Effect of progressive sarcopenia during postoperative 6 months on long-term prognosis of completely resected lung cancer
Introduction
Cachexia is a progressive wasting syndrome that accompanies numerous diseases, such as advanced cancer, chronic obstructive pulmonary disease (COPD), and cardiac disease, all of which are characterized by muscle loss (i.e., sarcopenia) (1,2). The European Working Group on Sarcopenia in Older People (EWGSOP) proposed the definition and the criteria for diagnosis of sarcopenia (3); the diagnosis is based on low muscle mass plus low muscle strength or low physical performance. Cachexia comprises disease-related sarcopenia, which is one category of sarcopenia. Accordingly, low muscle mass is an essential element of both sarcopenia and cachexia.
The skeletal muscle index (SMI) can be determined by dividing the skeletal muscle area (SMA) at the third lumbar vertebra (L3) on computed tomography (CT) by the patient’s height in meters squared; a low SMI reflects the extent of sarcopenia (4) and is associated with prognosis in various cancers, not only in advanced stages (5-7), but also after curative resection (8,9). Recently, several reports described that preoperative sarcopenia estimated by the cut-off values of SMI or psoas muscle index, calculated in the same way as SMI for only the psoas muscle, was one of the long-term prognostic factors in patients with resected non-small cell lung cancer (NSCLC) (10-12). We have also focused on the association of SMI with long-term prognosis after complete resection of lung cancer and we identified several problems in previous studies. First, the definitions of sarcopenia characterized by cut-off values of skeletal muscle area on CT must be different between men and women. Moreover, definitions of sarcopenia were not standardized and thus varied among reports. Second, it was not effective to use the presence of sarcopenia as the only preoperative variable to evaluate the impact of surgery on the progression of postoperative sarcopenia. In addition, preoperative sarcopenia has been shown to be associated with postoperative complications and mortality (13,14). In the analysis using only preoperative sarcopenia, it is the operation-related morbidity and mortality rather than the progression of sarcopenia that can influence the results. Third, the relationship between sarcopenia, smoking history, and long-term prognosis has not been investigated in detail in previous studies. Many patients with lung cancer have a heavy-smoking history and COPD. Sarcopenia is also seen in COPD patients, which is mainly caused by increased energy expenditure due to impaired respiration; this is known as pulmonary cachexia (15-17). Compared to gastrointestinal surgery, the progression of sarcopenia after complete resection of lung cancer could be more pronounced as respiratory function is impaired by lung resection. We hypothesized that long-term sarcopenia would be seen more often in patients with a heavy-smoking history.
The currently proposed definitions and severity grading systems for both generic and cancer cachexia emphasized the body mass index (BMI) decline rate or muscle mass loss (1,2). We presume that some of the problems encountered could be resolved by analyzing the rate of change in SMI from the preoperative period to 6 months after surgery while investigating the association between SMI depletion and long-term prognosis, instead of considering preoperative sarcopenia alone. One study reported the postoperative 1-year decrease of SMI at the 12th thoracic vertebra level was significantly associated with poor prognosis in 100 patients after complete resection of stage I lung cancer (18). There was no other report about the association of postoperative SMI change with long-term prognosis of lung cancer.
The current treatments for sarcopenia do not improve long-term prognosis in these patients, despite trials that have evaluated several nutritional therapies, rehabilitative techniques, and pharmacological methods (6,19-21). We assumed the reason for these failures is that patients with advanced cancer might have advanced sarcopenia, refractory to treatment. We have previously reported that preoperative BMI and C-reactive protein (CRP) levels correlated with prognosis among smokers after complete resection of lung cancer; this is likely to be related to cachexia progression (22). Therefore, we believe that these patients might benefit from effective sarcopenia treatment in the perioperative period and could be desirable candidates for related trials, as the sarcopenic condition in these patients is still conserved. In this study, we evaluated the change in SMI after complete resection of lung cancer and tested whether a postoperative decline in SMI could predict the long-term prognosis in these patients, particularly those with a heavy- smoking history. Moreover, we explored possible measures for counteracting perioperative sarcopenia.
Methods
Patients and data collection
A total of 518 patients underwent lobectomy (excluding bilobectomy and pneumonectomy) with no pathologically residual cancer for NSCLC at the Kanagawa Cancer Center between 2007 and 2010. Anonymized data for 483 patients were available for calculating the 6-month postoperative change in SMI. During the 6 months following the operation, we excluded 10 patients due to recurrences, 4 patients due to postoperative bronchopleural fistula, and one patient due to postoperative acute respiratory distress syndrome as these diseases could affect postoperative SMI considerably. This retrospective study therefore analyzed data from 468 patients. No other inclusion or exclusion criteria were applied. The study’s protocol complied with the Declaration of Helsinki and was approved by our institutional review board (ID 2018-81). The need for patient consent was waived because all data in this study were completely anonymized.
The patients’ medical records were analyzed to determine changes in SMI and survival outcomes. The associations between these variables were evaluated using multivariate analysis, which included the patients’ clinicopathological characteristics, which were collected immediately before surgery. Most patients underwent video-assisted surgery with 10 to 15 cm posterolateral incision during the lobectomy, and no patient was treated using complete thoracoscopic surgery. We usually performed systemic hilar and mediastinal lymph node dissection according to the lymph node map presented by the International Association for the Study of Lung Cancer (23). In case of no clinical findings of lymph node metastasis (cN0), upper mediastinal lymph nodes were systemically dissected for the tumors located in the upper lobes and lower ones were done for the tumors in lower lobes. In case of any clinical findings of lymph node metastasis (cN1 or 2) or the tumors in middle lobe with cN0–2, both upper and lower mediastinal lymph nodes were systemically dissected. However, based on the clinical or surgical stage and the patient’s comorbidities, the range of lymph node dissection could be reduced to systemic hilar lymph node dissection or non-systemic lymph node sampling. Postoperative follow-up was conducted every 3 months for the first 2 years, every 6 months during the next 2 years, and then annually thereafter. Recurrence was diagnosed pathologically via biopsy or clinically based on the presence of nodules that increased in size during CT or brain magnetic resonance imaging with accumulation of 2-[18]-fluoro-2-deoxy-D-glucose during positron-emission tomography. The lung cancer board at our institution officially diagnosed all cases.
Calculating the change in SMI based on CT images
SMIs before and 6 months after the surgery were determined using plain CT slices with an interval of 5 mm. There was no standardized protocol or parameters for the CT imaging. As indicated by previous reports, the abdominal and paraspinal muscles in an axial image at L3 can be identified using boundaries of –29 to 150 Hounsfield units (4-6,24). We routinely checked and manually corrected these boundaries on the CT images. The cross-sectional muscle areas were subsequently calculated using image analysis software (SYNAPSE VINCENT®; Fujifilm Medical Co., Tokyo, Japan). The average of the cross-sectional areas from the two adjacent images was defined as the SMA (cm2), and the SMI was calculated as SMA/height2 (cm2/m2). The 6-month postoperative change in SMI (%) was calculated as [(postoperative SMI – preoperative SMI)/preoperative SMI] ×100. A single investigator (MN) who was blinded to the patients’ outcomes performed these analyses. Intra- and inter-observer coefficients of variation were not calculated.
Statistical analysis
Categorical and continuous variables that were significantly associated with survival on univariate analyses were included in the multivariate analysis, which was performed using a Cox proportional hazards model constructed using the stepwise method. The cut-off value was determined using the maximum Youden’s index value on the receiver operating characteristic (ROC) curve. Survival curves were created using the Kaplan-Meier method. All tests were two-sided and differences were considered statistically significant at P values of <0.05. All statistical analyses were performed using the JMP software package (version 12.1.0; SAS Institute, Cary, NC, USA).
Results
Postoperative prognosis and 6-month change in SMI
The median follow-up period from 6 months after the operation was 51.3 months (range, 6.3–90 months). However, follow-up was discontinued for 34 patients (7.3%). The overall survival rates were 90.6% and 80.7% at 3 and 5 years, respectively (Figure 1). A total of 77 patients died during the study. Recurrence of lung cancer, pulmonary diseases such as pneumonia, interstitial pneumonitis, and other cancers accounted for 63.6%, 15.6%, and 7.8% of the deaths, respectively. Among those who died, 24 (31%) patients did not have any lung cancer recurrence. Table 1 presents median values and ranges of the preoperative and postoperative SMIs, and the 6-month changes in SMI among men and women. There was no significant difference between men and women in terms of 6-month change in SMI (P=0.74, Mann-Whitney U analysis). The decrease in SMI did not have a significantly linear association with age (correlation coefficient =−0.05).

Full table
Association of change in SMI with postoperative mortality
Table 2 shows the patients’ characteristics and the results of the univariate survival analyses. Table 3 shows the results of the multivariate analyses, which revealed that the 6-month change in SMI as a continuous variable was independently associated with a poor prognosis.
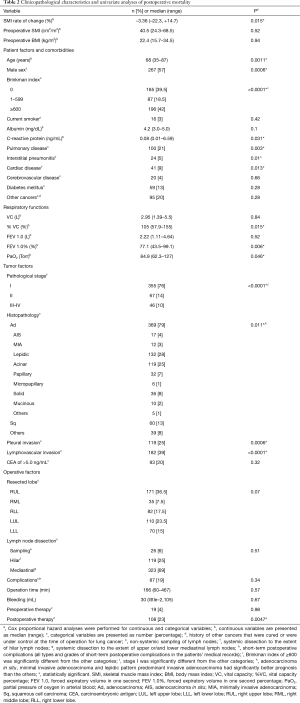
Full table
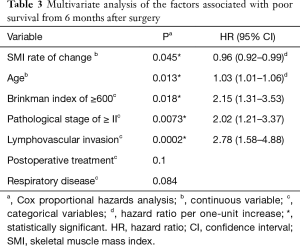
Full table
Identifying the optimal SMI change cut-off value
We randomly allocated 468 patients into two equal groups: a training set and a validation set. In the training set, based on the ROC curve analysis for mortality, the optimal cut-off value for the 6-month change in SMI was −9.9% [area under the curve (AUC): 0.57 Youden’s index: 0.20]. We then changed the cut off value for the 6-month change in SMI from −2.5% to −15% and then calculated the hazard ratio (HR). When the cut-off value was adapted as ≤−8.0%, the HR was significantly over 1 (Figure 2A). We decided −9.9% was a valid cut−off value in this study. As expected, the AUC and Youden’s index were low, because a 6-month change in SMI did not have a distinctive effect on mortality considering the length of the study. Figure 2B shows the curves for overall survival in patients with and without a change in SMI ≤−9.9% in the validation set. A change in SMI ≤−9.9% was strongly related to a poor prognosis (Table S1).
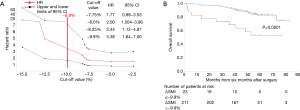
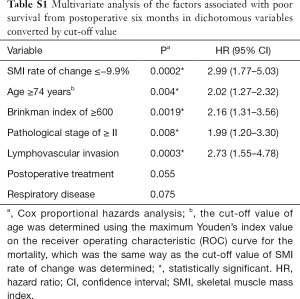
Full table
Subgroup analysis concerning smoking history
Figure 3 shows the curves for overall survival among patients with Brinkman index (BI) values of ≥600, 1–599, and 0. Patients with the highest BI category (≥600) had significantly poorer survival than that in patients in other BI categories. Tables S2 and S3 show the characteristics of patients with BI values of ≥600 and <600, as well as the results of the univariate survival analyses. Table 4 shows the results of the BI-specific multivariate analyses. Among patients with a BI of ≥600, the 6-month change in SMI was significantly associated with postoperative prognosis. However, among patients with a BI of <600, the 6-month change in SMI was not associated with mortality in the univariate analysis.
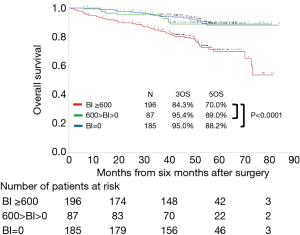
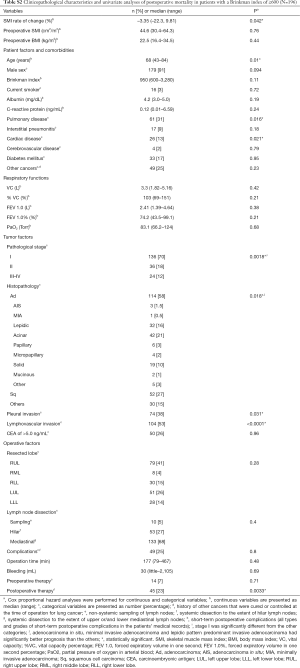
Full table
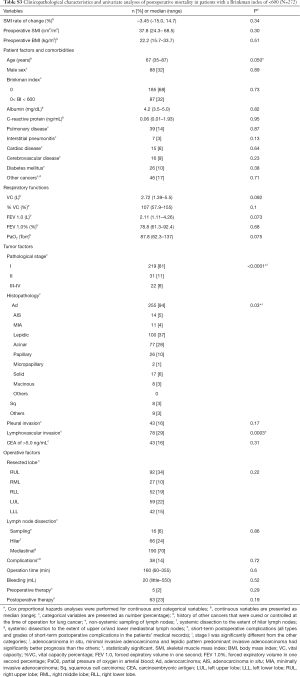
Full table
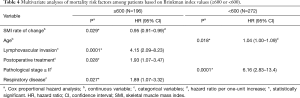
Full table
Discussion
The present study explored whether the 6-month postoperative change in SMI as a continuous variable could independently predict long-term prognosis after 6 months of complete lung cancer resection, without considering other risk factors for recurrence such as pathological stage and lymphovascular invasion, particularly in patients with a heavy-smoking history.
These results could suggest effective measures to prevent postoperative SMI decline, which could help prolong survival. This suggests the necessity of preventing perioperative progression of sarcopenia for patients after curative resection of lung cancer, especially heavy smokers. Previous studies on the association between preoperative sarcopenia and postoperative prognosis only demonstrated that sarcopenia is one of the risk factors for prognosis. Our study might pave the way for performing clinical trials to assess the impact of perioperative anti-sarcopenia treatments to improve long-term prognosis. For instance, anamorelin is a novel orally administered ghrelin receptor agonist that may improve cachexia symptoms among patients with advanced lung cancer. Various clinical studies, including a phase III clinical trial, have indicated that anamorelin treatment was associated with an increase in total and lean body weight, as well as improvements in the burden of cachexic syndrome and quality of life without severe adverse events (25-28). However, those studies failed to detect an improvement in survival. It is probably because patients with advanced cancer might have advanced cachexia that was refractory to treatment. Patients after complete resection of lung cancer, especially those who are heavy smokers, are at risk for progressive sarcopenia. However, many of their patients are not sarcopenic or at the early phase when clinical symptoms are absent [presarcopenia defined by EWGSOP (3)]; moreover these patients get cancer-free after the complete resection, ever if it may be temporary. If such patients receive treatments for muscle loss, including anamorelin, prognosis may improve. Previous studies on anamorelin in patients with advanced lung cancer revealed good tolerance and an adverse event profile that was not significantly different from that of the placebo group (25-28). Interestingly, the mechanism of action of anamorelin is not specific to cancer-related cachexia (25), which suggests that it could also be effective for pulmonary cachexia.
For the clinical trials mentioned above, heavy smokers with a BI ≥600 may be desirable candidates initially. Any related trials should incorporate requirements to cease smoking and accept nutritional and rehabilitative treatments. In the current study, patients with a BI <600 showed no significant relationship between the decrease of SMI and prognosis. However, patients with a BI <600 had substantially lower mortality than those with a BI ≥600. Similar studies that include more patients with a BI <600 could show different results.
Based on the international consensus for cachexia definition and grading, a decrease of 10% in SMI over 6 months is thought to represent severe sarcopenia that refractory to treatment (1,2). It would also be useful to identify perioperative factors associated with postoperative SMI decline. Our preliminary study revealed that for patients with a history of any other cancers, including gastric cancer (which was the most common), postoperative complications such as prolonged air leakage and long operation time were significantly associated with postoperative decline of SMI (data not shown). These are interesting findings. However, these factors were not associated with poorer long-term survival in this study. Takamori et al. reported that risk factors for skeletal muscle loss included poor performance status and forced expiratory volume (FEV) 1.0 <70% (18). The inclusion and exclusion criteria for patients in Takamori’s study are different from ours. Moreover, the postoperative 1-year decrease of SMI might be affected by the factors indifferent from the operation and perioperative managements. Additional research is imperative and may provide clues on the perioperative management of sarcopenia. There has been some speculation on whether preoperative sarcopenia indicates the presence micro-residual lesions or micro-metastases (29); however, our results showed that recurrence from 6 months after the operation and factors related to lung cancer such as pathological stage were not associated with the decrease of SMI over 6 months after surgery. In addition, there was no difference in the depletion of SMI between patients with variable smoking history. It is possible that the decline in SMI in the 6 months after surgery could be the trigger for progression of pulmonary- or cancer-related sarcopenia, or both, thereafter in patients with a heavy-smoking history.
We speculate that pulmonary sarcopenia and cancer sarcopenia cannot be considered separately in lung cancer patients with a heavy smoking history. Martin et al. have noted that both premature termination of cancer treatment and infection are possible contributors to shortened survival among patients with sarcopenia (5). A large decrease in SMI (≤–9.9%) was associated with poor survival among patients with and without recurrence in our cohort (date not shown). Many patients died because of cancer progression (lung cancer or other cancers) and respiratory diseases (e.g., pneumonia and interstitial pneumonitis), which would have been affected by sarcopenia. If recurrence or another cancer occurs, the sarcopenic tendency will be accelerated. Several reports have described sarcopenia as being associated with toxicities from many kinds of chemotherapy that are administered for cancer (30-32). In administering treatments for recurrent or other future cancers, prevention of sarcopenia may be important.
This study has several limitations. First, the study was designed to investigate the long-term prognostic factors in patients without recurrence and severe postoperative complications during a 6-month period postoperatively. However, the importance of sarcopenia may have been over-emphasized due to the study design. Unlike similar studies such as one by Nakamura et al. (12), the carcinoembryonic antigen (CEA) value in the current study was not predicative for prognosis. This was partially because we excluded the patients with early recurrence, some of which had a high CEA value. However, patients with early recurrence or severe complications must be treated intensively, and the assessment of anti-sarcopenic treatment should be considered separately in these patients. Second, variables indicating change during the postoperative 6-month period other than SMI were limited; it was therefore difficult to compare the SMI change with these limited variables. We had not obtained data on the detailed postoperative changes in BMI, CRP, albumin and other variables. Such facts might indicate that we had not before recognized the sarcopenic or nutritious aspects in the perioperative management. This is a challenge with which we will need to cope in the future. Third, the retrospective single-center design is associated with risks of bias and the small sample size limited the power of the analyses, which highlights the importance of prospective data accumulation at multiple centers. Fourth, we only considered Japanese patients and our findings may not be replicated in other patient populations. Fifth, this study did not include patients who underwent complete thoracoscopic surgery, which our hospital started to use in 2016. Few patients who underwent complete thoracoscopic surgery had a sufficient follow-up period. The effect of less invasive surgery on postoperative sarcopenia would be an interesting issue for further investigation. Finally, there is a need to continue exploring promising treatments for sarcopenia.
In conclusion, among patients who underwent complete lung cancer resection, a decrease in SMI during 6 months postoperatively significantly predicted the long-term prognosis after the 6-month period. This was particularly true among patients who were heavy smokers. Therefore, these results suggest that attention should be given to prevention concerning the perioperative progression of sarcopenia for patients after curative resection of lung cancer, especially those who are heavy smokers. Furthermore, thoracic surgeons should pursue countermeasures against perioperative sarcopenia. This study highlights the need for further research in this area.
Acknowledgments
None.
Footnote
Conflicts of Interest: The authors have no conflicts of interest to declare.
Ethical Statement: The authors are accountable for all aspects of the work in ensuring that questions related to the accuracy or integrity of any part of the work are appropriately investigated and resolved. The study’s protocol complied with the Declaration of Helsinki and it was approved by the Institutional Review Board of Kanagawa Cancer Center (ID 2018-81). The need for patient consent was waived because all data in this study were completely anonymized.
References
- Evans WJ, Morley JE, Argiles J, et al. Cachexia: a new definition. Clin Nutr 2008;27:793-9. [Crossref] [PubMed]
- Fearon K, Strasser F, Anker SD, et al. Definition and classification of cancer cachexia: an international consensus. Lancet Oncol 2011;12:489-95. [Crossref] [PubMed]
- Cruz-Jentoft AJ, Baeyens JP, Bauer JM, et al. Sarcopenia: European consensus on definition and diagnosis: Report of the European Working Group on Sarcopenia in Older People. Age Ageing 2010;39:412-23. [Crossref] [PubMed]
- Mourtzakis M, Prado CM, Lieffers JR, et al. A practical and precise approach to quantification of body composition in cancer patients using computed tomography images acquired during routine care. Appl Physiol Nutr Metab 2008;33:997-1006. [Crossref] [PubMed]
- Martin L, Birdsell L, Macdonald N, et al. Cancer cachexia in the age of obesity: skeletal muscle depletion is a powerful prognostic factor, independent of body mass index. J Clin Oncol 2013;31:1539-47. [Crossref] [PubMed]
- Jafri SH, Previgliano C, Khandelwal K, et al. Cachexia Index in Advanced Non-Small-Cell Lung Cancer Patients. Clin Med Insights Oncol 2015;9:87-93. [Crossref] [PubMed]
- Choi Y, Oh DY, Kim TY, et al. Skeletal Muscle Depletion Predicts the Prognosis of Patients with Advanced Pancreatic Cancer Undergoing Palliative Chemotherapy, Independent of Body Mass Index. PLoS One 2015;10:e0139749. [Crossref] [PubMed]
- Zheng ZF, Lu J, Zheng CH, et al. A Novel Prognostic Scoring System Based on Preoperative Sarcopenia Predicts the Long-Term Outcome for Patients After R0 Resection for Gastric Cancer: Experiences of a High-Volume Center. Ann Surg Oncol 2017;24:1795-803. [Crossref] [PubMed]
- Mayanagi S, Tsubosa Y, Omae K, et al. Negative Impact of Skeletal Muscle Wasting After Neoadjuvant Chemotherapy Followed by Surgery on Survival for Patients with Thoracic Esophageal Cancer. Ann Surg Oncol 2017;24:3741-7. [Crossref] [PubMed]
- Suzuki Y, Okamoto T, Fujishita T, et al. Clinical implications of sarcopenia in patients undergoing complete resection for early non-small cell lung cancer. Lung Cancer 2016;101:92-7. [Crossref] [PubMed]
- Deng HY, Hou L, Zha P, et al. Sarcopenia is an independent unfavorable prognostic factor of non-small cell lung cancer after surgical resection: A comprehensive systematic review and meta-analysis. Eur J Surg Oncol 2019;45:728-35. [Crossref] [PubMed]
- Nakamura R, Inage Y, Tobita R, et al. Sarcopenia in Resected NSCLC: Effect on Postoperative Outcomes. J Thorac Oncol 2018;13:895-903. [Crossref] [PubMed]
- Thomas PA, Berbis J, Falcoz PE, et al. National perioperative outcomes of pulmonary lobectomy for cancer: the influence of nutritional status. Eur J Cardiothorac Surg 2014;45:652-9. [Crossref] [PubMed]
- Miller JA, Harris K, Roche C, et al. Sarcopenia is a predictor of outcomes after lobectomy. J Thorac Dis 2018;10:432-40. [Crossref] [PubMed]
- Landbo C, Prescott E, Lange P, et al. Prognostic value of nutritional status in chronic obstructive pulmonary disease. Am J Respir Crit Care Med 1999;160:1856-61. [Crossref] [PubMed]
- Schols AM. Pulmonary cachexia. Int J Cardiol 2002;85:101-10. [Crossref] [PubMed]
- Lee SJ, Lee J, Park YS, et al. Impact of chronic obstructive pulmonary disease on the mortality of patients with non-small-cell lung cancer. J Thorac Oncol 2014;9:812-7. [Crossref] [PubMed]
- Takamori S, Toyokawa G, Okamoto T, et al. Clinical Impact and Risk Factors for Skeletal Muscle Loss After Complete Resection of Early Non-small Cell Lung Cancer. Ann Surg Oncol 2018;25:1229-36. [Crossref] [PubMed]
- Strasser F. Appraisal of current and experimental approaches to the treatment of cachexia. Curr Opin Support Palliat Care 2007;1:312-6. [Crossref] [PubMed]
- Fearon K, Argiles JM, Baracos VE, et al. Request for regulatory guidance for cancer cachexia intervention trials. J Cachexia Sarcopenia Muscle 2015;6:272-4. [Crossref] [PubMed]
- Mondello P, Mian M, Aloisi C, et al. Cancer cachexia syndrome: pathogenesis, diagnosis, and new therapeutic options. Nutr Cancer 2015;67:12-26. [Crossref] [PubMed]
- Nagata M, Ito H, Matsuzaki T, et al. Body mass index, C-reactive protein and survival in smokers undergoing lobectomy for lung cancer. Eur J Cardiothorac Surg 2017;51:1164-70. [Crossref] [PubMed]
- Rusch VW, Asamura H, Watanabe H, et al. The IASLC lung cancer staging project: a proposal for a new international lymph node map in the forthcoming seventh edition of the TNM classification for lung cancer. J Thorac Oncol 2009;4:568-77.
- Baracos VE, Reiman T, Mourtzakis M, et al. Body composition in patients with non-small cell lung cancer: a contemporary view of cancer cachexia with the use of computed tomography image analysis. Am J Clin Nutr 2010;91:1133S-1137S. [Crossref] [PubMed]
- Graf SA, Garcia JM. Anamorelin hydrochloride in the treatment of cancer anorexia-cachexia syndrome: design, development, and potential place in therapy. Drug Des Devel Ther 2017;11:2325-31. [Crossref] [PubMed]
- Currow D, Temel JS, Abernethy A, et al. ROMANA 3: a phase 3 safety extension study of anamorelin in advanced non-small-cell lung cancer (NSCLC) patients with cachexia. Ann Oncol 2017;28:1949-56. [Crossref] [PubMed]
- Nishie K, Yamamoto S, Nagata C, et al. Anamorelin for advanced non-small-cell lung cancer with cachexia: Systematic review and meta-analysis. Lung Cancer 2017;112:25-34. [Crossref] [PubMed]
- Bai Y, Hu Y, Zhao Y, et al. Anamorelin for cancer anorexia-cachexia syndrome: a systematic review and meta-analysis. Support Care Cancer 2017;25:1651-9. [Crossref] [PubMed]
- Icard P, Iannelli A, Lincet H, et al. Sarcopenia in resected non-small cell lung cancer: let's move to patient-directed strategies. J Thorac Dis 2018;10:S3138-42. [Crossref] [PubMed]
- Sjøblom B, Gronberg BH, Benth JS, et al. Low muscle mass is associated with chemotherapy-induced haematological toxicity in advanced non-small cell lung cancer. Lung Cancer 2015;90:85-91. [Crossref] [PubMed]
- Prado CM, Baracos VE, McCargar LJ, et al. Sarcopenia as a determinant of chemotherapy toxicity and time to tumor progression in metastatic breast cancer patients receiving capecitabine treatment. Clin Cancer Res 2009;15:2920-6. [Crossref] [PubMed]
- Ali R, Baracos VE, Sawyer MB, et al. Lean body mass as an independent determinant of dose-limiting toxicity and neuropathy in patients with colon cancer treated with FOLFOX regimens. Cancer Med 2016;5:607-16. [Crossref] [PubMed]