
- Ha TS, Yang JH, Cho YH, et al. Clinical outcomes after rescue extracorporeal cardiopulmonary resuscitation for out-of-hospital cardiac arrest. Emerg Med J 2017;34:107-11. [Crossref] [PubMed]
- Maciel CB, Barden MM, Greer DM. Neurological Recovery After Cardiac Arrest: a Multifaceted Puzzle Requiring Comprehensive Coordinated Care. Curr Treat Options Cardiovasc Med 2017;19:52. [Crossref] [PubMed]
- Friess SH, Sutton RM, French B, et al. Hemodynamic directed CPR improves cerebral perfusion pressure and brain tissue oxygenation. Resuscitation 2014;85:1298-303. [Crossref] [PubMed]
- Hayes MM, Berg RA, Otto CW. Monitoring during cardiac arrest: are we there yet? Curr Opin Crit Care 2003;9:211-7. [Crossref] [PubMed]
- Kleinman ME, Perkins GD, Bhanji F, et al. ILCOR Scientific Knowledge Gaps and Clinical Research Priorities for Cardiopulmonary Resuscitation and Emergency Cardiovascular Care: A Consensus Statement. Resuscitation 2018;127:132-46. [Crossref] [PubMed]
- Munshi L, Kiss A, Cypel M, et al. Oxygen Thresholds and Mortality During Extracorporeal Life Support in Adult Patients. Crit Care Med 2017;45:1997-2005. [Crossref] [PubMed]
- Nunes LB, Mendes PV, Hirota AS, et al. Severe hypoxemia during veno-venous extracorporeal membrane oxygenation: exploring the limits of extracorporeal respiratory support. Clinics 2014;69:173-8. [Crossref] [PubMed]
- Ortega-Deballon I, Hornby L, Shemie SD, et al. Extracorporeal resuscitation for refractory out-of-hospital cardiac arrest in adults: A systematic review of international practices and outcomes. Resuscitation 2016;101:12-20. [Crossref] [PubMed]
- Han KS, Kim SJ, Lee EJ, et al. Experience of extracorporeal cardiopulmonary resuscitation in a refractory cardiac arrest patient at the emergency department. Clin Cardiol 2019;42:459-66. [Crossref] [PubMed]
- Beyea MM, Tillmann BW, Iansavichene AE, et al. Neurological outcomes after extracorporeal membrane oxygenation assisted CPR for resuscitation of out-of-hospital cardiac arrest patients: A systematic review. Resuscitation 2018;130:146-58. [Crossref] [PubMed]
- Garcia-Dorado D. Piper. Postconditioning: Reperfusion of "reperfusion injury "after hibernation. Cardiovasc Res 2006;69:1-3. [Crossref] [PubMed]
- Buckberg GD. When is cardiac muscle damaged irreversibly? J Thorac Cardiovasc Surg 1986;92:483-7. [PubMed]
- Haab F, Julia P, Nochy D, et al. Improvement of postischemic renal function by limitation of initial reperfusion pressure. J Urol 1996;155:1089-93. [Crossref] [PubMed]
- Beyersdorf F, Unger A, Wildhirt A, et al. Studies of reperfusion injury in skeletal muscle: preserved cellular viability after extended periods of warm ischemia. J Cardiovasc Surg (Torino) 1991;32:664-76. [PubMed]
- Halldorsson AO, Kronon M, Allen BS, et al. Controlled reperfusion prevents pulmonary injury after 24 hours of lung preservation. Ann Thorac Surg 1998;66:877-84; discussion 884-5. [Crossref] [PubMed]
- Foerster K, Benk C, Beyersdorf F, et al. Twenty minutes of normothermic cardiac arrest in a pig model: the role of short-term hypothermia for neurological outcome. Perfusion 2018;33:270-7. [Crossref] [PubMed]
- Kreibich M, Trummer G, Beyersdorf F, et al. Improved Outcome in an Animal Model of Prolonged Cardiac Arrest Through Pulsatile High Pressure Controlled Automated Reperfusion of the Whole Body. Artif Organs 2018;42:992-1000. [Crossref] [PubMed]
- Liakopoulos OJ, Allen BS, Buckberg GD, et al. Resuscitation after prolonged cardiac arrest: role of cardiopulmonary bypass and systemic hyperkalemia. Ann Thorac Surg 2010;89:1972-9. [Crossref] [PubMed]
- Trummer G, Foerster K, Buckberg GD, et al. Successful resuscitation after prolonged periods of cardiac arrest: A new field in cardiac surgery. J Thorac Cardiovasc Surg 2010;139:1325-32, 1332.e1-2.
- Taunyane IC, Benk C, Beyersdorf F, et al. Preserved brain morphology after controlled automated reperfusion of the whole body following normothermic circulatory arrest time of up to 20 minutes. Eur J Cardiothorac Surg 2016;50:1025-34. [Crossref] [PubMed]
- Foerster K, D’Inka M, Beyersdorf F, et al. Prolonged cardiac arrest and resuscitation by extracorporeal life support: favourable outcome without preceding anticoagulation in an experimental setting. Perfusion 2013;28:520-8. [Crossref] [PubMed]
- Trummer G, Foerster K, Buckberg GD, et al. Superior neurologic recovery after 15 minutes of normothermic cardiac arrest using an extracorporeal life support system for optimized blood pressure and flow. Perfusion 2014;29:130-8. [Crossref] [PubMed]
- Allen BS, Ko Y, Buckberg GD, et al. Studies of isolated global brain ischaemia: III. Influence of pulsatile flow during cerebral perfusion and its link to consistent full neurological recovery with controlled reperfusion following 30 min of global brain ischaemia. Eur J Cardiothorac Surg 2012;41:1155-63. [Crossref] [PubMed]
- Allen BS, Ko Y, Buckberg GD, et al. Studies of isolated global brain ischaemia: II. Controlled reperfusion provides complete neurologic recovery following 30 min of warm ischaemia - the importance of perfusion pressure. Eur J Cardiothorac Surg 2012;41:1147-54. [Crossref] [PubMed]
- Okamoto F, Allen BS, Buckberg GD, et al. Reperfusion conditions: importance of ensuring gentle versus sudden reperfusion during relief of coronary occlusion. J Thorac Cardiovasc Surg 1986;92:613-20. [PubMed]
- Allen BS, Castellá M, Buckberg GD, et al. Conditioned blood reperfusion markedly enhances neurologic recovery after prolonged cerebral ischemia. J Thorac Cardiovasc Surg 2003;126:1851-8. [Crossref] [PubMed]
- Trummer G, Supady A, Beyersdorf F, et al. Controlled automated reperfusion of the whole body after 120 minutes of Cardiopulmonary resuscitation: first clinical report. Scand J Trauma Resusc Emerg Med 2017;25:66. [Crossref] [PubMed]
- Nolan JP, Neumar RW, Adrie C, et al. Post-cardiac arrest syndrome: epidemiology, pathophysiology, treatment, and prognostication. A Scientific Statement from the International Liaison Committee on Resuscitation; the American Heart Association Emergency Cardiovascular Care Committee; the Council on Cardiovascular Surgery and Anesthesia; the Council on Cardiopulmonary, Perioperative, and Critical Care; the Council on Clinical Cardiology; the Council on Stroke. Resuscitation 2008;79:350-79. [Crossref] [PubMed]
- Neumar RW, Nolan JP, Adrie C, et al. Post-cardiac arrest syndrome: epidemiology, pathophysiology, treatment, and prognostication. A consensus statement from the International Liaison Committee on Resuscitation (American Heart Association, Australian and New Zealand Council on Resuscitation, European Resuscitation Council, Heart and Stroke Foundation of Canada, InterAmerican Heart Foundation, Resuscitation Council of Asia, and the Resuscitation Council of Southern Africa); the American Heart Association Emergency Cardiovascular Care Committee; the Council on Cardiovascular Surgery and Anesthesia; the Council on Cardiopulmonary, Perioperative, and Critical Care; the Council on Clinical Cardiology; and the Stroke Council. Circulation 2008;118:2452-83. [Crossref] [PubMed]
- Jou C, Shah R, Figueroa A, et al. The Role of Inflammatory Cytokines in Cardiac Arrest. J Intensive Care Med 2018. [Epub ahead of print]. [Crossref] [PubMed]