Left ventricular assist devices as a bridge to cardiac transplantation
Introduction
Despite advances in medical technology and renewed interest in preventative health care, heart failure remains a significant cause of morbidity and mortality in the United States. Partially because of the improvements in the treatment of heart failure, more patients are living with advanced stage heart failure than ever before. By 2010, over five million Americans carried a diagnosis of heart failure with another 825,000 patients receiving the diagnosis in that year alone (1). The standard of care for end stage heart failure patients remains cardiac transplantation in patients deemed to be appropriate candidates. However, the disparity between available donor hearts and recipients waiting on the cardiac transplant list has continued to grow. With the advent of durable and reliable mechanical circulatory support (MCS), bridge to transplant (BTT) therapy has become the standard of care for many patients awaiting transplant who develop end-stage organ dysfunction or a life threatening exacerbation of their existing heart failure (2-4).
Background
Early efforts at MCS focused on short term, extra corporeal assist devices. As technology improved throughout the 1990s, durable and implantable assist devices were introduced that revolutionized the surgical treatment of heart failure refractory to medical intervention. While cardiac transplantation remains the gold standard, the availability of viable options for long-term mechanical support in patients with advanced stage heart failure ushered in the current era of MCS as a bridge to cardiac transplantation.
Initially conceived as an alternative to cardiac transplantation, the benefit and viability of left ventricular assist devices (LVAD) as a BTT was demonstrated in the landmark study conducted by Frazier et al. in 1995. In this nonrandomized study comparing implantation of an early model pulsatile LVAD versus optimal medical therapy, a 55% reduction in pre-transplant mortality was seen with LVAD support and a significant improvement in 1 year post-transplant survival in patients supported with a pulsatile LVAD (2). In addition, the Randomized Evaluation of Mechanical Assistance for the Treatment of Congestive Heart Failure (REMATCH) trial demonstrated that LVAD implantation improved survival compared to optimal medical management in patients ineligible for heart transplantation (5).
Despite promising results, the early implantable mechanical circulatory devices, which were of the pulsatile, volume displacement variety, did not have the durability required to provide ongoing, long-term support without frequent LVAD pump exchanges (5). An unacceptably high rate of device infection or malfunction requiring LVAD exchange [up to 65% by 2 years of support by one study (6)] limited widespread acceptance and use of this first generation, pulsatile LVADs. In addition, they were large, limiting their use in women, adolescents, and some men. The development of second generation continuous flow (CF), rotary pump devices [Heartware VAD (HVAD) (Figure 1), Heartmate II (Figure 2)] that were smaller, quieter, and more durable allowed for longer durations of support and the ability to implant LVADs in underserved patient populations.
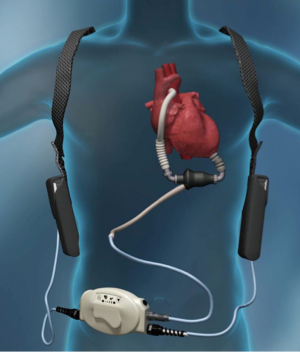
In the current era, with continued improvements in LVAD technology, improved patient selection, and post-operative clinical optimization, patients implanted with ventricular assist devices are designated as BTT, meant to act as a mechanical assist in the ambulatory setting as a bridge to cardiac transplantation, or destination therapy (DT), meant as a permanent solution for patients not deemed to be transplant candidates. While the BTT and DT designations allow for the categorizing of patients, helping standardize patient populations for outcomes based studies, in reality patients may crossover from one group to the other as their clinical condition deteriorates or improves, or other medical co-morbidites cause a once transplant-eligible patient to remain on indefinite MCS. In fact, the term “bridge to decision” has become common at many institutions, wherein a patient is implanted with an LVAD and the decision to for eventual cardiac transplantation UNOS listing is determined by their clinical course in the months following implantation. In addition, as devices have improved and experience with MCS become more extensive, a subset of patients have been found to recover myocardial function after temporary LVAD support (8), eventually allowing for explanation of the LVAD, leading these patients to be referred to as “bridge to recovery”. In the end, it is important to recognize that a patient’s initial designation as BTT or DT is not a diagnosis but part of an ongoing clinical assessment of the patient’s functional status and response to LVAD therapy.
Patient selection
Patient selection is critical for optimal outcomes after LVAD implantation. Patients being considered for MCS must first have all modifiable or reversible causes of heart failure optimally treated medically prior to surgical consideration of LVAD implantation. Additionally, their transplant eligibility should be assessed to determine type and timing of LVAD implantation. Generally, patients with a high one-year mortality from heart failure, those who are inotrope dependent, or those who are otherwise unable to maintain end-organ function and are not expected to recover without long term MCS should be considered for LVAD placement (9). New York Heart Association functional class should be assessed and Interagency Registry for Mechanical Assisted Support (INTERMACS) profiles should be determined. Additionally, cognitive and psychosocial testing, as well as family/community support networks must be considered when determining patient eligibility for LVAD implantation. In general, patients with irreversible multi-organ dysfunction are not eligible for LVAD implantation.
Currently, optimal timing for implantation of an LVAD remains unclear. However, many centers believe that in transplant eligible patients’ early implantation, prior to the development of end organ dysfunction and inotrope dependence, improves outcomes, quality of life, and survival to transplant. Pre-operative risk assessment scores remain imperfect but have highlighted risk factors, such as pre-existing renal dysfunction, liver dysfunction, poor nutritional status, and coagulopathy, which have adverse effects on patient outcomes post-VAD implantation (10), helping to identify patients who may not benefit from an LVAD.
Contemporary results
Device selection and outcomes
Outcomes in patients supported by LVADs have continued to consistently improve over time with improvement in device design, patient selection, and post-operative care.
CF-LVADs appear to have excellent outcomes when used as a BTT (11) as measured by survival to transplant, cardiac recovery, or ongoing LVAD support at six months. In the contemporary era, improvements in LVAD technology and durability have led to an increasing use of LVAD as a BTT with alternative therapeutic options becoming increasingly obsolete. Supporting patients with intravenous inotropes in order to delay LVAD implantation is becoming increasingly rare. Improvements in survival, device durability, and reduced adverse events have led many centers to eliminate inotropic therapy as an alternative to LVADs.
Following the US Food and Drug Administration’s (FDA) approval of the Thoratec Heartmate II CF-LVAD as a BTT in 2008, improvements in survival have continued to increase. One year survival from the initial 133 patients reported by Miller et al. in 2007 was 68% (11). By 2009, this number had climbed to 73% one year survival (12), and by 2011, the one year survival reported by INTERMACS from the postapproval study had climbed to 85% (13) (see Figure 3). Most importantly, patients with CF-LVADs were noted to have improved survival compared with patients supported by pulsatile flow devices (15).
Another CF-LVAD that has demonstrated similar outcomes and improvements in quality of life metrics is the Heartware Ventricular Assist System. HVAD, which utilizes centrifugal flow technology rather than the axial flow design seen in the Heartmate II, is designed with an integrated inflow cannula meant to allow for complete intra-pericardial implantation. The HVAD has consistently demonstrated excellent survival outcomes (16,17), with the ADVANCE trial reporting 86% survival at one year post-implant with a significant improvement in functional capacity and quality of life (17). Compared with optimal medical therapy, the improvements seen in 6-minute walk times was nearly three times better for patients implanted with the HVAD (17,18), further highlighting the dramatic improvements that can be seen with CF-LVAD implantation in heart failure patients.
The Jarvik 2000 is yet another example of a second generation, CF LVAD which utilizes axial flow, but is unique in that it uses a single, vanned impeller and its pump is positioned intraventricularly with the outflow graft most commonly anastomosed to the descending aorta, rather than the ascending aortic outflow graft commonly used with the Heartmate II and HVAD. This novel design allows for the possibility of less invasive implantation, through subcostal or thoracotomy incisions, and in some cases, without the need for cardiopulmonary bypass (19,20). These techniques have been reported to decrease need for intraoperative blood transfusions and intensive care unit (ICU) length of stay in patients with a prior sternotomy (19,21,22). Although several single-center and multi-center studies have indicated that the Jarvik 2000 provides safe, effective MCS for heart failure patients (21,23-30) which in some cases compared favorably with Heartmate II recipients (21) these studies’ authors also indicated that the Jarvik 2000 functions optimally as an adjunct to native left ventricular function, with only partial unloading of the ventricle. Thus, although clinical experience indicates the ability of the Jarvik 2000 to provide full cardiac output if necessary (7,23,24), its maximal clinical benefit lies in its ability to augment left ventricular function and preserving as much native function as possible, which limits its application in some heart failure patients being considered for LVAD implantation.
More recently, CF-LVAD outcomes have continued to improve as our collective experience implantation and post-operative care has grown (Table 1). A retrospective multi-center analysis comparing patients implanted with a Heartmate II LVAD after FDA approval in commercial use with the results from the clinical trial reported a consistent improvement in outcomes (14), with an increase in the percentage of patients who were either transplanted, explanted, or receiving ongoing LVAD support at 6 months and 1 year in the posttrial cohort. Similarly, recently reported figures from patients implanted with the HVAD have also shown remarkable improvements in outcomes, with survival now exceeding 90% at 1 year (31). In fact, the increased utilization of CF-LVADs, along with their excellent long-term outcomes, have led to a decrease in the number of patients transplanted in the first year, from 48% during the Heartmate II BTT clinical trial to only 39% during the post-trial period (14). In this same period, one year survival on the heart transplant waiting list actually increased despite the decreased transplantation rate. This indicates an increase in the number and proportion of patients awaiting heart transplantation after LVAD implantation, further highlighting the feasibility and reliability of extended LVAD support.
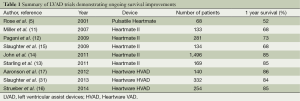
Full table
As results in patients receiving LVADs as a BTT have continued to improve, even rivaling the results seen in heart transplant recipients (32), a national discourse has begun on whether to re-evaluate the current status criteria for listing patients implanted with a LVAD on the United Network for Organ Sharing (UNOS) for cardiac transplantation. However, an equally compelling question is how LVAD implantation impacts heart transplant outcomes. Previously reported outcomes have been conflicting with regard to the impact LVAD implantation has on post-cardiac transplantation survival (33-37). In addition, optimal duration of VAD support prior to cardiac transplantation remains unknown (38,39) with some data suggesting adverse post-transplant survival in patients who required prolonged LVAD support prior to cardiac transplant (33,35-37,40,41). However, more recent data suggests that post-transplant mortality is not adversely affected by the duration of LVAD support (34), even in patients who were supported for over one year prior to transplant. In support of this, contemporary results appear to suggest that BTT patients implanted with CF-LVADs do not have significantly different post-transplant survival rates or adverse outcomes (42).
Complications
While inherent device malfunction or failure has been virtually eliminated in the modern era with the development of reliable CF devices, major adverse events continue to be a significant concern. Bleeding, infection, stroke, malignant arrhythmias, and pump thrombosis continue to be valid concerns requiring ongoing monitoring and vigilance. Perhaps surprisingly given the improvements in patient survival and lessons learned regarding the management of CF-LVAD patients in the modern era, the percentage of patients who required upgrading to status 1A because of an LVAD-related complication has not changed significantly since its introduction and remains at almost 30% of patients on LVAD support (43).
Early concerns regarding the long-term effects of low systemic arterial pulsatility on end-organ dysfunction have largely been refuted (44). Nevertheless, the lack of pulsatility in CF-LVAD devices does pose some unique challenges in the management of patients in the clinical setting and may be responsible for some of the changes in vascular endothelial function and tone observed in patients supported with CF LVADs.
The decrease in pulse pressure observed in CF-LVADs is primarily related to augmentation of diastolic blood flow. Due to the continuous unloading of the left ventricle with CF-LVADs, increased blood flow during diastole leads to an increase in diastolic blood pressure. Pump speed settings must be carefully monitored and adjusted to provide the appropriate unloading of the left ventricle. Pump speed settings that are high may result in collapse of the left ventricle, leading to a “suck-down” effect on the ventricle, causing obstruction of the inflow cannula and, potentially, malignant ventricular arrhythmias. In addition, continuous unloading of the left ventricle can potentially lead to decreased aortic valve opening and increased trans-aortic pressure gradients. Aortic insufficiency has been observed in patients requiring a prolonged duration of support, with increased frequency and severity reported when the aortic valve remains closed for prolonged periods (45-48).
The lack of pulsatility in CF devices also appears to cause histologic changes in the endothelium. Disruption of the renin-angiotensin system, altered responses to vasopressors, and medial arterial wall thickening with changes in the smooth muscle and elastin content have all been demonstrated as consequences of decreased pulsatility (49-52). These changes may be responsible for the reported increase in hemodynamic compromise observed in patients on prolonged CF device support, as demonstrated by an increase in the required dose and duration of press or support after cardiac transplantation (53).
Additionally, gastrointestinal bleeding [occurring in up to 40% of CF LVAD patients (54)] appears to be at least partially caused by the lack of pulsatility, thought to be responsible for the formation of angiodysplasias and arteriovenous malformations. First reported in 2005 by Letsou et al. (55), the association of gastrointestinal bleeding with CF-LVADs remains a concern. Further study to determine the etiology of this phenomenon led some observant investigators to notice a similarity to a physiologic state observed in patients with aortic stenosis (56). Aortic stenosis patients, who also exhibit a narrow pulse pressure similar to that seen in patients on CF-LVAD support, are thought to develop an “acquired von Willebrand disease” leading to gastrointestinal bleeding episodes (57). The finding that has garnered the most attention is the loss of large von Willebrand factor (vWF) multimers, much like Heyde syndrome as described in patients with critical aortic stenosis (58). Veyradier et al. (59) noted a high rate of von Willebrand disease in non-VAD patients with bleeding angiodysplasia and proposed that this deficiency was particularly pertinent at the very high shear conditions related to these malformations. In sharp contrast to heart transplant recipients, Geisen et al. (60) first reported loss of large vWF multimers in patients supported with Heartmate II and pulsatile VADs despite amounts of vWF antigen that were comparable to that seen in heart transplant recipients. Taken together, the evidence of acquired von Willebrand disease in the CF-VAD population is compelling and likely a key contributor to the pathophysiology of gastrointestinal bleeding. However, the fact that not all CF-VAD patients experience bleeding complications implies that other factors are also critical.
Finally, infectious complications continue to be an important risk factor for long-term survival (61). In fact, a recent UNOS analysis found that device-related infectious complications led to decreased post-transplant mortality (62). Therefore, prevention strategies, including patient education and aggressive, early treatment of driveline infections, are paramount. As trauma to the percutaneous lead exit site may be a potential cause of many device-related infections, preventative stabilization of the lead is an important prevention strategy to minimize post-operative infectious risk.
Future directions
When the clinical trials with CF LVADs began just over a decade ago, there was concern over the uncertainty of the long-term effects of arterial blood flow with low pulsatility (63). However, the large amount of cumulative experience with CF-VADS indicates that long-term support does not carry detrimental effects on organ function (44). Although it appears that the reliability issues of the past have been largely addressed with the newer CF-LVADs, some adverse events continue to limit the overall effectiveness of the technology. Although infection rates have decreased with the smaller LVADs now being implanted, infection continues to be an important risk factor for long-term survival (62). Aggressive strategies for prevention and treatment of infection need to be refined. Because antibiotic-resistant organisms are the frequent source of many device-related infections (61), preventative measures are likely to have the greatest impact on infection rates. For long durations, a totally implantable LVAD with a transcutaneous energy transmission system may offer the best option for preventing infection (64).
Experience from the clinical trials and post-trial studies with CF pumps have yielded several additional lessons about patient selection, perioperative management, operative technique, and long-term management of these patients. Looking forward, there are several areas of potential progress in our efforts to reduce the risks of bleeding and thrombosis in patients with contemporary CF-VADs. The availability of oral direct thrombin inhibitors is one such opportunity. Preliminary studies in other populations have suggested an acceptable and potentially improved safety profile compared with vitamin K antagonists (65), but the higher risk of bleeding in CF-VAD patients coupled with the difficulty of prompt reversal of these agents must be carefully considered. Finally, noninvasive analytics such as acoustic signature analysis may provide an opportunity to diagnose pump thrombosis earlier in the course of development (66), when aggressive anticoagulation may be sufficient to prevent progression and the need for surgical pump exchange.
Future generation devices close to clinical trials include Thoratec’s HeartMate III and Heartware’s MVAD Pump. The Heartmate III is a CF centrifugal pump that is magnetically levitated pump that may have a more favorable blood pump contact profile (67). In addition to increased miniaturization compared to the HM II, the HM III features improved flow dynamics which may limit shear forces compared to current CF-LVADs (68). The MVAD pump is a continuous axial flow pump, approximately one-third the size of the HVAD Pump. The MVAD Pump is based on the same proprietary “contactless” impeller suspension technology used in the HVAD Pump, with its single moving part held in place through a combination of passive-magnetic and hydrodynamic forces. The MVAD Pump is designed to support a wide range of flows to enable both full and partial support capability.
Conclusions
CF-LVAD has become the therapeutic standard for management of advanced heart failure in patients awaiting heart transplantation with excellent outcomes reported which continue to improve over time. Initial pulsatile flow LVADs had significant device related complications and device failure, limiting its widespread acceptance. The significant early mortality risk [up to 30% in the first three weeks (69)] led to a UNOS cardiac allocation policy allowing for 1A status for thirty days following LVAD implantation, and permanent 1B status thereafter. However, with improvements in LVAD outcomes, as well data that suggested increased mortality in LVAD-bridged transplant patients receiving transplants within 1 month after pulsatile LVAD implant (38,39,69), the policy was modified in 2002 to allow for thirty days of 1A status at any timepoint after LVAD implantation at the physician’s discretion (69,70). In the contemporary era, outcomes have improved to the point that patients supported with CF-LVADs as a BTT have similar mortality risk as patients listed as status 2 and may even have improved survival (43).
Complications related to LVAD implantation have improved as well, but remain a significant cause for concern. While non-device related adverse events from CF-LVADs appear to occur at lower rates than in their pulsatile counterparts (12), the risk is hardly eliminated and complications specific to CF-LVADs are now being seen. It appears the highest risk for complications occurs peri-operatively, with a rapid decrease within two months after LVAD implantation (43).
Our institution’s experience with CF-LVAD compare favorably to those reported at other centers (71). Similar to results published from other groups nationally, we have observed significant improvements in baseline hemodynamics (42) and excellent survival outcomes, both in patients receiving ongoing LVAD support as well as after cardiac transplant (42,71). In addition, while gastrointestinal bleeding remains a concern in patients implanted with CF-LVADs at our institution, very few devices have required replacement due to device thrombosis, malfunction, or infection and no mechanical failures have been reported (71). As our center’s experience approaches 300 patients implanted with the Heartmate II LVAD, the reliability, durability, and improvements in survival and quality of life in heart failure patients implanted with CF-LVADs, have provided us with a viable surgical strategy for the growing number of patients awaiting heart transplantation.
The increased acceptance and utilization of CF-LVADs will likely lead to continued improvement in patient outcomes through increased clinical experience and device design. Although LVAD-related complications requiring status 1A listing continues to occur at a high rate, contemporary results for BTT patients on LVAD support may lead to revision of the current UNOS allocation policy regarding cardiac transplantation to assure the most equitable and appropriate priority for listing of heart transplant eligible patients.
Acknowledgements
Dr. Ranjit John receives research grant support from Thoratec and Heartware.
Disclosure: The authors declare no conflict of interest.
References
- Go AS, Mozaffarian D, Roger VL, et al. Executive summary: heart disease and stroke statistics--2014 update: a report from the American Heart Association. Circulation 2014;129:399-410. [PubMed]
- Frazier OH, Rose EA, McCarthy P, et al. Improved mortality and rehabilitation of transplant candidates treated with a long-term implantable left ventricular assist system. Ann Surg 1995;222:327-36; discussion 336-8. [PubMed]
- Farrar DJ, Hill JD, Pennington DG, et al. Preoperative and postoperative comparison of patients with univentricular and biventricular support with the thoratec ventricular assist device as a bridge to cardiac transplantation. J Thorac Cardiovasc Surg 1997;113:202-9. [PubMed]
- Frazier OH, Rose EA, Oz MC, et al. Multicenter clinical evaluation of the HeartMate vented electric left ventricular assist system in patients awaiting heart transplantation. J Thorac Cardiovasc Surg 2001;122:1186-95. [PubMed]
- Rose EA, Gelijns AC, Moskowitz AJ, et al. Long-term use of a left ventricular assist device for end-stage heart failure. N Engl J Med 2001;345:1435-43. [PubMed]
- Dembitsky WP, Tector AJ, Park S, et al. Left ventricular assist device performance with long-term circulatory support: lessons from the REMATCH trial. Ann Thorac Surg 2004;78:2123-9; discussion 2129-30. [PubMed]
- John R. Current axial-flow devices--the HeartMate II and Jarvik 2000 left ventricular assist devices. Semin Thorac Cardiovasc Surg 2008;20:264-72. [PubMed]
- Birks EJ, Tansley PD, Hardy J, et al. Left ventricular assist device and drug therapy for the reversal of heart failure. N Engl J Med 2006;355:1873-84. [PubMed]
- Feldman D, Pamboukian SV, Teuteberg JJ, et al. The 2013 International Society for Heart and Lung Transplantation Guidelines for mechanical circulatory support: executive summary. J Heart Lung Transplant 2013;32:157-87. [PubMed]
- Lietz K, Long JW, Kfoury AG, et al. Outcomes of left ventricular assist device implantation as destination therapy in the post-REMATCH era: implications for patient selection. Circulation 2007;116:497-505. [PubMed]
- Miller LW, Pagani FD, Russell SD, et al. Use of a continuous-flow device in patients awaiting heart transplantation. N Engl J Med 2007;357:885-96. [PubMed]
- Pagani FD, Miller LW, Russell SD, et al. Extended mechanical circulatory support with a continuous-flow rotary left ventricular assist device. J Am Coll Cardiol 2009;54:312-21. [PubMed]
- Starling RC, Naka Y, Boyle AJ, et al. Results of the post-U.S. Food and Drug Administration-approval study with a continuous flow left ventricular assist device as a bridge to heart transplantation: a prospective study using the INTERMACS (Interagency Registry for Mechanically Assisted Circulatory Support). J Am Coll Cardiol 2011;57:1890-8. [PubMed]
- John R, Naka Y, Smedira NG, et al. Continuous flow left ventricular assist device outcomes in commercial use compared with the prior clinical trial. Ann Thorac Surg 2011;92:1406-13; discussion 1413. [PubMed]
- Slaughter MS, Rogers JG, Milano CA, et al. Advanced heart failure treated with continuous-flow left ventricular assist device. N Engl J Med 2009;361:2241-51. [PubMed]
- Strueber M, Larbalestier R, Jansz P, et al. Results of the post-market Registry to Evaluate the HeartWare Left Ventricular Assist System (ReVOLVE). J Heart Lung Transplant 2014;33:486-91. [PubMed]
- Aaronson KD, Slaughter MS, Miller LW, et al. Use of an intrapericardial, continuous-flow, centrifugal pump in patients awaiting heart transplantation. Circulation 2012;125:3191-200. [PubMed]
- Lindenfeld J, Feldman AM, Saxon L, et al. Effects of cardiac resynchronization therapy with or without a defibrillator on survival and hospitalizations in patients with New York Heart Association class IV heart failure. Circulation 2007;115:204-12. [PubMed]
- Frazier OH, Gregoric ID, Cohn WE. Initial experience with non-thoracic, extraperitoneal, off-pump insertion of the Jarvik 2000 Heart in patients with previous median sternotomy. J Heart Lung Transplant 2006;25:499-503. [PubMed]
- Selzman CH, Sheridan BC. Off-pump insertion of continuous flow left ventricular assist devices. J Card Surg 2007;22:320-2. [PubMed]
- Sorensen EN, Pierson RN 3rd, Feller ED, et al. University of Maryland surgical experience with the Jarvik 2000 axial flow ventricular assist device. Ann Thorac Surg 2012;93:133-40. [PubMed]
- Sun BC, Firstenberg MS, Louis LB, et al. Placement of long-term implantable ventricular assist devices without the use of cardiopulmonary bypass. J Heart Lung Transplant 2008;27:718-21. [PubMed]
- Frazier OH, Myers TJ, Gregoric ID, et al. Initial clinical experience with the Jarvik 2000 implantable axial-flow left ventricular assist system. Circulation 2002;105:2855-60. [PubMed]
- Frazier OH, Myers TJ, Westaby S, et al. Use of the Jarvik 2000 left ventricular assist system as a bridge to heart transplantation or as destination therapy for patients with chronic heart failure. Ann Surg 2003;237:631-6; discussion 636-7. [PubMed]
- Frazier OH, Myers TJ, Westaby S, et al. Clinical experience with an implantable, intracardiac, continuous flow circulatory support device: physiologic implications and their relationship to patient selection. Ann Thorac Surg 2004;77:133-42. [PubMed]
- Haj-Yahia S, Birks EJ, Rogers P, et al. Midterm experience with the Jarvik 2000 axial flow left ventricular assist device. J Thorac Cardiovasc Surg 2007;134:199-203. [PubMed]
- Myers TJ, Robertson K, Pool T, et al. Continuous flow pumps and total artificial hearts: management issues. Ann Thorac Surg 2003;75:S79-85. [PubMed]
- Siegenthaler MP, Frazier OH, Beyersdorf F, et al. Mechanical reliability of the Jarvik 2000 Heart. Ann Thorac Surg 2006;81:1752-8; discussion 1758-9.
- Siegenthaler MP, Westaby S, Frazier OH, et al. Advanced heart failure: feasibility study of long-term continuous axial flow pump support. Eur Heart J 2005;26:1031-8. [PubMed]
- Westaby S, Banning AP, Saito S, et al. Circulatory support for long-term treatment of heart failure: experience with an intraventricular continuous flow pump. Circulation 2002;105:2588-91. [PubMed]
- Slaughter MS, Pagani FD, McGee EC, et al. HeartWare ventricular assist system for bridge to transplant: combined results of the bridge to transplant and continued access protocol trial. J Heart Lung Transplant 2013;32:675-83. [PubMed]
- Williams ML, Trivedi JR, McCants KC, et al. Heart transplant vs left ventricular assist device in heart transplant-eligible patients. Ann Thorac Surg 2011;91:1330-3; discussion 1333-4. [PubMed]
- Cleveland JC Jr, Grover FL, Fullerton DA, et al. Left ventricular assist device as bridge to transplantation does not adversely affect one-year heart transplantation survival. J Thorac Cardiovasc Surg 2008;136:774-7. [PubMed]
- John R, Pagani FD, Naka Y, et al. Post-cardiac transplant survival after support with a continuous-flow left ventricular assist device: impact of duration of left ventricular assist device support and other variables. J Thorac Cardiovasc Surg 2010;140:174-81. [PubMed]
- Morgan JA, Park Y, Kherani AR, et al. Does bridging to transplantation with a left ventricular assist device adversely affect posttransplantation survival? A comparative analysis of mechanical versus inotropic support. J Thorac Cardiovasc Surg 2003;126:1188-90. [PubMed]
- Patlolla V, Patten RD, Denofrio D, et al. The effect of ventricular assist devices on post-transplant mortality an analysis of the United network for organ sharing thoracic registry. J Am Coll Cardiol 2009;53:264-71. [PubMed]
- Robertson JO, Lober C, Smedira NG, et al. One hundred days or more bridged on a ventricular assist device and effects on outcomes following heart transplantation. Eur J Cardiothorac Surg 2008;34:295-300. [PubMed]
- Ashton RC Jr, Goldstein DJ, Rose EA, et al. Duration of left ventricular assist device support affects transplant survival. J Heart Lung Transplant 1996;15:1151-7. [PubMed]
- Gammie JS, Edwards LB, Griffith BP, et al. Optimal timing of cardiac transplantation after ventricular assist device implantation. J Thorac Cardiovasc Surg 2004;127:1789-99. [PubMed]
- Aaronson KD, Eppinger MJ, Dyke DB, et al. Left ventricular assist device therapy improves utilization of donor hearts. J Am Coll Cardiol 2002;39:1247-54. [PubMed]
- Jaski BE, Kim JC, Naftel DC, et al. Cardiac transplant outcome of patients supported on left ventricular assist device vs. intravenous inotropic therapy. J Heart Lung Transplant 2001;20:449-56. [PubMed]
- Kamdar F, John R, Eckman P, et al. Postcardiac transplant survival in the current era in patients receiving continuous-flow left ventricular assist devices. J Thorac Cardiovasc Surg 2013;145:575-81. [PubMed]
- Wever-Pinzon O, Drakos SG, Kfoury AG, et al. Morbidity and mortality in heart transplant candidates supported with mechanical circulatory support: is reappraisal of the current United network for organ sharing thoracic organ allocation policy justified? Circulation 2013;127:452-62. [PubMed]
- Russell SD, Rogers JG, Milano CA, et al. Renal and hepatic function improve in advanced heart failure patients during continuous-flow support with the HeartMate II left ventricular assist device. Circulation 2009;120:2352-7. [PubMed]
- Cowger J, Pagani FD, Haft JW, et al. The development of aortic insufficiency in left ventricular assist device-supported patients. Circ Heart Fail 2010;3:668-74. [PubMed]
- John R, Mantz K, Eckman P, et al. Aortic valve pathophysiology during left ventricular assist device support. J Heart Lung Transplant 2010;29:1321-9. [PubMed]
- Jorde UP, Uriel N, Nahumi N, et al. Prevalence, significance, and management of aortic insufficiency in continuous flow left ventricular assist device recipients. Circ Heart Fail 2014;7:310-9. [PubMed]
- Pak SW, Uriel N, Takayama H, et al. Prevalence of de novo aortic insufficiency during long-term support with left ventricular assist devices. J Heart Lung Transplant 2010;29:1172-6. [PubMed]
- Lou X, Templeton DL, John R, et al. Effects of continuous flow left ventricular assist device support on microvascular endothelial function. J Cardiovasc Transl Res 2012;5:345-50. [PubMed]
- Nishimura T, Tatsumi E, Nishinaka T, et al. Diminished vasoconstrictive function caused by long-term nonpulsatile left heart bypass. Artif Organs 1999;23:722-6. [PubMed]
- Travis AR, Giridharan GA, Pantalos GM, et al. Vascular pulsatility in patients with a pulsatile- or continuous-flow ventricular assist device. J Thorac Cardiovasc Surg 2007;133:517-24. [PubMed]
- Westaby S, Bertoni GB, Clelland C, et al. Circulatory support with attenuated pulse pressure alters human aortic wall morphology. J Thorac Cardiovasc Surg 2007;133:575-6. [PubMed]
- Stewart AS, Russo MJ, Martens TP, et al. Longer duration of continuous-flow ventricular assist device support predicts greater hemodynamic compromise after return of pulsatility. J Thorac Cardiovasc Surg 2008;136:524-5. [PubMed]
- Stern DR, Kazam J, Edwards P, et al. Increased incidence of gastrointestinal bleeding following implantation of the HeartMate II LVAD. J Card Surg 2010;25:352-6. [PubMed]
- Letsou GV, Shah N, Gregoric ID, et al. Gastrointestinal bleeding from arteriovenous malformations in patients supported by the Jarvik 2000 axial-flow left ventricular assist device. J Heart Lung Transplant 2005;24:105-9. [PubMed]
- Heyde E. Gastrointestinal bleeding in aortic stenosis N Engl J Med 1958;259:196.
- Warkentin TE, Moore JC, Morgan DG. Aortic stenosis and bleeding gastrointestinal angiodysplasia: is acquired von Willebrand’s disease the link? Lancet 1992;340:35-7. [PubMed]
- Vincentelli A, Susen S, Le Tourneau T, et al. Acquired von Willebrand syndrome in aortic stenosis. N Engl J Med 2003;349:343-9. [PubMed]
- Veyradier A, Balian A, Wolf M, et al. Abnormal von Willebrand factor in bleeding angiodysplasias of the digestive tract. Gastroenterology 2001;120:346-53. [PubMed]
- Geisen U, Heilmann C, Beyersdorf F, et al. Non-surgical bleeding in patients with ventricular assist devices could be explained by acquired von Willebrand disease. Eur J Cardiothorac Surg 2008;33:679-84. [PubMed]
- Topkara VK, Kondareddy S, Malik F, et al. Infectious complications in patients with left ventricular assist device: etiology and outcomes in the continuous-flow era. Ann Thorac Surg 2010;90:1270-7. [PubMed]
- Healy AH, Baird BC, Drakos SG, et al. Impact of ventricular assist device complications on posttransplant survival: an analysis of the United network of organ sharing database. Ann Thorac Surg 2013;95:870-5. [PubMed]
- Thalmann M, Schima H, Wieselthaler G, et al. Physiology of continuous blood flow in recipients of rotary cardiac assist devices. J Heart Lung Transplant 2005;24:237-45. [PubMed]
- Asgari SS, Bonde P. Implantable physiologic controller for left ventricular assist devices with telemetry capability. J Thorac Cardiovasc Surg 2014;147:192-202. [PubMed]
- Salazar CA, del Aguila D, Cordova EG. Direct thrombin inhibitors versus vitamin K antagonists for preventing cerebral or systemic embolism in people with non-valvular atrial fibrillation. The Cochrane database of systematic reviews 2014;3:CD009893. [PubMed]
- Uriel N, Morrison KA, Garan AR, et al. Development of a novel echocardiography ramp test for speed optimization and diagnosis of device thrombosis in continuous-flow left ventricular assist devices: the Columbia ramp study. J Am Coll Cardiol 2012;60:1764-75. [PubMed]
- Farrar DJ, Bourque K, Dague CP, et al. Design features, developmental status, and experimental results with the Heartmate III centrifugal left ventricular assist system with a magnetically levitated rotor. ASAIO J 2007;53:310-5. [PubMed]
- Farrar DJ, Bourque K, Reichenbach SH, et al. Innovation Update. In: Kyo S. eds. Ventricular assist devices in advanced-stage heart failure. Springer, 2014.
- Renlund DG, Taylor DO, Kfoury AG, et al. New UNOS rules: historical background and implications for transplantation management. United Network for Organ Sharing. J Heart Lung Transplant 1999;18:1065-70. [PubMed]
- Moazami N, Sun B, Feldman D. Stable patients on left ventricular assist device support have a disproportionate advantage: time to re-evaluate the current UNOS policy. J Heart Lung Transplant 2011;30:971-4. [PubMed]
- John R, Kamdar F, Eckman P, et al. Lessons learned from experience with over 100 consecutive HeartMate II left ventricular assist devices. Ann Thorac Surg 2011;92:1593-9; discussion 1599-600. [PubMed]