In vitro potency of antipseudomonal β-lactams against blood and respiratory isolates of P. aeruginosa collected from US hospitals
Introduction
Pseudomonas aeruginosa is an opportunistic pathogen associated with a variety of infections ranging from simple folliculitis to sever septic shocks depending on the host immune status and severity of any underlying conditions present. As a result of its ability to adapt to variable environmental conditions as well as develop biofilms, P. aeruginosa is capable of avoiding innate immune clearance mechanisms and thus has enhanced pathogenicity (1). Moreover, P. aeruginosa flourish under selective antimicrobial pressure, are intrinsically resistance to many classes of antimicrobials and are capable of acquiring additional resistance genes from other organisms. These characteristics combined with the organisms’ ability to develop resistance using a variety of mechanisms makes P. aeruginosa a formidable pathogen in the clinical arena (1,2).
The corner stone of P. aeruginosa therapy most often involves the administration of a β-lactam antimicrobial; however, escalating resistance within this class has eroded the treatment armamentarium. In December 2014, ceftolozane/tazobactam (C/T) a β-lactam/β-lactamase inhibitor combination with antipseudomonal activity including multi-drug resistant (MDR) isolates was approved by the FDA to treat complicated urinary tract infections (cUTI) and intra-abdominal infections (IAI) (3). Recently, a phase III multicenter clinical trail completed enrollment of 726 patients with ventilated nosocomial pneumonia to assess C/T efficacy and safety in comparison with meropenem (clinicaltrials.gov, NCT 02070757).
In the current era of rapidly evolving antimicrobial resistance, studies assessing the potency of available antimicrobials are fundamental to informing decisions regarding the most appropriate choice of therapy (4,5). As well as, focused effort on using the source of isolates (i.e., respiratory versus blood) as a guide to select proper empiric therapy is an increasing demand. Thus, we assessed the in vitro potency of 7 antipseudomonal agents including C/T against P. aeruginosa collected from numerous hospitals across the US.
Methods
Consecutive non-duplicate, non-urine, respiratory or blood isolates of P. aeruginosa were obtained from adult inpatients as part of their routine medical management. Isolates were collected from 35 different hospitals across the United States, in 2017 and 2018. Organisms were identified at each participating site using methods normally employed by their laboratories and were transferred onto trypticase soy agar slants for shipping.
Once received at the central processing laboratory (Center for Anti-Infective Research and Development, Hartford Hospital, Hartford, CT, USA) isolates were transferred onto trypticase soy agar plates containing 5% sheep blood for minimum inhibitory concentration (MIC) determination. The MIC determinations for the following agents: aztreonam (ATM), C/T, cefepime (FEP), ceftazidime (CAZ), imipenem (IPM), meropenem (MEM) and piperacillin/tazobactam (TZP) were undertaken using Clinical Laboratory Standards Institute (CLSI) broth microdilution methods (6).
Merck Pharmaceuticals provided C/T, all others antibiotics were purchased from Sigma (St. Louis, MO, USA). MIC trays were prepared using the Biomek 3000 (Beckman Instruments, Inc., Fullerton, CA, USA). As recommended by CLSI, K. pneumoniae 700603 and P. aeruginosa 27853 were utilized as quality control (QC) strains; all QC values were within CLSI acceptable ranges (6). Colony counts were performed on each isolate to verify the correct inoculum. The CLSI interpretative susceptibility criteria were utilized for each agent. P. aeruginosa were classified as carbapenem non-susceptible if isolates were non-susceptible to IPM or MEM with MIC >2 mg/L. Additionally, isolates were defined as multidrug resistant (MDR) if they displayed resistance to 3 or more classes as represented by the following phenotypic resistance profiles: CIP (MIC ≥4 mg/L), IPM (MIC ≥8 mg/L), CAZ (MIC ≥32 mg/L), TZP (MIC ≥128 mg/L), and TOB (MIC ≥16 mg/L) (6).
Results
A total of 1,209 P. aeruginosa isolates were collected and tested. Antipseudomonal potency of assessed agents is presented in Table 1. The MIC for which 50% and 90% of isolates were inhibited (MIC50/90) by C/T was 0.5/2 mg/L, MEM 0.5/16 mg/L, and IPM 2/16 mg/L. The MIC distribution for all agents is displayed in Figure 1, among this population of P. aeruginosa; C/T exhibited the highest activity with 95% susceptibility, followed by CAZ (78%) and FEP (76%). All other antipseudomonal agents, consisting of representative examples across classes, demonstrated a range of 66–74% susceptibility (Table 1).
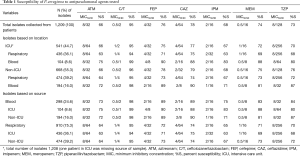
Full table
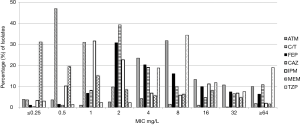
Based on location, around half (55.2%) of isolates tested were collected from patients outside the ICU. Similar %S were found in isolates collected inside and outside the ICU with C/T exhibiting the highest susceptibility in both settings. However, based on source of isolates tested, the majority of samples were from respiratory sources in both ICU and non-ICU setting. Isolates from blood samples were found more susceptible compared with isolates from respiratory samples. Additionally, isolates collected from blood samples outside ICU exhibited reduced susceptibilities compared with blood samples collected inside ICU (Table 1).
Overall, carbapenem non-susceptibility defined by IPM or MEM MIC of >2 mg/L was 35% (n=423) in the current P. aeruginosa population. Among carbapenem non-susceptible isolates, C/T showed the highest susceptibility exhibiting 89% followed by CAZ 60% and all other agents were below 54% susceptibility (Table 2). Among the assessed P. aeruginosa population, 322 isolates were TZP NS; C/T was found to have 85% susceptible with 17–39% for the remaining antipseudomonal agents tested (Table 2). Thirteen percent of isolates (n=153) were defined as MDR by reference broth microdilution methods. The degree of susceptibility among these MDR isolates for C/T was 74% whereas that of the other agents tested ranges from 5–12% (Table 2).
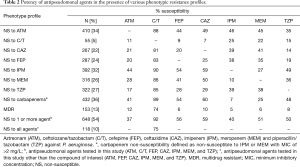
Full table
Susceptibilities varied by resistance status to 1 or more antipseudomonal versus isolates resistant to all antipseudomonal agents. P. aeruginosa resistant to 1 or more composed 54% and presented 92% susceptibility to C/T followed by 59% and 56% for CAZ and FEP, respectively. Similar to that observed in MDR subset, P. aeruginosa non-susceptible to all antipseudomonal retained 75% susceptibility to C/T. Isolates that demonstrated non-susceptibility to C/T (n=55) displayed low susceptibility rates ranging from 7–25% for the other antipseudomonal agents. Susceptibility and MIC distribution over the 9 U.S. census divisions were assessed. In all U.S. census divisions, susceptibility rates were ≥93% and ranging from 93–99% (Table 3).
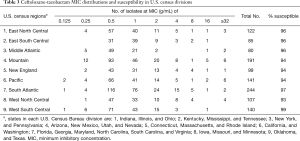
Full table
Discussion
Based on MIC90 observed in this study, C/T was at least 3 folds more active than other antipseudomonal agents tested, while its overall susceptibility was 95% in this population of P. aeruginosa. These data are in line with a previous surveillance program done by our group in 2015 where 1,257 P. aeruginosa were tested and reported C/T MIC90 was 2 mg/L, and 97% susceptibility, followed by CAZ (77%) and MEM (76%) (7). Additionally, a surveillance study testing 3,815 P. aeruginosa isolates from 32 U.S. hospitals between 2012–2015 reported 97% C/T susceptibility and MIC50/90 of 0.5/2 mg/L (8). Similarly, a recent surveillance study in 2017 with 1909 P. aeruginosa isolates collected from 70 U.S. medical centers and tested using broth micro-dilution found C/T the most active compound with 97.5% susceptibility rates (9). Moreover, a surveillance study conducted between 2007–2016 among P. aeruginosa collected from patients in ICU reported 97.5%S to C/T followed by 96.1%S to amikacin (10).
Quarter of P. aeruginosa population observed herein was from blood source, with higher susceptibilities in comparison to respiratory isolates. However, as 75% of isolates in this study were from respiratory samples and susceptibilities among these isolates are reduced in comparison with isolates from blood samples, studies similar to ASPECT-NP (clinicaltrials.gov, NCT 02070757) are needed to focus more efforts on considering source of isolates in selecting the most appropriate therapy.
Albeit the majority of isolates were collected outside ICU (55.2%), these organisms were more susceptible than those collected inside ICU. Our findings are in line with a previous study that reported 65% of P. aeruginosa were collected outside ICU with higher susceptibility observed in isolates collected outside ICU (11). These observations are of paramount importance in clinical settings, as understanding susceptibility of P. aeruginosa based on source and location of these isolates can guide providers to select the most appropriate empiric therapy in high-risk patients. Especially that P. aeruginosa was often thought of as an ICU pathogen; however, the prevalence of this pathogen is increasing outside the ICU (11).
While an overall basal assessment of potency is required for the total isolates collected, subset analysis looking at target phenotypic profiles where a novel compound of interest may be used in clinical settings is of increased relevance. Since P. aeruginosa are commonly resistance to 1 or more of the conventional β-lactams this phenotypic profile appears as a reasonable target to assess the potency of new therapies. In a recent study of 309 P. aeruginosa was resistant to 1 or more antipseudomonal β-lactams, 72.5% of the isolates were C/T susceptible (12). In our current population, 648 (54%) of the isolates were non-susceptible to 1 or more and 118 (10%) were non-susceptible to all antipseudomonal antibiotics. Despite varying phenotypic profiles to individual β-lactams, C/T demonstrated 81–90% susceptibility, which diminished to 74% and 75% when considering the MDR and NS to all agents, respectively. Similar to our previous observation, this current in vitro assessment of P. aeruginosa reveals that resistant to 1 β-lactam does not translate to resistance to the entire class and thus the testing of novel therapies in the wake of resistance to conventional agents may often yield viable treatment options (13). In infections suspected to be caused by resistant P. aeruginosa, combination therapy is the most common strategy in clinical settings. Although synergistic interactions are not confirmed to be superior over monotherapy against P. aeruginosa in clinical studies, combination therapy increases the probability of providing appropriate initial therapy by having at least one active agent against the suspected pathogen especially in cases where susceptibilities are not known (5).
Some variations in susceptibility rates to C/T were observed between U.S. census divisions. However, the overall susceptibility rates to C/T were high, ranging from 93–99% (Table 3). These observations are in line with a previous study that reported C/T susceptibility rates ranging 94–99% (8). However, these observations suggest the growing need of regional resistance rates surveillance studies.
In an attempt to initiate early appropriate therapy in the at-risk patient, data derived in the current study highlights the need for routine susceptibility testing of C/T in institutions where >20% of the P. aeruginosa are non-susceptibility to conventional β-lactams, while reflective testing schemes may be suitable in institutions with lower resistance.
Conclusions
In this P. aeruginosa population, C/T demonstrated the highest overall susceptibility (95%), while, other antipseudomonal agents including carbapenems displayed susceptibilities ranging from 66–78%. In the era of escalating P. aeruginosa resistance to conventionally utilized β-lactams, the enhanced potency of newer novel therapies should be considered in an attempt optimize both clinical and microbiologic outcomes.
Acknowledgments
We wish to acknowledge the staff of the Center for Anti-Infective Research and Development for assistance with MIC testing, Christina Sutherland for coordination of isolates collection across participating sites, and investigators at participating hospitals. Participating hospitals include: David Geffen School of Medicine at UCLA (Los Angeles, CA), Jackson Memorial Hospital (Miami, FL), University of Louisville Hospital (Louisville, KY), Barnes-Jewish Hospital at Washington University (St. Louis, MO), Geisinger Health System (Danville, PA), University of Maryland School of Medicine (Baltimore, MD), Hendrick Medical Center (Abilene, TX), Rhode Island Hospital (Providence, RI), Summa Health System (Akron, OH), Virginia Commonwealth University Medical Center (Richmond, VA), Grady Memorial Hospital (Atlanta, GA), Harborview Medical Center (Seattle, WA), University of Arizona, College of Medicine (Phoenix, AZ), Hartford Hospital (Hartford, CT), Deaconess Hospital (Evansville, IN), ARUP Laboratories (Salt Lake City, UT), St. Anthony Hospital (Oklahoma City, OK), Stanford Hospital and Clinics (Palo Alto, CA), Medical University of South Carolina (Charleston, SC), Lakeland Regional Medical Center (Lakeland, FL), Methodist Dallas Medical Center (Dallas, TX), NewYork-Presbyterian/Weill Cornell Medical Center (New York, NY), University Medical Center of S. Nevada (Las Vegas, NV), St. Joseph’s Hospital and Medical Center (Phoenix, AZ), St. Dominic-Jackson Memorial Hospital (Jackson, MS), Tufts Medical Center (Boston, MA), Northwestern University Chicago College of Pharmacy (Downers Grove, Illinois), University of New Mexico Hospital (Albuquerque, NM), University of Iowa Hospital and Clinic (Iowa City, IA), Hennepin County Medical Center (Minneapolis, MN), Anderson Cancer Center (Houston, TX), Beth Israel Deaconess Medical Center (Boston, MA), UCSF Clinical Labs (San Francisco, CA), Labcorp Tennessee Knoxville (Knoxville, Tennessee), Vidant Medical Center (Greenville, NC), and Manchester Memorial Hospital (Manchester, CT).
This work was supported by Merck & Co.
Footnote
Conflicts of Interest: DP Nicolau has acted as a consultant or speaker’s bureau member for, or has received research funding from: Achaogen, Bayer, Cepheid, Melinta, Merck, Pfizer, Shionogi, and Tetraphase. The other authors have no conflicts of interest to declare.
References
- Planet PJ. Pseudomonas aeruginosa. In: Long S, Prober C, Fischer M. Principles and Practice of Pediatric Infectious Diseases. Canada: Elsevier, 2018:866-70.e1.
- Sievert DM, Ricks P, Edwards JR, et al. Antimicrobial-resistant pathogens associated with healthcare-associated infections: summary of data reported to the National Healthcare Safety Network at the Centers for Disease Control and Prevention, 2009-2010. Infect Control Hosp Epidemiol 2013;34:1-14. [Crossref] [PubMed]
- ZERBAXA (ceftolozane/tazobactam) for injection, for intravenous use. Initial U.S. approval 2014. Lexington, Mass: Cubist Pharmaceuticals, 2014.
- Pfaller MA, Bassetti M, Duncan LR, et al. Ceftolozane/tazobactam activity against drug-resistant Enterobacteriaceae and Pseudomonas aeruginosa causing urinary tract and intraabdominal infections in Europe: report from an antimicrobial surveillance programme (2012-15). J Antimicrob Chemother 2017;72:1386-95. [Crossref] [PubMed]
- Boyd N, Nailor MD. Combination antibiotic therapy for empiric and definitive treatment of gram-negative infections: insights from the Society of Infectious Diseases Pharmacists. Pharmacotherapy 2011;31:1073-84. [Crossref] [PubMed]
- Clinical and Laboratory Standards Institute (CLSI). Performance Standards for Antimicrobial Susceptibility Testing, 26th ed. 2016. Clinical and Laboratory Standards Institute, Wayne, PA.
- Sutherland CA, Nicolau DP. Susceptibility Profile of Ceftolozane/Tazobactam and Other Parenteral Antimicrobials Against Escherichia coli, Klebsiella pneumoniae, and Pseudomonas aeruginosa From US Hospitals. Clin Ther 2015;37:1564-71. [Crossref] [PubMed]
- Shortridge D, Castanheira M, Pfaller MA, et al. Ceftolozane-Tazobactam Activity against Pseudomonas aeruginosa Clinical Isolates from U.S. Hospitals: Report from the PACTS Antimicrobial Surveillance Program, 2012 to 2015. Antimicrob Agents Chemother 2017. [Crossref] [PubMed]
- Sader HS, Flamm RK, Carvalhaes CG, et al. Antimicrobial Susceptibility of Pseudomonas aeruginosa to Ceftazidime-Avibactam, Ceftolozane-Tazobactam, Piperacillin-Tazobactam, and Meropenem Stratified by U.S. Census Divisions: Results from the 2017 INFORM Program. Antimicrob Agents Chemother 2018. [Crossref] [PubMed]
- Denisuik AJ, Garbutt LA, Golden AR, et al. Antimicrobial-resistant pathogens in Canadian ICUs: results of the CANWARD 2007 to 2016 study. J Antimicrob Chemother 2019;74:645-53. [Crossref] [PubMed]
- Eagye KJ, Banevicius MA, Nicolau DP. Pseudomonas aeruginosa is not just in the intensive care unit any more: implications for empirical therapy. Crit Care Med 2012;40:1329-32. [Crossref] [PubMed]
- Humphries RM, Hindler JA, Wong-Beringer A, et al. Activity of Ceftolozane-Tazobactam and Ceftazidime-Avibactam against Beta-Lactam-Resistant Pseudomonas aeruginosa Isolates. Antimicrob Agents Chemother 2017. [Crossref] [PubMed]
- Goodlet KJ, Nicolau DP, Nailor MD. In Vitro Comparison of Ceftolozane-Tazobactam to Traditional Beta-Lactams and Ceftolozane-Tazobactam as an Alternative to Combination Antimicrobial Therapy for Pseudomonas aeruginosa. Antimicrob Agents Chemother 2017. doi: . [Crossref]