Extracorporeal membrane oxygenation as rescue therapy for severe hypoxemic respiratory failure
History of extracorporeal membrane oxygenation (ECMO) for hypoxemic respiratory failure
Neonates
ECMO has been used for more than 50 years as salvage therapy for patients with severe cardiopulmonary failure that is refractory to conventional treatment. In the late 1930s, John Gibbon (1), after witnessing a young patient’s death from a pulmonary embolism, began experimenting with extracorporeal blood-flow circuits that might temporarily support cardiorespiratory function. He hypothesized that an effective circuit might allow surgical thrombectomy of massive life-threatening emboli and even potentially allow surgery on the heart. After two decades of painstaking experimentation, Gibbon performed the first successful operation using such an extracorporeal circuit, to close a large atrial septal defect in an 18-year-old woman (2). However, his device, and similar ones of that era, required direct contact between blood and gas, which damaged blood constituents and thus could be used for only a few hours at a time. An interesting observation published in 1944 helped to solve that problem and led to the next generation of extracorporeal oxygenators: Kolff and colleagues (3) observed that blood was oxygenated as it crossed the cellophane chambers in their first artificial kidney machine. By 1956, Clowes et al. (4) had developed a unique oxygenator with a membrane that separated the gaseous and liquid phases, allowing lengthier extracorporeal circulation of blood. This device was initially used as a “cardiopulmonary bypass” in the burgeoning field of open heart surgery (5).
By the 1960s, ECMO technology had been adapted for extracorporeal perfusion to support patients with cardiac and respiratory failure. In 1965, Rashkind and colleagues (6) made the first attempt to use extracorporeal circulation in a newborn with respiratory failure. Ten years later, Bartlett et al. (7) reported the first successful use of ECMO in a neonate with severe meconium aspiration syndrome. As a result of these efforts, the use of ECMO in newborns with refractory respiratory failure increased substantially, supported by results from randomized controlled trials confirming improved survival with ECMO in these infants (8-10). Since 1989, when the Extracorporeal Life Support Organization (ELSO) Registry was established and began collecting data on extracorporeal life support use and survival, more than 31,500 neonates have been treated with ECMO for respiratory failure; of these, 87% survived their ECMO course and 73% were subsequently discharged or transferred (11).
Adults
In 1972, Hill et al. (12) reported the first successful use of long-term ECMO in an adult with the newly described acute respiratory distress syndrome (ARDS). Two years later, the National Institutes of Health launched the first multicenter randomized clinical trial of ECMO versus conventional therapy in adults with ARDS (Table 1) (13). Of 90 trial participants, 42 received partial-flow venoarterial (VA)-ECMO. Two-week mortality was comparable in the treatment and control groups (90%); no survival advantage was associated with ECMO. In 1994, Morris et al. (14) published a second randomized trial that evaluated mechanical ventilation by using an inverse ratio of inspiratory and expiratory times with low-flow venovenous (VV)-ECMO versus conventional therapy. Of the 40 participants, 21 received VV ECMO. Survival 30 days after randomization did not differ significantly between the control and VV-ECMO groups (42% vs. 33%, respectively).
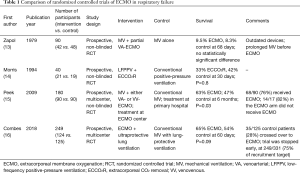
Full table
Given the discouraging results of these two trials, ECMO was not adopted into algorithms of evidence-based treatment for ARDS for the next 15 years. The results of those early trials, however, may not be relevant to modern ARDS management, because both ECMO deployment and technology, as well as conventional treatments for adult ARDS, have evolved considerably over time. Current ECMO support for hypoxemic respiratory failure is provided by VV (vena cava to right atrium) circuits, which are much less traumatic and better tolerated than the original VA circuits that arose from cardiopulmonary bypass practice. Newer oxygenators provide much more efficient gas exchange, and today’s circuits are much less likely to induce clotting, which has reduced the need for anticoagulation and blood products. These advances have enabled more effective, longer-term ECMO support with a lower incidence of complications, and by the late 1990s multiple centers had begun to report encouraging results from VV-ECMO use in respiratory failure caused by various reversible lung pathologies (17-19).
Today, ECMO is an accepted treatment for multiple causes of reversible respiratory failure, including primary graft dysfunction in lung transplant patients, and as a bridge to lung transplant for very select patients (20). Current indications, as well as absolute and relative contraindications for ECMO, are displayed in Figure 1.
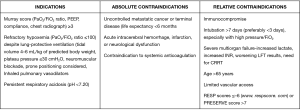
ARDS and ECMO
ARDS is currently understood as the final common pathway of many, if not most, lung injuries and is often the condition associated with severe hypoxemic respiratory failure in adults. There is no specific cure for ARDS, despite more than a half century of investigation. ARDS treatment is supportive and is based primarily on positive-pressure mechanical ventilation (21). ARDS is prevalent in intensive care units throughout the world, carries a high cost burden, and is associated with substantial mortality (22-24). In the 1980s and 1990s, even as investigators were exploring the utility of ECMO for patients in severe hypoxemic respiratory failure, an understanding of the toxic effects of positive-pressure mechanical ventilation was evolving. Mechanical ventilation was found to potentially injure lungs, primarily by overdistension (volutrauma), high airway pressure (barotrauma), and even underdistension (atelectotrauma) (25). Such trauma was found to induce local and systemic inflammation and to worsen already-impaired alveolar gas exchange. The sum of adverse effects from ventilators was termed “ventilator-induced lung injury” (VILI) (26,27). Unquestionably, the very foundation of supportive therapy for ARDS, the mechanical ventilator, was actually escalating lung injury and contributing to the high mortality from ARDS. Thus, in the 1990s there began an intense search for techniques and tools to mitigate the toxic effects of mechanical ventilation (28,29).
The landmark ARDSnet trial showed that a tidal volume of 6 mL/kg of predicted body weight and a plateau pressure of 30 cmH2O lowered in-hospital mortality and the number of ventilation days for patients with ARDS, compared with the traditional tidal volume of 12 mL/kg and plateau pressure of 50 cmH2O (30). However, further studies found morphological and physiological evidence of VILI despite such lung-protective tidal volumes (6 mL/kg) and plateau pressures (28–30 cmH2O), suggesting that more-robust lung-protective settings were warranted (31-33). Additionally, in many cases of severe ARDS, recommended ventilator settings do not allow adequate oxygenation or ventilation, forcing providers to use maximal oxygen concentrations and larger tidal volumes with higher airway pressures to adequately oxygenate and ventilate patients. Recent advances in prone positioning (34), positive end-expiratory pressure management, and lung recruitment maneuvers (35) were intended to improve oxygenation and decrease atelectotrauma and appear to have enhanced overall ARDS survival outcomes (36); nonetheless, much more improvement will be needed to mitigate VILI.
Such considerations prompted renewed interest in including ECMO in the treatment algorithm for ARDS. By supporting oxygenation and CO2 removal in severe ARDS with lung-protective mechanical ventilation, ECMO might achieve the ultimate protection from VILI.
ECMO makes a comeback in ARDS
Two different currents came together in the late 2000s to promote a global increase in the use of ECMO for respiratory failure: (I) the 2009 global swine-origin influenza A (H1N1) pandemic, during which many previously healthy young patients developed severe respiratory failure; and (II) the first major randomized controlled trial of VV-ECMO for respiratory failure [the CESAR (Conventional ventilation or ECMO for Severe Adult Respiratory failure) trial], in which ECMO was shown to have beneficial effects. This landmark trial set the foundation for the recently published EOLIA (Extracorporeal Membrane Oxygenation for Severe Acute Respiratory Distress Syndrome) trial.
ECMO for influenza management
ECMO use as a rescue treatment for severe ARDS and hypoxemic respiratory failure surged during the H1N1 pandemic. Reported results were highly encouraging. The Australia and New Zealand Extracorporeal Membrane Oxygenation Influenza Investigators reported a 75% survival rate among 68 patients who received ECMO for refractory H1N1-induced ARDS (37). Notably, these patients had severe ARDS at the time of ECMO cannulation: Median values were 56 mmHg for the ratio of partial pressure of arterial oxygen (PaO2) to fraction of inspired oxygen (FiO2), 18 cmH2O for the positive end-expiratory pressure requirement, and 3.8 for the Murray score. In a propensity-matched study from the United Kingdom, hospital mortality in patients with H1N1 transferred to an ECMO center was half that of matched non-ECMO-referred patients (38).
CESAR trial
In the UK-based multicenter CESAR trial (15), patients with severe ARDS were randomized 1:1 to receive ECMO or standard treatment (Table 1). A total of 180 patients (90 per arm) were enrolled from 68 centers over 5 years (July 2001–August 2006). ECMO patients were transferred to a single center, whereas conventional-care patients remained at their primary hospitals. Inclusion criteria were a Murray score ≥3, uncompensated hypercapnia, and age 18–65 years. Exclusion criteria were peak airway pressure >30 cmH2O or FiO2 >0.8 for ≥7 days, signs of intracranial bleeding, or any contraindication to systemic anticoagulation. Pneumonia was the underlying cause of ARDS for approximately two thirds of participants. The mean baseline PaO2/FiO2 ratio was 75 mmHg in the control group and 76 mmHg in the ECMO group.
The primary endpoint was death or severe disability within 6 months of randomization. Survival was significantly better in the ECMO group than in the conventional-treatment group (63% vs. 47%, respectively); the relative risk was 0.69 [95% confidence interval (CI), 0.05–0.97; P=0.03], and the number needed to treat was 7 patients. The absolute risk reduction for the primary outcome was 16%, and the number needed to treat was 6 patients (Figure 2). However, 24% of the patients in the intervention arm (22/90) never received ECMO: 5 died before or during transfer, and of the 17 who received conventional ventilation alone, 82% (14/17) survived without requiring ECMO. The trial design did not mandate a specific lung-protective ventilation strategy for the control group; as a result, only 70% of the control patients were managed with a lung-protective strategy, compared with 93% in the ECMO group. Prone positioning was employed comparably in both groups (42% in the conventional medical management group vs. 36% in the ECMO group; P=0.58).
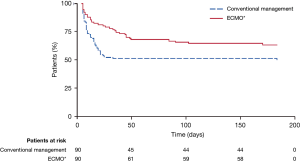
Despite its limitations, the CESAR trial showed the importance of transferring patients with potentially reversible respiratory failure to centers that specialize in ARDS management. The trial also supported lung-protective ventilation and VV-ECMO as effective treatments for severe ARDS, leading to expanded use of ECMO in clinical practice and further ECMO research. Nevertheless, because ECMO skeptics could question whether patients in the ECMO arm benefitted more from ECMO or from superior medical management, additional investigation to attempt to answer that question was warranted, and hence the EOLIA trial was undertaken.
EOLIA trial
The EOLIA trial was a multicenter, international, prospective, randomized trial for adults with severe ARDS that compared early VV-ECMO with standard lung-protective ventilation (16) (Table 1). The trial was designed to remedy the methodological limitations of the CESAR trial with strict mechanical ventilation control, ECMO initiation before transportation, and better adherence to the mechanical ventilation protocol in the control group. Inclusion criteria were severe hypoxemia with a PaO2/FiO2 ratio of <50 mmHg for >3 hours or <80 mmHg for >6 hours, or pH <7.25 and a partial pressure of arterial CO2 of ≥60 mmHg for >6 hours. Crossover to ECMO was allowed for control-group patients with refractory hypoxemia (defined as oxygen saturation of <80% for at least 6 hours) and no irreversible multiorgan failure. The primary endpoint was 60-day mortality.
At randomization, this high-risk cohort had a mean PaO2/FiO2 ratio of 73 mmHg and a mean sequential organ failure assessment score of 10.7; 59% had previously used prone positioning, 94% had had neuromuscular blockade, and 53% had used inhaled nitric oxide or prostacyclin. An 11% absolute reduction in 60-day mortality was found for the ECMO group (35% vs. 46%, P=0.07), with a relative risk reduction of 0.76 (95% CI, 0.55–1.04; P=0.09). (That this difference was not statistically significant may be due to the study's being underpowered, discussed below.)
In contrast, the 60-day mortality rate for the 28% (35/125) of control patients who crossed over to receive rescue ECMO was 57%. The original ECMO group began ECMO approximately 34 hours after intubation and 3 hours after randomization, whereas the rescue ECMO group crossed over later (6.5±9.7 days after randomization, approximately 5 days after the original group commenced ECMO). These results suggest an advantage for earlier versus later ECMO. Secondary analysis revealed a relative risk for treatment failure (defined as death by day 60 for the ECMO group and as either crossover to ECMO or death for the control group) of 0.62 (95% CI, 0.47–0.82; P<0.001) and a treatment-failure rate of 35% in the ECMO group versus 58% in the control arm. ECMO support lasted for 15±13 days. Delayed ECMO initiation and the high crossover rate may have confounded the 60-day mortality results.
ECMO has traditionally been associated with a high incidence of adverse events. However, in the EOLIA trial, the incidence of stroke was actually lower in the ECMO group (2% vs. 6%). Although the ECMO group had higher rates of massive transfusion (2% vs. 1%) and severe thrombocytopenia (27% vs. 16%), these rates were not prohibitively excessive, given the severity of the underlying respiratory illness. Notably, the targeted anticoagulation level was an activated partial thromboplastin time of 40–55 seconds, lower than the 60–80 seconds often used in other centers. Moreover, in an intention-to-treat analysis of secondary outcomes, the ECMO group had 20 fewer days of mechanical ventilation, 9 fewer days of vasopressor requirement, and 18 fewer days of renal replacement therapy. These data suggest that ECMO somehow decreases circulatory and renal dysfunction and may even protect against multiple organ dysfunction syndrome. In addition, during ECMO there was a 43% reduction in tidal volume and a 23% reduction in respiratory rate, while the positive end-expiratory pressure remained unchanged. This indicated a 66% reduction in mechanical power to the lungs (28 to 10 J/min), which may have promoted earlier lung recovery (39).
An often-cited, likely shortcoming of the EOLIA trial was that its power calculation was based on an expected 20% survival advantage for ECMO versus conventional medical management. This is probably an unrealistic expectation for any supportive intervention in ARDS. In the trial’s design, failure to achieve such a reduction was considered evidence of futility; thus, when a 20% reduction in mortality was not projected as an outcome after 75% (249/331) of the planned cohort was enrolled, trial recruitment was stopped early, per protocol. The underpowering of the study has been cited as the reason that the mortality difference between the ECMO and control groups did not achieve statistical significance (P=0.07) (40). Indeed, 624 patients would be required for adequate power to detect an 11% mortality reduction in the ECMO patients from the 46% mortality in the non-ECMO patients. Given EOLIA’s enrollment rate, it would have taken 9 years to complete the trial (39).
Other inherent difficulties make another EOLIA-style trial unfeasible. First, it would have been unethical to withhold crossover ECMO from patients in the non-ECMO control arm. Nonetheless, there may be value in seeking to characterize predictors of non-ECMO recovery in severe ARDS, so as to identify those patients who will not require ECMO and those patients who are at risk for conventional (non-ECMO) treatment failure and should begin ECMO earlier rather than later. Future studies could investigate whether, in patients with less-severe ARDS (PaO2/FiO2 ratio of 75–125 mmHg), earlier ECMO would improve survival and reduce dependence on ventilatory support, vasoactive medication, and renal replacement. Moreover, if lower anticoagulation levels were feasible, then some of the intrinsic risks of ECMO could be reduced, tilting the risk-to-benefit ratio in favor of earlier ECMO. Second, recruitment for the EOLIA trial was difficult, given that only 249 (24.5%) of 1015 eligible patients were enrolled. Indeed, 23% of the patients screened for EOLIA were eliminated because they had already begun ECMO, which may reflect widespread clinician acceptance of the procedure. That ECMO has a role in treating respiratory failure and severe ARDS is now well accepted; the ongoing debate centers on when ECMO should be initiated.
Mortality risk models
The use of VV-ECMO is increasing as it evolves as a therapeutic option for patients with severe ARDS. In the January 2019 ELSO registry report, 59% of adults on VV-ECMO survived to discharge (11). However, identifying the best candidates for ECMO remains challenging for clinicians. When several clinicians discuss the same patient, using a mortality risk score provides an objective assessment of the patient’s survival prospects. Moreover, identifying patients for whom treatment is likely to be futile reduces unnecessary resource utilization and helps to provide family members with a more realistic prognosis.
Several predictive mortality risk models are available to help clinicians identify those patients most likely to survive. These include the ECMOnet score developed during the H1N1 epidemic (41), the PRESERVE (PRedicting dEath for SEvere ARDS on VV-ECMO) mortality risk score (42), and the RESP (Respiratory Extracorporeal Membrane Oxygenation Survival Prediction) score developed from ELSO registry data (43) (Table 2). In our experience, the RESP score, which has a convenient online calculator and appears to be the most thoroughly validated of these models (44), provides clinical utility.
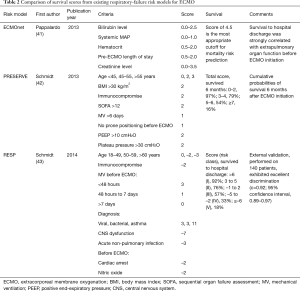
Full table
Complications of VV-ECMO
Neurological complications
Neurological complications of VV-ECMO include seizures, ischemic stroke, intracranial hemorrhage, and brain death; any of these could increase morbidity and mortality. Using ELSO registry data from 1992–2015, Lorusso et al. (45) reported a 7.1% overall incidence of neurological injury, including intracranial hemorrhage (42.5%), brain death (23.5%), ischemic stroke (19.9%), and seizures (14.1%). (The ELSO registry is voluntary, and as such is subject to the intrinsic limitations of self-reporting and consistency of definitions as interpreted by individual centers.) Pre-ECMO cardiac arrest, continuous venovenous hemofiltration, and severe hyperbilirubinemia during ECMO were associated with higher risk for neurological complications. Mechanisms of brain injury include pre-ECMO hypoxic injury, embolism, reperfusion injury, and deranged coagulation. Unless the patient has undiagnosed intracardiac shunting, the risk of embolic stroke from the circuit is lessened when the ECMO cannula is placed in the venous system instead of the venoarterial system.
Nonetheless, the incidence of neurological injury has decreased over the past two decades, possibly as a result of better patient selection, improved technology, consistent monitoring, increased clinician experience, and the need for less anticoagulation. This is consistent with findings from the EOLIA trial that showed a lower incidence of stroke in the ECMO group (16).
Bleeding/thrombosis
Bleeding is one of the most common complications in patients on VV-ECMO, occurring in approximately 16% of cases analyzed in a systematic review of 18 studies and 646 patients (46). Bleeding risk is heightened by systemic anticoagulation and coagulopathy from interaction between blood and the ECMO circuit. The 2019 ELSO Registry International Report (47) noted cannula hemorrhage in 7.8% of adults who received ECMO from 2014 to January 2019, surgical hemorrhage in 6.8%, pulmonary hemorrhage in 3.9%, and cerebral hemorrhage in 2.5%. Transfusion necessitated by bleeding also is associated with greater mortality risk. In addition, the systematic review cited above (46) found that thrombosis occurred in 53% of patients, although not all study results were clinically significant. This demonstrates the need to balance the risks of bleeding and thrombosis for each patient.
Conclusions
Over the course of several decades, survival in patients placed on ECMO for hypoxemic respiratory failure has grown from 10% in the 1970s to approximately 60% today. Extracorporeal technology has become much safer and more effective in the past five decades. Additionally, the use of ECMO for hypoxemic respiratory failure has dramatically increased in the past 10 years, owing to its success in the H1N1 pandemic and the CESAR trial. Although ECMO’s exact role and optimal timing in ARDS, and even its effect on the pathophysiology of ARDS, have not been fully determined, the recently published EOLIA trial suggests that ECMO’s role in the treatment algorithm for ARDS—and certainly severe ARDS—should be expanded, producing significantly better outcomes. The results of the studies cited here suggest that in cases of ARDS, often the final common pathway for lung injuries that cause severe hypoxemia, ECMO should be deployed early, before VILI occurs and possibly even before the onset of multiple organ dysfunction syndrome.
Acknowledgments
Jeanie F. Woodruff, BS, ELS, and Stephen N. Palmer, PhD, ELS contributed to the editing of the manuscript.
Footnote
Conflicts of Interest: The authors have no conflicts of interest to declare.
References
- Gibbon JH Jr. Artificial maintenance of circulation during experimental occlusion of pulmonary artery. Arch Surg 1937;34:1105-31. [Crossref]
- Gibbon JH Jr. Application of a mechanical heart and lung apparatus to cardiac surgery. Minn Med 1954;37:171-85. [PubMed]
- Kolff WJ, Berk HTJ, Welle M, et al. The artificial kidney: A dialyser with a great area. Acta Med Scand 1944;117:121-34. [Crossref]
- Clowes GH Jr, Hopkins AL, Neville WE. An artificial lung dependent upon diffusion of oxygen and carbon dioxide through plastic membranes. J Thorac Surg 1956;32:630-7. [PubMed]
- Lim MW. The history of extracorporeal oxygenators. Anaesthesia 2006;61:984-95. [Crossref] [PubMed]
- Rashkind WJ, Freeman A, Klein D, et al. Evaluation of a disposable plastic, low volume, pumpless oxygenator as a lung substitute. J Pediatr 1965;66:94-102. [Crossref] [PubMed]
- Bartlett RH, Gazzaniga AB, Jefferies MR, et al. Extracorporeal membrane oxygenation (ECMO) cardiopulmonary support in infancy. Trans Am Soc Artif Intern Organs 1976;22:80-93. [PubMed]
- UK Collaborative ECMO Trial Group. UK collaborative randomised trial of neonatal extracorporeal membrane oxygenation. Lancet 1996;348:75-82. [Crossref] [PubMed]
- Bartlett RH, Roloff DW, Cornell RG, et al. Extracorporeal circulation in neonatal respiratory failure: a prospective randomized study. Pediatrics 1985;76:479-87. [PubMed]
- O'Rourke PP, Crone RK, Vacanti JP, et al. Extracorporeal membrane oxygenation and conventional medical therapy in neonates with persistent pulmonary hypertension of the newborn: a prospective randomized study. Pediatrics 1989;84:957-63. [PubMed]
- Extracorporeal Life Support Organization. ECLS Registry Report International Summary. January 2019 [cited February 7, 2019]. Available online: https://www.elso.org/Portals/0/Files/Reports/2019/International%20Summary%20January%202019.pdf
- Hill JD, O'Brien TG, Murray JJ, et al. Prolonged extracorporeal oxygenation for acute post-traumatic respiratory failure (shock-lung syndrome). Use of the Bramson membrane lung. N Engl J Med 1972;286:629-34. [Crossref] [PubMed]
- Zapol WM, Snider MT, Hill JD, et al. Extracorporeal membrane oxygenation in severe acute respiratory failure. A randomized prospective study. JAMA 1979;242:2193-6. [Crossref] [PubMed]
- Morris AH, Wallace CJ, Menlove RL, et al. Randomized clinical trial of pressure-controlled inverse ratio ventilation and extracorporeal CO2 removal for adult respiratory distress syndrome. Am J Respir Crit Care Med 1994;149:295-305. [Crossref] [PubMed]
- Peek GJ, Mugford M, Tiruvoipati R, et al. Efficacy and economic assessment of conventional ventilatory support versus extracorporeal membrane oxygenation for severe adult respiratory failure (CESAR): a multicentre randomised controlled trial. Lancet 2009;374:1351-63. [Crossref] [PubMed]
- Combes A, Hajage D, Capellier G, et al. Extracorporeal membrane oxygenation for severe acute respiratory distress syndrome. N Engl J Med 2018;378:1965-75. [Crossref] [PubMed]
- Lewandowski K, Rossaint R, Pappert D, et al. High survival rate in 122 ARDS patients managed according to a clinical algorithm including extracorporeal membrane oxygenation. Intensive Care Med 1997;23:819-35. [Crossref] [PubMed]
- Kolla S, Awad SS, Rich PB, et al. Extracorporeal life support for 100 adult patients with severe respiratory failure. Ann Surg 1997;226:544-64; discussion 565-6. [Crossref] [PubMed]
- Peek GJ, Moore HM, Moore N, et al. Extracorporeal membrane oxygenation for adult respiratory failure. Chest 1997;112:759-64. [Crossref] [PubMed]
- Mattar A, Chatterjee S, Loor G. Bridging to lung transplantation. Crit Care Clin 2019;35:11-25. [Crossref] [PubMed]
- Yadav H, Thompson BT, Gajic O. Fifty years of research in ARDS. Is acute respiratory distress syndrome a preventable disease? Am J Respir Crit Care Med 2017;195:725-36. [Crossref] [PubMed]
- Bice T, Cox CE, Carson SS. Cost and health care utilization in ARDS—different from other critical illness? Semin Respir Crit Care Med 2013;34:529-36. [Crossref] [PubMed]
- Marti J, Hall P, Hamilton P, et al. One-year resource utilisation, costs and quality of life in patients with acute respiratory distress syndrome (ARDS): secondary analysis of a randomised controlled trial. J Intensive Care 2016;4:56. [Crossref] [PubMed]
- Pham T, Rubenfeld GD. Fifty years of research in ARDS. The epidemiology of acute respiratory distress syndrome. A 50th birthday review. Am J Respir Crit Care Med 2017;195:860-70. [Crossref] [PubMed]
- Parker JC, Hernandez LA, Peevy KJ. Mechanisms of ventilator-induced lung injury. Crit Care Med 1993;21:131-43. [Crossref] [PubMed]
- Dreyfuss D, Basset G, Soler P, et al. Intermittent positive-pressure hyperventilation with high inflation pressures produces pulmonary microvascular injury in rats. Am Rev Respir Dis 1985;132:880-4. [PubMed]
- Amato MB, Barbas CS, Medeiros DM, et al. Effect of a protective-ventilation strategy on mortality in the acute respiratory distress syndrome. N Engl J Med 1998;338:347-54. [Crossref] [PubMed]
- Brochard L, Roudot-Thoraval F, Roupie E, et al. Tidal volume reduction for prevention of ventilator-induced lung injury in acute respiratory distress syndrome. Am J Respir Crit Care Med 1998;158:1831-8. [Crossref] [PubMed]
- Stewart TE, Meade MO, Cook DJ, et al. Evaluation of a ventilation strategy to prevent barotrauma in patients at high risk for acute respiratory distress syndrome. N Engl J Med 1998;338:355-61. [Crossref] [PubMed]
- Brower RG, Matthay MA, Morris A, et al. Ventilation with lower tidal volumes as compared with traditional tidal volumes for acute lung injury and the acute respiratory distress syndrome. N Engl J Med 2000;342:1301-8. [Crossref] [PubMed]
- Terragni PP, Del Sorbo L, Mascia L, et al. Tidal volume lower than 6 ml/kg enhances lung protection: role of extracorporeal carbon dioxide removal. Anesthesiology 2009;111:826-35. [Crossref] [PubMed]
- Slutsky AS, Ranieri VM. Ventilator-induced lung injury. N Engl J Med 2013;369:2126-36. [Crossref] [PubMed]
- Terragni PP, Rosboch G, Tealdi A, et al. Tidal hyperinflation during low tidal volume ventilation in acute respiratory distress syndrome. Am J Respir Crit Care Med 2007;175:160-6. [Crossref] [PubMed]
- Guérin C, Reignier J, Richard JC, et al. Prone positioning in severe acute respiratory distress syndrome. N Engl J Med 2013;368:2159-68. [Crossref] [PubMed]
- Suzumura EA, Figueiró M, Normilio-Silva K, et al. Effects of alveolar recruitment maneuvers on clinical outcomes in patients with acute respiratory distress syndrome: a systematic review and meta-analysis. Intensive Care Med 2014;40:1227-40. [Crossref] [PubMed]
- Fan E, Brodie D, Slutsky AS. Acute respiratory distress syndrome: Advances in diagnosis and treatment. JAMA 2018;319:698-710. [Crossref] [PubMed]
- Davies A, Jones D, Bailey M, et al. Extracorporeal membrane oxygenation for 2009 influenza A (H1N1) acute respiratory distress syndrome. JAMA 2009;302:1888-95. [Crossref] [PubMed]
- Noah MA, Peek GJ, Finney SJ, et al. Referral to an extracorporeal membrane oxygenation center and mortality among patients with severe 2009 influenza A(H1N1). JAMA 2011;306:1659-68. [Crossref] [PubMed]
- Gattinoni L, Vasques F, Quintel M. Use of ECMO in ARDS: does the EOLIA trial really help? Crit Care 2018;22:171. [Crossref] [PubMed]
- Harrington D, Drazen JM. Learning from a trial stopped by a data and safety monitoring board. N Engl J Med 2018;378:2031-2. [Crossref] [PubMed]
- Pappalardo F, Pieri M, Greco T, et al. Predicting mortality risk in patients undergoing venovenous ECMO for ARDS due to influenza A (H1N1) pneumonia: the ECMOnet score. Intensive Care Med 2013;39:275-81. [Crossref] [PubMed]
- Schmidt M, Zogheib E, Roze H, et al. The PRESERVE mortality risk score and analysis of long-term outcomes after extracorporeal membrane oxygenation for severe acute respiratory distress syndrome. Intensive Care Med 2013;39:1704-13. [Crossref] [PubMed]
- Schmidt M, Bailey M, Sheldrake J, et al. Predicting survival after extracorporeal membrane oxygenation for severe acute respiratory failure. The Respiratory Extracorporeal Membrane Oxygenation Survival Prediction (RESP) score. Am J Respir Crit Care Med 2014;189:1374-82. [Crossref] [PubMed]
- Klinzing S, Wenger U, Steiger P, et al. External validation of scores proposed for estimation of survival probability of patients with severe adult respiratory distress syndrome undergoing extracorporeal membrane oxygenation therapy: a retrospective study. Crit Care 2015;19:142. [Crossref] [PubMed]
- Lorusso R, Gelsomino S, Parise O, et al. Neurologic injury in adults supported with veno-venous extracorporeal membrane oxygenation for respiratory failure: Findings from the extracorporeal life support organization database. Crit Care Med 2017;45:1389-97. [Crossref] [PubMed]
- Sklar MC, Sy E, Lequier L, et al. Anticoagulation practices during venovenous extracorporeal membrane oxygenation for respiratory failure. A systematic review. Ann Am Thorac Soc 2016;13:2242-50. [Crossref] [PubMed]
- Thiagarajan RR, Barbaro RP, Rycus PT, et al. Extracorporeal Life Support Organization Registry International Report 2016. ASAIO J 2017;63:60-7. [Crossref] [PubMed]