Targeting pro-resolution pathways to combat chronic inflammation in COPD
Introduction
Chronic obstructive pulmonary disease (COPD) is a major global health issue and is a leading cause of morbidity and mortality worldwide (1). Cigarette smoke exposure is a primary cause of COPD and as smoking rates continue to rise in South-East Asia, the long disease latency of COPD will impact dramatically on global health care resources for many decades to come. It is also well established that environmental influences such as indoor and outdoor air pollutants contribute to the development of chronic lung disease in individuals with no smoking history (2). Pathologically, COPD manifests into impaired lung function and irreversible airflow obstruction as a consequence of small airways disease, excessive mucus production and the development of emphysema. The mechanisms that drive such deleterious remodelling processes are heterogeneous in nature; however it is evident that chronic inflammation and excessive oxidative stress become central to the progression of COPD (3).
Nature of persistent inflammation in COPD
Innate and adaptive immune cells accumulate with progression of COPD. CD8+ T cells increase with disease severity and can release proteolytic enzymes that contribute to apoptosis of structural cells (4). CD4+ T cells, B cells and dendritic cells also aggregate into organised tertiary lymphoid organs (TLOs), which are found in COPD lungs and experimental models of chronic cigarette smoke exposure (5). The significance of these lymphoid follicles has yet to be established, where B cell accumulation may prove to be beneficial in terms of antibody production to colonising and invading pathogens, or detrimental through autoantibody production against lung tissue antigens (6). Of note, targeted disruption of TLO formation through neutralisation of CXCL13 does not reduce lung inflammation and alveolar enlargement in a chronic smoke exposure model (7), suggesting that immune cells of the innate system are sufficient to drive pathological changes that occur in COPD. In addition, inflammation does not fully resolve even when individuals with COPD stop smoking. Inflammation persists in bronchial biopsies and in sputum samples from COPD patients when compared to asymptomatic smokers (8-10). In particular, neutrophilic inflammation remained elevated in COPD subjects who had ceased smoking for at least one year (8-10). Given that neutrophils are a relatively short-lived immune cell; their persistence in COPD indicates that there is continual recruitment of leukocytes into the airways even when the primary insult of smoke exposure is removed.
Smoke exposure is particularly deleterious to host immunity against respiratory pathogens. Macrophage function is compromised in a microenvironment where there is excessive oxidative stress, leading to a deficiency in phagocytosis of bacteria (11) and efferocytosis of apoptotic cells (12). Free radicals have been shown to impair clearance mechanisms by directly causing cytoskeletal instability and carbonyl modification of pseudopodia (13-15). Macrophages also interact with carbonyl-adduct modified extracellular matrix proteins, which impair their ability to clear apoptotic neutrophils (16). Reduced macrophage function is consistent with high colonisation rates in COPD, where up to 50% of COPD patients are chronically colonized with microorganisms including Haemophilus influenzae, Streptococcus pneumoniae and Moraxella catarrhalis (17,18). Impaired immunity to respiratory pathogens also contributes to susceptibility to acute exacerbations of COPD (AECOPD), which are predominately caused by a new respiratory pathogen that is different to the colonized pathogen (19). AECOPDs result in a more rapid decline in lung function (20), which adversely affects mortality rates (21) and health related quality of life (22). Viral infections are a common cause of AECOPDs (23,24), as are bacterial infections, which are associated with a marked increase in neutrophilic inflammation (25).
Bacterial colonization and AECOPDs contribute to persistent inflammation of the airways, as neutrophils and monocytes are recruited from the circulation in an attempt to clear the invading pathogens. Since resident airway macrophages in COPD have a reduced capacity to clear efferocytic neutrophils, the emergence of necrotic neutrophils may further facilitate a vicious cycle of inflammation through release of endogenous damage associated molecular patterns (DAMPs). For example, degranulating necrotic bodies release neutrophil elastase, which localize to lung elastic fibers in emphysematic patients and degrades extracellular matrix components (26). Excessive neutrophil elastase activity can also promote the release of mucins through Epidermal Growth Factor Receptor (EGFR)-dependent mechanisms (27). Furthermore, increased EGFR transactivation augments inflammatory responses initiated by rhinovirus infection in bronchial epithelial cells (28). Necrotic neutrophils also release High-Mobility Group Box-1 (HMGB1), which is a DNA binding protein that is elevated in COPD airways (29). HMGB1 can synergize with microbial products and endogenous cytokines to enhance inflammation through Toll Like Receptor (TLR) (30,31) and Receptor for Advanced Glycosylation End (RAGE) (29) dependent mechanisms. Hence, COPD airways represent a highly complex milieu consisting of inhaled irritants, respiratory pathogens and endogenous mediators released from damaged tissue, which collectively drive excessive host immunity and sterile inflammation.
Pro-resolution mediators promote catabasis in the inflamed airway
The ongoing recruitment of leukocytes from the circulation is consistent with increased local production of CXCL chemokines in COPD including IL-8 (CXCL8), ENA78 (CXCL5), GCP-2 (CXCL6) and GRO isoforms (CXCL1-3) (32,33). The CXCR family of G coupled protein receptors (GPCR) binds to these endogenous chemokines, where CXCR2 is the cognate receptor for this family (34). There is also a high degree of redundancy in leukocyte mobilization as alternate mediators such as leukotriene B4 (LTB4) are associated with neutrophilic inflammation in colonized COPD patients (35). These pathways are essential to lung host immunity and are normally self-limiting through the activation of pro-resolution pathways. Pro-resolving mediators actively counterbalance inflammation by effectively switching off mechanisms that maintain leukocyte recruitment and survival. Eicosanoids such as lipoxins, resolvins and protectins and the protein termed Annexin A1 are integral to resolution of inflammation, [reviewed in (36-38)]; where there is a class switch of eicosanoid production from chemoattractants (such as the leukotriene LTB4) to pro-resolving mediators (such as lipoxin A4, LXA4) (39).
Lipoxins are synthesized in response to cell-cell interactions [reviewed in (40,41)]. In the lung, 15-lipoxygenase is expressed in epithelial cells and converts arachidonic acid to 15S-hydroxyleicosatetraenoic acid (15S-HETE). This metabolite is then taken up by neutrophils and converted to LXA4 by the enzyme 5-lipoxygenase (42). LXA4 opposes leukocyte migration and activation through multiple mechanisms including suppression of transendothelial (43) and transepithelial (44) migration and azurophilic degranulation (45). LXA4 can also directly target activated mucosal epithelial cells by opposing production of inflammatory cytokines (46). In vivo, administration of a stable analog of LXA4 reduced pulmonary inflammation and airway hyperreactivity in a murine model of asthma (47). Furthermore, this eicosanoid can directly contribute to tissue repair through the promotion of basal cell proliferation required for wound healing following mucosal injury (46). Another important anti-inflammatory role for LXA4 is its influence on macrophage function. Treatment with nanomolar concentrations of LXA4 stimulated more efficient efferocytosis of apoptotic neutrophils in human monocyte derived macrophages without provoking release of inflammatory mediators (48,49). LXA4-induced uptake of apoptotic neutrophils was reduced in macrophages from Fpr2 deficient mice, which is the mouse ortholog of the human ALX/FPR2 G-protein coupled receptor (GPCR) that potently interacts with lipoxins (50).
ALX/FPR2 is central to resolution of inflammation
In addition to lipoxins, the alternate anti-inflammatory mediators Annexin A1 and Resolvin D1 also interact with ALX/FPR2, implicating this receptor as an integral component of pro-resolution pathways. Accordingly, they display overlapping functions, where both LXA4 (51) and Annexin A1 (52) promote apoptosis of neutrophils as a mechanism of resolving acute inflammatory responses. Unlike lipid mediators derived from cell-cell interactions, Annexin A1 is highly abundant in neutrophils where the 37kDa protein is localized to the cytoplasm and in gelatinase/azurophilic granules. Annexin A1 is a glucocorticoid-induced lipocortin that can be rapidly mobilized through degranulation of neutrophils. Cytoplasmic Annexin A1 is also subjected to post translational modifications such as phosphorylation, which facilitate translocation to the cellular membrane [reviewed in (52)]. In addition to promoting neutrophil apoptosis, Annexin A1 reduces neutrophil endothelial attachment and subsequent transmigration. Like LXA4, Annexin A1 has also been shown to enhance efferocytosis of apoptotic neutrophils by macrophages [reviewed in (52)], thereby facilitating catabasis of the inflamed tissue to homeostasis.
Another family of mediators that target ALX/FPR2 is the D series resolvins derived from the omega-3 fatty acid, docosahexaenoic acid (DHA) [reviewed in (53)]. Although there is relatively less information on the actions of D-series resolvins in lung diseases, there is emerging evidence to support an important role in resolution of lung inflammation. In a murine model of acute lung injury, treatment with stable derivatives of Resolvin D1 improved epithelial and endothelial integrity and reduced neutrophilic inflammation concurrently with reduced inflammatory cytokine secretion in the airways (54). In addition, aspirin-triggered Resolvin D1 (AT-RvD1) significantly reduced airway inflammation and enhanced macrophage phagocytosis in a murine asthma model (55). Furthermore, Resolvin D1 reduced neutrophilic lung inflammation, inflammatory cytokine production and phagocytosis in an acute cigarette smoke exposure model (56).
Is there an imbalance between inflammatory and pro-resolving mediators in COPD?
Given their important role in resolution of acute inflammation, any perturbation in the production of pro-resolving mediators can lead to the generation of pathogenic inflammatory conditions. Indeed, it has been shown that reduced production of LXA4 relative to cysteinyl leukotrienes is associated with the persistence of inflammation in severe asthma (57,58). Reduced levels of LXA4 are also observed in exhaled breath condensate during asthma exacerbations (59) and in the airway fluid of patients with cystic fibrosis (60). Since multiple mediators converge on ALX/FPR2, their relative abundance within the airway milieu of the chronically inflamed lung can profoundly influence catabasis and restoration of homeostasis.
ALX/FPR2 belongs to the N-formyl peptide receptor (FPR) family, of which there are three human members (FPR1, ALX/FPR2 and FPR3). FPRs demonstrate wide tissue distribution, although ALX/FPR2 expression is particularly prominent on myeloid cells including neutrophils and monocytes (61). Airway mucosal epithelial cells also express ALX/FPR2 in a manner that is increased via COX-2 dependent mechanisms in response to injury (46). Prominent staining is also observed on the apical and basolateral side of the epithelium of COPD airways (62).
ALX/FPR2 is a GPCR superfamily member characterized by seven putative TM domains that displays diverse ligand affinities that extend beyond interactions with lipoxins, series D-resolvins and Annexin A1. Accordingly, ALX/FPR2 can interact with over 30 ligands that can exert opposing biological actions (63). Hence, selective agonists are likely to bind with different affinities and given the diverse conformation of endogenous and synthetic ligands, are likely to bind to alternate regions of the receptor. Numerous receptor conformations can exist where ligand binding alters receptor formation, which facilitates alternate functional behaviors that control multiple downstream signaling pathways (64). This is true for ALX/FPR2, where agonist biased signaling can either promote inflammation or resolution contingent on alternate receptor conformations [reviewed in (65)]. Serum Amyloid A (SAA) and the anti-microbial peptide LL-37 both interact with ALX/FPR2 and in complete contrast to pro-resolving mediators, promote neutrophil transmigration, activation and survival (summarized in Figure 1). Another high affinity ligand for ALX/FPR2 includes mitochondrial N-formylated hexapeptides derived from NADH dehydrogenase and cytochrome c oxidase subunits (66). These formylated peptides are released from damaged cells and can interact with FPR1 and ALX/FPR2 (66). Although it is not known whether these ligands are elevated in COPD, there is increased mitochondrial dysfunction and cytochrome c oxidase levels in the skeletal muscle of COPD patients (67-69).
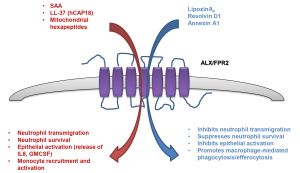
There are multiple mechanisms that may address why ALX/FPR2 receptors modulate cell responsiveness in a ligand-biased fashion. This includes the formation of homologous and/or heterologous receptor dimers and differential conformational ligand activation of specific receptor domains. The molecular nature of how ALX/FPR2 downstream signaling pathways are activated in a ligand-specific manner has yet to be fully characterized. However, there is evidence for conformational ligand activation. LXA4 has been shown to activate ALX/FPR2 by interacting with extracellular loop III and the associated transmembrane region (70), whereas SAA initiates extracellular loops I and II dependent signaling (71). In addition, SAA has recently been shown to promote ALX/FPR2 homodimerisation and activation of pro-survival pathways, which can be counteracted by Annexin A1 that promotes an alternative receptor conformation that engages pro-apoptotic pathways (72).
Conclusions
Since the accurate detection of lipid pro-resolution mediators in human tissue requires LC-LC-MS approaches that have only recently become available, there are currently relatively few studies demonstrating their presence in human tissue (73). Hence, there is a current knowledge gap in terms of whether there is a deficiency in production of pro-resolving mediators in COPD that facilitates a chronic inflammatory state, and advances in detection technology will reveal new insight here. Importantly, this should inform on the development of new classes of drugs to treat COPD away from conventional steroid-like anti-inflammatory approaches that can detrimentally dampen immunity leading to immunosuppression. Specialized pro-resolving mediators offer an alternative approach to switching off chronic inflammation and may concurrently boost beneficial host immunity and tissue repair mechanisms. Whilst the organic synthesis of pro-resolving lipid mediators can be challenging, chemically stable E-class resolvin analogs have been successfully synthesized and are currently in Phase III clinical trial for chronic inflammatory dry eye syndrome (Resolvyx Pharmaceuticals in partnership with Celtic Therapeutics).
Of interest, two human phenotypes have been described in response to cantharidin-induced skin blisters in male healthy volunteers involving distinct production of LXA4, which controlled the longevity and severity of the inflammation (74). The relative abundance of alternative ALX/FPR2 ligands may also directly influence the functional behavior of this complex receptor in COPD airways, where there is a rich milieu of microbial derived products and endogenous mediators. LL-37 (or hCAP-18) is a breakdown product of cathelicidin that promotes microbial killing through pore formation. LL-37 is also a chemoattractant for neutrophils, monocytes and lymphocytes via its actions on ALX/FPR2 (75). Since LL-37 levels are elevated in sputum samples from stable COPD patients (76) and are further increased during bacterial AECOPDs (77), this normally protective molecule may contribute to persistent inflammation. Likewise, SAA, which has previously been characterized as a systemic biomarker for AECOPD severity (78), is prominently expressed in lung resection tissue from COPD patients (62). Using the same lung resection tissue, SAA transcript expression was also detected and found to be positively associated with the number of tissue neutrophils (79).
In addition to promoting neutrophil survival via ALX/FPR2, SAA is a potent chemotactic factor that mediates migration of leukocytes (80) and can also stimulate expression of pro- inflammatory mediators under in vitro (81) and in vivo conditions (62). More recently, SAA was shown to promote the differentiation of monocyte derived macrophages into a pro-inflammatory phenotype that expresses higher levels of the TH17 polarizing cytokines, IL-6 and IL-1β in a manner that was dependent on CSF-1R signaling (82). Hence, the relative abundance and persistence of pro-inflammatory agonists such as SAA and LL-37 in COPD airways is likely to facilitate agonist biased signaling that favors leukocyte recruitment, activation and survival. The nature of this receptor also provides therapeutic opportunities to address ALX/FPR2 conformations that may lead to pathogenic functions through the development of more stable analogs of lipoxins, D-series resolvins and cleavage resistant Annexin A1 mimetics, which can override pro-inflammatory signals to initiate resolution of inflammation.
Acknowledgements
This work was supported by the National Health & Medical Research Council (NHMRC) of Australia and an Australian Research Council (ARC) Future Fellowship to Steven Bozinovski. The authors declare no conflict of interest.
Disclosure: The authors declare no conflict of interest.
References
- Jemal A, Ward E, Hao Y, et al. Trends in the leading causes of death in the United States, 1970-2002. Jama 2005;294:1255-9. [PubMed]
- Lopez AD, Mathers CD, Ezzati M, et al. Measuring the Global Burden of Disease and Risk Factors, 1990-2001. Washington DC: World Bank, 2006.
- Barnes PJ. Immunology of asthma and chronic obstructive pulmonary disease. Nat Rev Immunol 2008;8:183-92. [PubMed]
- Cosio MG, Saetta M, Agusti A. Immunologic aspects of chronic obstructive pulmonary disease. N Engl J Med 2009;360:2445-54. [PubMed]
- Brusselle GG, Joos GF, Bracke KR. New insights into the immunology of chronic obstructive pulmonary disease. Lancet 2011;378:1015-26. [PubMed]
- Hansbro PM, Knight DA. Are lymphoid follicles important in the pathogenesis of chronic obstructive pulmonary disease? Am J Respir Crit Care Med 2013;188:267-9. [PubMed]
- Bracke KR, Verhamme FM, Seys LJ, et al. Role of CXCL13 in cigarette smoke-induced lymphoid follicle formation and chronic obstructive pulmonary disease. Am J Respir Crit Care Med 2013;188:343-55. [PubMed]
- Rutgers SR, Postma DS, ten Hacken NH, et al. Ongoing airway inflammation in patients with COPD who Do not currently smoke. Chest 2000;117:262S. [PubMed]
- Stănescu D, Sanna A, Veriter C, et al. Airways obstruction, chronic expectoration, and rapid decline of FEV1 in smokers are associated with increased levels of sputum neutrophils. Thorax 1996;51:267-71. [PubMed]
- Willemse BW, ten Hacken NH, Rutgers B, et al. Effect of 1-year smoking cessation on airway inflammation in COPD and asymptomatic smokers. Eur Respir J 2005;26:835-45. [PubMed]
- Martí-Lliteras P, Regueiro V, Morey P, et al. Nontypeable Haemophilus influenzae clearance by alveolar macrophages is impaired by exposure to cigarette smoke. Infect Immun 2009;77:4232-42. [PubMed]
- Hodge S, Hodge G, Ahern J, et al. Smoking alters alveolar macrophage recognition and phagocytic ability: implications in chronic obstructive pulmonary disease. Am J Respir Cell Mol Biol 2007;37:748-55. [PubMed]
- Banan A, Zhang Y, Losurdo J, et al. Carbonylation and disassembly of the F-actin cytoskeleton in oxidant induced barrier dysfunction and its prevention by epidermal growth factor and transforming growth factor alpha in a human colonic cell line. Gut 2000;46:830-7. [PubMed]
- Smerjac SM, Bizzozero OA. Cytoskeletal protein carbonylation and degradation in experimental autoimmune encephalomyelitis. J Neurochem 2008;105:763-72. [PubMed]
- Bozinovski S, Vlahos R, Zhang Y, et al. Carbonylation caused by cigarette smoke extract is associated with defective macrophage immunity. Am J Respir Cell Mol Biol 2011;45:229-36. [PubMed]
- Kirkham PA, Spooner G, Rahman I, et al. Macrophage phagocytosis of apoptotic neutrophils is compromised by matrix proteins modified by cigarette smoke and lipid peroxidation products. Biochem Biophys Res Commun 2004;318:32-7. [PubMed]
- Monsó E, Ruiz J, Rosell A, et al. Bacterial infection in chronic obstructive pulmonary disease. A study of stable and exacerbated outpatients using the protected specimen brush. Am J Respir Crit Care Med 1995;152:1316-20. [PubMed]
- Pela R, Marchesani F, Agostinelli C, et al. Airways microbial flora in COPD patients in stable clinical conditions and during exacerbations: a bronchoscopic investigation. Monaldi Arch Chest Dis 1998;53:262-7. [PubMed]
- Papi A, Bellettato CM, Braccioni F, et al. Infections and airway inflammation in chronic obstructive pulmonary disease severe exacerbations. Am J Respir Crit Care Med 2006;173:1114-21. [PubMed]
- Donaldson GC, Seemungal TA, Bhowmik A, et al. Relationship between exacerbation frequency and lung function decline in chronic obstructive pulmonary disease. Thorax 2002;57:847-52. [PubMed]
- Soler-Cataluña JJ, Martinez-Garcia MA, Roman Sanchez P, et al. Severe acute exacerbations and mortality in patients with chronic obstructive pulmonary disease. Thorax 2005;60:925-31. [PubMed]
- Donaldson GC, Wilkinson TM, Hurst JR, et al. Exacerbations and time spent outdoors in chronic obstructive pulmonary disease. Am J Respir Crit Care Med 2005;171:446-52. [PubMed]
- Hutchinson AF, Ghimire AK, Thompson MA, et al. A community-based, time-matched, case-control study of respiratory viruses and exacerbations of COPD. Respir Med 2007;101:2472-81. [PubMed]
- Mallia P, Message SD, Gielen V, et al. Experimental rhinovirus infection as a human model of chronic obstructive pulmonary disease exacerbation. Am J Respir Crit Care Med 2011;183:734-42. [PubMed]
- Gompertz S, O'Brien C, Bayley DL, et al. Changes in bronchial inflammation during acute exacerbations of chronic bronchitis. Eur Respir J 2001;17:1112-9. [PubMed]
- Damiano VV, Tsang A, Kucich U, et al. Immunolocalization of elastase in human emphysematous lungs. J Clin Invest 1986;78:482-93. [PubMed]
- Shao MX, Nadel JA. Neutrophil elastase induces MUC5AC mucin production in human airway epithelial cells via a cascade involving protein kinase C, reactive oxygen species, and TNF-alpha-converting enzyme. J Immunol 2005;175:4009-16. [PubMed]
- Liu K, Gualano RC, Hibbs ML, et al. Epidermal growth factor receptor signaling to Erk1/2 and STATs control the intensity of the epithelial inflammatory responses to rhinovirus infection. J Biol Chem 2008;283:9977-85. [PubMed]
- Ferhani N, Letuve S, Kozhich A, et al. Expression of high-mobility group box 1 and of receptor for advanced glycation end products in chronic obstructive pulmonary disease. Am J Respir Crit Care Med 2010;181:917-27. [PubMed]
- Sha Y, Zmijewski J, Xu Z, et al. HMGB1 develops enhanced proinflammatory activity by binding to cytokines. J Immunol 2008;180:2531-7. [PubMed]
- Youn JH, Oh YJ, Kim ES, et al. High mobility group box 1 protein binding to lipopolysaccharide facilitates transfer of lipopolysaccharide to CD14 and enhances lipopolysaccharide-mediated TNF-alpha production in human monocytes. J Immunol 2008;180:5067-74. [PubMed]
- Qiu Y, Zhu J, Bandi V, et al. Biopsy neutrophilia, neutrophil chemokine and receptor gene expression in severe exacerbations of chronic obstructive pulmonary disease. Am J Respir Crit Care Med 2003;168:968-75. [PubMed]
- Stevenson CS, Coote K, Webster R, et al. Characterization of cigarette smoke-induced inflammatory and mucus hypersecretory changes in rat lung and the role of CXCR2 ligands in mediating this effect. Am J Physiol Lung Cell Mol Physiol 2005;288:L514-22. [PubMed]
- Wolf M, Delgado MB, Jones SA, et al. Granulocyte chemotactic protein 2 acts via both IL-8 receptors, CXCR1 and CXCR2. Eur J Immunol 1998;28:164-70. [PubMed]
- Banerjee D, Khair OA, Honeybourne D. Impact of sputum bacteria on airway inflammation and health status in clinical stable COPD. Eur Respir J 2004;23:685-91. [PubMed]
- Dufton N, Perretti M. Therapeutic anti-inflammatory potential of formyl-peptide receptor agonists. Pharmacol Ther 2010;127:175-88. [PubMed]
- Levy BD, Vachier I, Serhan CN. Resolution of inflammation in asthma. Clin Chest Med 2012;33:559-70. [PubMed]
- Serhan CN, Chiang N, Van Dyke TE. Resolving inflammation: dual anti-inflammatory and pro-resolution lipid mediators. Nat Rev Immunol 2008;8:349-61. [PubMed]
- Levy BD, Clish CB, Schmidt B, et al. Lipid mediator class switching during acute inflammation: signals in resolution. Nat Immunol 2001;2:612-9. [PubMed]
- Chiang N, Serhan CN, Dahlen SE, et al. The lipoxin receptor ALX: potent ligand-specific and stereoselective actions in vivo. Pharmacol Rev 2006;58:463-87. [PubMed]
- Serhan CN. Lipoxins and aspirin-triggered 15-epi-lipoxins are the first lipid mediators of endogenous anti-inflammation and resolution. Prostaglandins Leukot Essent Fatty Acids 2005;73:141-62. [PubMed]
- Serhan CN. Lipoxins and novel aspirin-triggered 15-epi-lipoxins (ATL): a jungle of cell-cell interactions or a therapeutic opportunity? Prostaglandins 1997;53:107-37. [PubMed]
- Papayianni A, Serhan CN, Brady HR. Lipoxin A4 and B4 inhibit leukotriene-stimulated interactions of human neutrophils and endothelial cells. J Immunol 1996;156:2264-72. [PubMed]
- Colgan SP, Serhan CN, Parkos CA, et al. Lipoxin A4 modulates transmigration of human neutrophils across intestinal epithelial monolayers. J Clin Invest 1993;92:75-82. [PubMed]
- Soyombo O, Spur BW, Lee TH. Effects of lipoxin A4 on chemotaxis and degranulation of human eosinophils stimulated by platelet-activating factor and N-formyl-L-methionyl-L-leucyl-L-phenylalanine. Allergy 1994;49:230-4. [PubMed]
- Bonnans C, Fukunaga K, Levy MA, et al. Lipoxin A(4) regulates bronchial epithelial cell responses to acid injury. Am J Pathol 2006;168:1064-72. [PubMed]
- Levy BD, De Sanctis GT, Devchand PR, et al. Multi-pronged inhibition of airway hyper-responsiveness and inflammation by lipoxin A(4). Nat Med 2002;8:1018-23. [PubMed]
- El Kebir D, Jozsef L, Pan W, et al. 15-epi-lipoxin A4 inhibits myeloperoxidase signaling and enhances resolution of acute lung injury. Am J Respir Crit Care Med 2009;180:311-9. [PubMed]
- Godson C, Mitchell S, Harvey K, et al. Cutting edge: lipoxins rapidly stimulate nonphlogistic phagocytosis of apoptotic neutrophils by monocyte-derived macrophages. J Immunol 2000;164:1663-7. [PubMed]
- Maderna P, Cottell DC, Toivonen T, et al. FPR2/ALX receptor expression and internalization are critical for lipoxin A4 and annexin-derived peptide-stimulated phagocytosis. FASEB J 2010;24:4240-9. [PubMed]
- El Kebir D, Jozsef L, Khreiss T, et al. Aspirin-triggered lipoxins override the apoptosis-delaying action of serum amyloid A in human neutrophils: a novel mechanism for resolution of inflammation. J Immunol 2007;179:616-22. [PubMed]
- Perretti M, D’Acquisto F. Annexin A1 and glucocorticoids as effectors of the resolution of inflammation. Nat Rev Immunol 2009;9:62-70. [PubMed]
- Levy BD. Resolvin D1 and Resolvin E1 Promote the Resolution of Allergic Airway Inflammation via Shared and Distinct Molecular Counter-Regulatory Pathways. Front Immunol 2012;3:390. [PubMed]
- Eickmeier O, Seki H, Haworth O, et al. Aspirin-triggered resolvin D1 reduces mucosal inflammation and promotes resolution in a murine model of acute lung injury. Mucosal Immunol 2013;6:256-66. [PubMed]
- Rogerio AP, Haworth O, Croze R, et al. Resolvin D1 and aspirin-triggered resolvin D1 promote resolution of allergic airways responses. J Immunol 2012;189:1983-91. [PubMed]
- Hsiao HM, Sapinoro RE, Thatcher TH, et al. A novel anti-inflammatory and pro-resolving role for resolvin D1 in acute cigarette smoke-induced lung inflammation. PLoS One 2013;8:e58258. [PubMed]
- Planagumà A, Kazani S, Marigowda G, et al. Airway lipoxin A4 generation and lipoxin A4 receptor expression are decreased in severe asthma. Am J Respir Crit Care Med 2008;178:574-82. [PubMed]
- Levy BD, Bonnans C, Silverman ES, et al. Diminished lipoxin biosynthesis in severe asthma. Am J Respir Crit Care Med 2005;172:824-30. [PubMed]
- Hasan RA, O'Brien E, Mancuso P. Lipoxin A(4) and 8-isoprostane in the exhaled breath condensate of children hospitalized for status asthmaticus. Pediatr Crit Care Med 2012;13:141-5. [PubMed]
- Karp CL, Flick LM, Park KW, et al. Defective lipoxin-mediated anti-inflammatory activity in the cystic fibrosis airway. Nat Immunol 2004;5:388-92. [PubMed]
- Murphy PM, Ozcelik T, Kenney RT, et al. A structural homologue of the N-formyl peptide receptor. Characterization and chromosome mapping of a peptide chemoattractant receptor family. J Biol Chem 1992;267:7637-43. [PubMed]
- Bozinovski S, Uddin M, Vlahos R, et al. Serum amyloid A opposes lipoxin A(4) to mediate glucocorticoid refractory lung inflammation in chronic obstructive pulmonary disease. Proc Natl Acad Sci U S A 2012;109:935-40. [PubMed]
- Ye RD, Boulay F, Wang JM, et al. International Union of Basic and Clinical Pharmacology. LXXIII. Nomenclature for the formyl peptide receptor (FPR) family. Pharmacol Rev 2009;61:119-61. [PubMed]
- Kenakin T. Functional selectivity and biased receptor signaling. J Pharmacol Exp Ther 2011;336:296-302. [PubMed]
- Filep JG. Biasing the lipoxin A4/formyl peptide receptor 2 pushes inflammatory resolution. Proc Natl Acad Sci U S A 2013;110:18033-4. [PubMed]
- Rabiet MJ, Huet E, Boulay F. Human mitochondria-derived N-formylated peptides are novel agonists equally active on FPR and FPRL1, while Listeria monocytogenes-derived peptides preferentially activate FPR. Eur J Immunol 2005;35:2486-95. [PubMed]
- Puente-Maestu L, Perez-Parra J, Godoy R, et al. Abnormal mitochondrial function in locomotor and respiratory muscles of COPD patients. Eur Respir J 2009;33:1045-52. [PubMed]
- Puente-Maestu L, Perez-Parra J, Godoy R, et al. Abnormal transition pore kinetics and cytochrome C release in muscle mitochondria of patients with chronic obstructive pulmonary disease. Am J Respir Cell Mol Biol 2009;40:746-50. [PubMed]
- Rabinovich RA, Bastos R, Ardite E, et al. Mitochondrial dysfunction in COPD patients with low body mass index. Eur Respir J 2007;29:643-50. [PubMed]
- Chiang N, Fierro IM, Gronert K, et al. Activation of lipoxin A(4) receptors by aspirin-triggered lipoxins and select peptides evokes ligand-specific responses in inflammation. J Exp Med 2000;191:1197-208. [PubMed]
- Bena S, Brancaleone V, Wang JM, et al. Annexin A1 interaction with the FPR2/ALX receptor: identification of distinct domains and downstream associated signaling. J Biol Chem 2012;287:24690-7. [PubMed]
- Cooray SN, Gobbetti T, Montero-Melendez T, et al. Ligand-specific conformational change of the G-protein-coupled receptor ALX/FPR2 determines proresolving functional responses. Proc Natl Acad Sci U S A 2013;110:18232-7. [PubMed]
- Serhan CN. Pro-resolving lipid mediators are leads for resolution physiology. Nature 2014;510:92-101. [PubMed]
- Morris T, Stables M, Colville-Nash P, et al. Dichotomy in duration and severity of acute inflammatory responses in humans arising from differentially expressed proresolution pathways. Proc Natl Acad Sci U S A 2010;107:8842-7. [PubMed]
- De Yang, Chen Q, Schmidt AP, et al. LL-37, the neutrophil granule- and epithelial cell-derived cathelicidin, utilizes formyl peptide receptor-like 1 (FPRL1) as a receptor to chemoattract human peripheral blood neutrophils, monocytes, and T cells. J Exp Med 2000;192:1069-74. [PubMed]
- Xiao W, Hsu YP, Ishizaka A, et al. Sputum cathelicidin, urokinase plasminogen activation system components, and cytokines discriminate cystic fibrosis, COPD, and asthma inflammation. Chest 2005;128:2316-26. [PubMed]
- Parameswaran GI, Sethi S, Murphy TF. Effects of bacterial infection on airway antimicrobial peptides and proteins in COPD. Chest 2011;140:611-7. [PubMed]
- Bozinovski S, Hutchinson A, Thompson M, et al. Serum amyloid A is a biomarker of acute exacerbations of chronic obstructive pulmonary disease. Am J Respir Crit Care Med 2008;177:269-78. [PubMed]
- Anthony D, Seow HJ, Uddin M, et al. Serum amyloid A promotes lung neutrophilia by increasing IL-17A levels in the mucosa and gammadelta T cells. Am J Respir Crit Care Med 2013;188:179-86. [PubMed]
- Su SB, Gong W, Gao JL, et al. A seven-transmembrane, G protein-coupled receptor, FPRL1, mediates the chemotactic activity of serum amyloid A for human phagocytic cells. J Exp Med 1999;189:395-402. [PubMed]
- He R, Sang H, Ye RD. Serum amyloid A induces IL-8 secretion through a G protein-coupled receptor, FPRL1/LXA4R. Blood 2003;101:1572-81. [PubMed]
- Anthony D, McQualter JL, Bishara M, et al. SAA drives proinflammatory heterotypic macrophage differentiation in the lung via CSF-1R-dependent signaling. FASEB J 2014. [Epub ahead of print]. [PubMed]