Elevation of pulmonary CD163+ and CD204+ macrophages is associated with the clinical course of idiopathic pulmonary fibrosis patients
Introduction
Idiopathic pulmonary fibrosis/usual interstitial pneumonia (IPF/UIP) is the most common subtype of idiopathic interstitial pneumonias (IIPs) with high mortality (1-3). Acute exacerbation (AE) of IPF occurs in about 8% to 14% of patients annually, resulting in rapid and lethal respiratory failure (1,4-6). Indeed, a cohort study found that AE was the most frequent cause of death in Japanese IPF patients (7). Although the pathogenesis of IPF is unclear, recent studies posited that epithelial injury and impaired wound repair are the principal etiologies (8). Macrophages, which participate in the regulation of tissue repair, regeneration, and fibrosis (9,10), can be broadly divided into two subpopulations; namely, classically activated M1-like/kill and alternatively activated M2-like/repair phenotypes, which differentiate in response to distinct microenvironmental signals and have different functions (11-13). M1-like macrophages differentiate in response to interferon-γ and produce high levels of proinflammatory cytokines (11), whereas M2-like macrophages differentiate in response to Th2 cytokines [e.g., interleukin (IL)-4, IL-13] and anti-inflammatory cytokines (e.g., IL-10) (11,12). Previous reports have suggested that M2-like macrophages produce large amounts of transforming growth factor-β1 (TGF-β1), and TGF-β1 signaling in non-activated macrophages promotes polarization towards M2-like phenotype (11,12,14-16). TGF-β1 contributes to wound healing by inducing extracellular matrix protein deposition, fibroblast activation, and cell death, suggesting that M2-like macrophages may play a central role in the fibrotic process in IPF (13,16-18). However, whether M2-like alveolar macrophages produce TGF-β1 and are involved in the pathogenesis of IPF has not yet been determined.
CD163 and CD204 are scavenger receptors and are generally regarded as M2-like macrophage markers (10-12,19). CD163 is a member of the scavenger receptor cysteine-rich family B and recognizes hemoglobin/haptoglobin complexes (20). CD163+ macrophages participate in resolution of inflammation, wound healing, and angiogenesis (21). Soluble CD163 is released into serum from the surface of stimulated macrophages through a shedding mechanism (22), and serum soluble CD163 levels have been reported to be associated with the severity of fibrosis in diseases such as liver cirrhosis and systemic sclerosis (23,24). CD204 is a member of the class A type 1 scavenger receptor family and plays a role in many processes, including lipid metabolism, atherogenesis, and suppression of the Toll-like receptor 4-mediated proinflammatory response (25). We have previously shown that expression of the M2-like macrophage markers CD163, CD204, and CD206 on alveolar cells correlates with disease severity in patients with chronic obstructive lung disease (26).
Pentraxin 2/serum amyloid protein (PTX2/SAP), a pentraxin family protein, inhibits differentiation of human peripheral blood mononuclear cells (PBMCs) into fibrocytes via activation of Fcγ receptor (27). PTX2/SAP has been reported to attenuate rat bleomycin-induced and murine TGF-β1-induced lung injury by inhibiting M2-like macrophage accumulation in the lungs (16,28,29). Notably, a recent phase 2 clinical trial demonstrated that PTX2/SAP could suppress the decline of forced vital capacity (FVC) of patients with IPF (30).
Here, we sought to clarify several questions about the involvement of M2-like macrophages in IPF by performing the following analyses: (I) immunohistochemical (IHC) staining of CD163+ and/or CD204+ cell accumulation in the lungs of patients with IPF and nonspecific interstitial pneumonia (NSIP), and their contributions to disease progression; (II) in situ hybridization (ISH) of TGF-β1 mRNA expression and ELISA analysis of TGF-β1 protein concentration in culture supernatants from human PBMC-derived macrophages in vitro to determine whether lung-associated CD163+ and/or CD204+ cells can produce TGF-β1; and (III) cell surface CD163 and CD204 expression and TGF-β1 production by human PBMC-derived macrophages in vitro to determine whether recombinant PTX2/SAP (rPTX2/SAP) inhibits the differentiation of human PBMC-derived macrophages to M2-like phenotype.
Methods
Subjects
Lung tissues were obtained by surgical lung biopsy (SLB) from 16 patients with IPF [11 males, aged 65.5 (63.3–67.0) years, IPF group] and 8 patients with NSIP [3 males, aged 52.0 (44.5–63.8) years, NSIP group] or by autopsy from 9 patients who died due to AE of IPF [9 males, aged 75.0 (66.0–77.5) years, IPF-AE group] at our or an affiliated hospital between January 1991 and August 2016. All patients were diagnosed as having IPF and/or IPF-AE by multidisciplinary discussion based on the official global guidelines for IPF (1,2). Staging of disease severity was in accordance with the gender-age-pulmonary physiology (GAP) score, which predicts IPF patient survival based on multiple factors as reported previously, i.e., classifying patients into three stages based on clinical, e.g., gender or age and physiologic, e.g., FVC and diffusing capacity of the lung for carbon monoxide (DLCO) variables (31). Patients with complicated malignant disease, infection, congestive heart failure at the day of SLB and hypersensitivity pneumonia, or connective tissue disease were excluded from the study. None of IPF or NSIP patients received drug therapy before SLB. As controls, noncancerous lung tissues were obtained from 14 patients who underwent resection for lung cancer. Overall survival (OS) was measured from the day of SLB to the final observation day (August 31, 2016). Time-to-AE (TTA) was measured from the day of SLB to the day of confirmed AE. Specific approval for all procedures was obtained from the Institutional Review Board of our hospital in accordance with the ethical standards of the Helsinki Declaration of 2013 (approval number 16070, 19 July 2016). Informed consent to participate in this study was obtained from all patients and healthy volunteers.
Laboratory analysis and pulmonary function testing
Commercially available ELISA kits were employed to measure serum concentrations of Krebs von den Lungen-6 (KL-6; EIDIA Co., Japan) and surfactant protein D (SP-D; Yamasa Co., Japan), which have been reported to be biomarkers of IPF (32-34). Pulmonary function tests, including spirometry, lung volumes and DLCO, were performed as previously described (35).
IHC staining
IHC assessment of CD68, a pan-macrophage marker and CD163, and CD204 expression in lung tissues was performed as previously reported (26). In brief, tissue sections were deparaffinized and heated in 0.01 M citrate buffer to allow antigen retrieval. Sections were incubated in 0.3% H2O2 for 10 min to block endogenous peroxidase activity and then incubated with monoclonal antibodies against CD68 (PG-M1; Dako, Glostrup, Denmark), CD163 (10D6; Novocastra, Newcastle, UK), or CD204 (SRA-E5; Transgenic, Kumamoto, Japan) overnight at 4 °C. Sections were washed and incubated with a secondary antibody (Dual Link System-HRP; Dako) for 30 min. Unbound antibody was removed, and the sections were incubated with Liquid DAB+ Substrate Chromogen System reagent (Dako, Tokyo, Japan). Adjacent serial sections were stained with hematoxylin & eosin (H&E) and Masson’s trichrome stain. The specificity of macrophage detection by anti-CD68, -CD163, and -CD204 antibody binding was confirmed by staining adjacent sections for T and B lymphocytes with anti-CD3, -CD8, and -CD20 antibodies.
The intensity and extent of immunostaining was assessed on a semi-quantitative scale as reported previously (26,36). Sections were evaluated by two investigators who were blinded to the clinical information. The number of cells in 10 randomly selected high-power fields (×400) of lung interstitium in serial sections were counted. To avoid evaluation of desquamative interstitial pneumonia (DIP)-like reaction, cells in the alveolar space were excluded (37,38). Digitized video images of the entire lung field were analyzed using a computerized color image analysis software system (BZ-9000; Keyence, Osaka, Japan).
ISH
TGF-β1 expression in lung sections was detected by mRNA ISH using an RNAscope® 2.5 HD Assay Kit-Brown (322300; Advanced Cell Diagnostics, Newark, CA, USA) with TGF-β1 mRNA-specific probes (Probe-Hs-TGFB1 400881; Advanced Cell Diagnostics), as reported previously (39).
In vitro macrophage analysis
Human PBMCs were obtained from healthy volunteers. Monocytes were isolated using a RosetteSep cocktail (StemCell, Vancouver, Canada), plated in UpCell culture plates (CellSeed, Tokyo, Japan), and cultured in medium containing 2% human serum, 1 ng/mL granulocyte macrophage-colony stimulating factor (Wako, Tokyo, Japan), and 50 ng/mL macrophage-colony stimulating factor (Wako) for 7 days to induce macrophage differentiation (40). The macrophages were then stimulated by incubation with recombinant IL-6 or IL-10 (both 10 ng/mL, Wako) for 24 hours as reported previously (19). TGF-β1 in macrophage culture supernatant was quantified using enzyme-linked immunosorbent assay (ELISA, R&D Systems, Minneapolis, MN, USA). When present, rPTX2/SAP (R&D Systems) was added to cultures at 40 ng/mL. For IHC staining, macrophages were fixed with 1% paraformaldehyde and incubated with anti-CD163 or anti-CD204 antibodies (clones AM-3K and SR-E5, respectively; Transgenic). After incubation with a secondary horseradish peroxidase-conjugated anti-mouse IgG antibody (Nichirei, Tokyo, Japan), antigen expression was visualized by incubation with 3,3'-diaminobenzidine.
Statistical analysis
Data were expressed as the median [25th to 75th percentiles of the interquartile range (IQR)]. Wilcoxon’s rank sum test or Fisher’s exact test followed by Bonferroni’s correction were used for multiple comparisons. Correlations between cell counts or ratios and clinical data were evaluated with Spearman’s rank correlation coefficient. Predictors of OS and TTA were analyzed using the log-rank test. For correlations with survival, the cell ratio cut-off values were defined as those with the highest Youden index (i.e., sensitivity + specificity –1) by receiver operating characteristic curve (ROC) analysis (41). P value <0.05 was considered statistically significant. Statistical analysis was carried out using JMP 13.0 (SAS Institute, Cary, NC, USA).
Results
Patient characteristics
The characteristics and clinical course of the study cohorts are shown in Table 1. Of the 16 patients with IPF, 9 (56%) were in GAP stage I and 7 (44%) were in stage II. DLCO/VA and PaO2 were lower in the IPF-AE group than in the IPF group. There were no significant differences in the serum concentration of KL-6 or in FVC among the IPF, IPF-AE, and NSIP groups. Eight IPF patients (50%) developed AE, and 7 (44%) died during the observation period [973.0 (283.5–1,831.5) days]. Of the IPF patients, 9 (56%) were treated with corticosteroids and/or immunosuppressants and 10 (63%) were treated with anti-fibrotic drugs, including nintedanib or pirfenidone. All 9 patients in the IPF-AE group had been treated with methylprednisolone before death from AE.
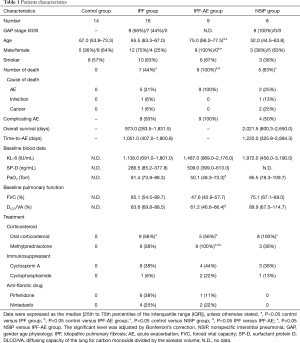
Full table
IHC analysis of lung tissues from patients with IPF or NSIP
Representative images of IHC staining in SLB samples from the control and IPF groups are shown in Figure 1A. CD68+, CD163+, and CD204+ cells were abundant in the alveolar space and lung interstitium of the IPF samples, whereas only a few cells were detectable in the control samples. Positively stained cells were not observed in bronchiolar or alveolar epithelium or fibroblastic foci in samples from either patient group. We next evaluated immature and progressive fibrotic lesions in IPF lung tissues. We found that the immature lesions contained numerous CD68+, CD163+, and CD204+ cells with sparse collagen deposition (Figure 1B, upper panel). Conversely, the progressive fibrotic lesions contained fewer CD68+, CD163+, and CD204+ cells but dense collagen deposits were evident (Figure 1B, lower panel). Analysis of the staining patterns in serial lung sections indicated that majority of CD68+ cells also express both CD163 and CD204, suggesting the presence of triple-positive CD68+CD163+CD204+ cells. Similarly, the majority of CD163+ cells appeared to co-express CD204, although a few CD163+CD204− and CD163−CD204+ cells were detectable, as reported previously (Figures 1,2) (26). Importantly, we did not observe co-staining of CD68+, CD163+, or CD204+ cells with the lymphocyte-specific markers CD3, CD8, and CD20 (Figure 2), confirming the specificity of macrophage-specific antibodies.
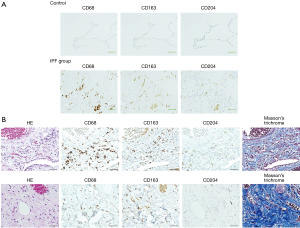
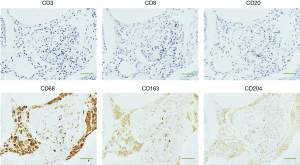
The relative abundance of CD68+, CD163+, and CD204+ cells was measured using a semi-quantitative method (Table 2). Compared with the control group samples, IPF lung samples contained significantly higher numbers of CD68+, CD163+, and CD204+ cells (all P<0.0001) as well as a significantly higher CD204+/CD68+ cell ratio [0.68 (0.53–0.74) vs. 0.23 (0.075–0.37), P=0.0006] and tended to be significantly higher CD163+/CD68+ cell ratio [0.90 (0.78–1.1) vs. 0.50 (0.30–0.93), P=0.0482]. The CD68+, CD163+, and CD204+ cell counts and CD163+/CD68+ and CD204+/CD68+ cell ratios in the IPF samples were not significantly associated with age, gender, smoking history, or GAP stage (data not shown). In the NSIP group, the numbers of CD68+, CD163+, and CD204+ cells, and the ratios of CD163+/CD68+ and CD204+/CD68+ cells were not significantly different from the corresponding values in the IPF group but were significantly higher than those in the control group.
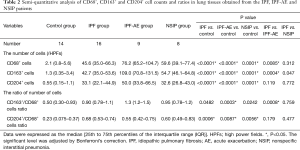
Full table
IHC analysis of lung tissues from patients with IPF-AE
Next, we examined effects of AE on cell counts and ratios. We first examined lung sections obtained from a male patient with IPF at SLB (at 64 years of age) and at autopsy after death of AE (at 65 years of age). As shown in Figure 3, CD68+, CD163+, and CD204+ cells were more abundant at the AE phase (i.e., autopsy specimen) compared with the chronic phase (SLB specimen). Semi-quantitative analysis of samples from the IPF (n=16) and IPF-AE (n=9) patient cohorts confirmed that the median numbers of CD163+ and CD68+ cells and ratio of CD163+/CD68+ cells were significantly higher in the IPF-AE group compared with the IPF group [109.0 (70.8–131.5) vs. 42.7 (35.0–53.6), 76.2 (65.2–104.7) vs. 45.6 (35.0–66.3), and 1.3 (1.2–1.5) vs. 0.90 (0.78–1.1), respectively, all P<0.01; Table 2]. However, there were no significant differences between the IPF and IPF-AE groups with respect to mean CD204+ cell count or CD204+/CD68+ cell ratio (Table 2). All of the parameters measured were significantly higher in samples from the IPF-AE group compared with the control group (Table 2). In contrast, the absolute number of CD68+, CD163+, and CD204+ cells, and the ratio of CD163+/CD68+ cells and CD204+/CD68+ cells, were not significant different between the IPF-AE patients who were treated with antifibrotic drugs and were not treated for the observation period (data not shown).
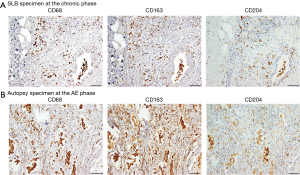
Correlations between accumulation of CD68+, CD163+, and CD204+ cells and the survival of IPF patients
Next, we analyzed whether accumulation of particular subsets of macrophages in the lungs of IPF patients was associated with TTA or OS (Figure 4). For this, patients were dichotomized into high/low CD163+/CD68+ or CD204+/CD68+ cell ratios based on the cut-off values 0.86 and 0.70, respectively, which were derived from ROC analysis. Log-rank tests revealed that high CD163+/CD68+ and CD204+/CD68+ cell ratios were both significantly associated with shorter OS (P<0.05) and shorter TTA (P<0.01 and P<0.05, respectively; Figure 4). In contrast, no significant correlations were detected between OS or TTA and CD68+, CD163+, or CD204+ cell count, age, gender, smoking history, serum KL-6 levels, pulmonary function, or drug treatments (data not shown). Thus, CD163+ and CD204+ macrophage accumulation is associated with poor prognosis in IPF patients.
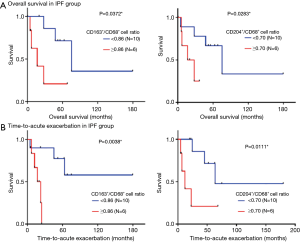
TGF-β1 production by M2-like macrophages
To determine whether lung-associated CD163+ and/or CD204+ cells can produce TGF-β1, we performed ISH of TGF-β1 mRNA in serial sections of lung tissue from the IPF group. As shown in Figure 5, TGF-β1 mRNA hybridization co-localized with IHC staining of CD163+ cells in serial sections, suggesting that pulmonary M2-like macrophages can produce TGF-β1. To confirm this, we differentiated M2-like macrophages from human PBMCs in vitro by incubation with IL-6 and IL-10, which are known inducers of CD163+ cells (42,43). Indeed, IL-6 and IL-10 stimulation significantly increased the expression of CD163, but not CD204 (Figure 6A,B). IL-6 and IL-10 also increased the production of active TGF-β1; however, this reached statistical significance only in the case of IL-10-differentiated cells (P<0.05; Figure 6C).
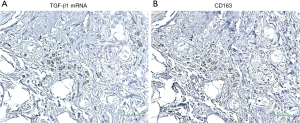
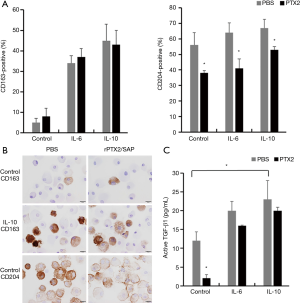
Suppressive effect of rPTX2/SAP on M2-like macrophages in vitro
Finally, we analyzed the effect of rPTX2/SAP, which has been reported to suppress M2-like macrophage differentiation in the lung (27-30), on CD163, CD204, and active TGF-β1 expression by human PBMC-derived macrophages in vitro. Notably, rPTX2/SAP suppressed the expression of CD204 in control and IL-6- or IL-10-stimulated cells, but did not influence CD163 expression (Figure 6A,B). Moreover, rPTX2/SAP significantly decreased the production of active TGF-β1 by non-stimulated macrophages, but had no effect on the production by IL-6- or IL-10-stimulated cells (Figure 6C).
Discussion
In the present study, we showed that lung tissues from IPF patients contained more CD68+, CD163+, and CD204+ cells and had higher CD163+/CD68+ and CD204+/CD68+ cell ratios than those from control group. Moreover, both cell ratios were significantly associated with OS and TTA, indicating that M2-like macrophage accumulation in the lung is associated with disease progression in IPF patients. Patients with NSIP, one of the major subtypes of IIPs, tend to have a more favorable prognosis than IPF patients, although some NSIP patients progress to end-stage fibrosis and have a poor outcome. The histological features of NSIP include uniform interstitial inflammation and fibrosis (2,44). In the present study, the accumulation of CD163+ and CD204+ cells was comparable in the lungs of NSIP and IPF patients.
Previous reports support the involvement of CD163+ and CD204+ cells in pulmonary fibrosis (45-47). Yamashita et al. showed that the density of CD163+ cells and CD163+/CD68+ cell ratio were significantly increased in lung tissues from patients with NSIP and cryptogenic organizing pneumonia, but not those with IPF, compared with control lungs (45). These results differ from those obtained in the present study, possibly because of methodological differences in cell counting. For example, Yamashita et al. included cells in the alveolar space, whereas we excluded those cells to avoid the possible influence of DIP-like reactions. These are nonspecific reactions with focal intra-alveolar accumulation of macrophages, and are associated with conditions such as smoking and environmental exposure to noxious substances (37,38). In our study, smoking history was not significantly associated with the cell counts or ratios, in contrast to a previous report showing that the CD163+/CD68+ cell ratio tends to be lower in smokers with IPF (45).
We found that TGF-β1 mRNA expression was co-localized with CD163 expression in serial sections of lung tissues from IPF patients. Furthermore, human macrophages polarized to the M2-like phenotype by exposure to IL-6 or IL-10 produced active TGF-β1 in vitro. Thus, alveolar M2-like macrophages could be a source of TGF-β1 in IPF patients and may contribute to the fibrotic process via TGF-β1 production.
Our study also demonstrated that rPTX2/SAP inhibited the expression of CD204, but not CD163, in macrophages in vitro. Beamer et al. previously showed that CD204-null mice fail to develop lung fibrosis following silica exposure (46). Stahl et al. showed that CD204 expression was required for collagen type I-induced upregulation of CC-chemokine ligand 18 (CCL18) production by alveolar macrophages from IPF patients (47). CCL18, which is regarded as an M2-like macrophage marker, is a key chemokine in promoting the fibrotic process in IPF (10,48,49). CD204+ macrophages play a critical role in the development of IPF and may be a potential therapeutic target of PTX2/SAP. Using a lung-specific TGF-β1 transgenic mouse model, Murray et al. showed that PTX2/SAP inhibited the expression of CD163 mRNA in cells isolated from bronchoalveolar lavage fluid (BALF) (16). Further study will be necessary to analyze the potential therapeutic effects of PTX2/SAP in vivo and to clarify whether the inhibitory effects of PTX2/SAP depend on CD163+ macrophages.
In the present study, we found that the ratio of CD163+/CD68+ cells, but not of CD204+/CD68+ cells, was higher in autopsied lung tissues from IPF patients at the AE phase compared with those at the chronic phase of disease. An earlier comprehensive analysis of biomarkers in BALF showed that increased levels of CCL18 could predict the development of AE in IPF patients (50). IPF-AE manifests histologically as diffuse alveolar damage with rapid progressive lung injury and structural remodeling of unknown etiology (1,4). The results presented here suggest that M2-like macrophages may be associated with the rapid progressive lung fibrosis observed in patients with IPF-AE.
This study had several limitations. First, the sample size was small, mainly because the study was conducted at only two centers. Further studies with larger populations will be needed to validate our findings. Second, this study subjects did not include patients who did not undergo SLB or autopsy. The role of M2-like macrophages in these patients should be clarified in analyses using samples taken by noninvasive procedure. Third, the in vitro analyses of PBMC samples from healthy volunteers were performed retrospectively, and it was not possible to analyze PBMC samples from patients with IIP. The cell numbers, ratios, and activities may differ between the healthy volunteers and IIP patients. However, Scott et al. suggested that elevated PBMCs is associated with poor survival in IPF patients from two independent cohorts (COMET and Yale), although its contribution to pathophysiology is unclear (51). Further analysis is needed to evaluate the functional roles of CD163+ and CD204+ cells in vitro using PBMCs obtained from IIP patients.
Conclusions
Accumulation of CD163+ and CD204+ cells in the lung is associated with shorter survival in IPF patients. The abundance of CD163+ cells, but not CD204+ cells, is higher during AE than at the chronic phase of disease. M2-like macrophage accumulation in the lungs may contribute to the progression of IPF, potentially via production of TGF-β1. Thus, our results suggest that suppression of macrophage activation and/or macrophage-derived TGF-β1 production may be therapeutic targets for IPF.
Acknowledgments
We thank Anne M. O’Rourke, PhD, from Edanz Group (www.edanzediting.com/ac) for editing a draft of this manuscript.
Footnote
Conflicts of Interest: The authors have no conflicts of interest to declare.
Ethical Statement: The authors are accountable for all aspects of the work in ensuring that questions related to the accuracy or integrity of any part of the work are appropriately investigated and resolved. Specific approval for all procedures was obtained from the Institutional Review Board of our hospital in accordance with the ethical standards of the Helsinki Declaration of 2013 (approval number 16070, 19 July 2016). Informed consent to participate in this study was obtained from all patients and healthy volunteers.
References
- Raghu G, Collard HR, Egan JJ, et al. An official ATS/ERS/JRS/ALAT statement: idiopathic pulmonary fibrosis: evidence-based guidelines for diagnosis and management. Am J Respir Crit Care Med 2011;183:788-824. [Crossref] [PubMed]
- Travis WD, Costabel U, Hansell DM, et al. An official American Thoracic Society/European Respiratory Society statement: Update of the international multidisciplinary classification of the idiopathic interstitial pneumonias. Am J Respir Crit Care Med 2013;188:733-48. [Crossref] [PubMed]
- Raghu G, Rochwerg B, Zhang Y, et al. An Official ATS/ERS/JRS/ALAT Clinical Practice Guideline: Treatment of Idiopathic Pulmonary Fibrosis. An Update of the 2011 Clinical Practice Guideline. Am J Respir Crit Care Med 2015;192:e3-19. Erratum in: Am J Respir Crit Care Med. 2015 Sep 1;192(5):644. Dosage error in article text. [Crossref] [PubMed]
- Collard HR, Moore BB, Flaherty KR, et al. Idiopathic Pulmonary Fibrosis Clinical Research Network Investigators. Acute exacerbations of idiopathic pulmonary fibrosis. Am J Respir Crit Care Med 2007;176:636-43. [Crossref] [PubMed]
- Song JW, Hong SB, Lim CM, et al. Acute exacerbation of idiopathic pulmonary fibrosis: incidence, risk factors and outcome. Eur Respir J 2011;37:356-63. [Crossref] [PubMed]
- Kim DS, Park JH, Park BK, et al. Acute exacerbation of idiopathic pulmonary fibrosis: frequency and clinical features. Eur Respir J 2006;27:143-50. [Crossref] [PubMed]
- Natsuizaka M, Chiba H, Kuronuma K, et al. Epidemiologic survey of Japanese patients with idiopathic pulmonary fibrosis and investigation of ethnic differences. Am J Respir Crit Care Med 2014;190:773-9. [Crossref] [PubMed]
- Strieter RM, Mehrad B. New mechanisms of pulmonary fibrosis. Chest 2009;136:1364-70. [Crossref] [PubMed]
- Wynn TA, Vannella KM. Macrophages in Tissue Repair, Regeneration, and Fibrosis. Immunity 2016;44:450-62. [Crossref] [PubMed]
- Mosser DM, Edwards JP. Exploring the full spectrum of macrophage activation. Nat Rev Immunol 2008;8:958-69. [Crossref] [PubMed]
- Gordon S, Martinez FO. Alternative activation of macrophages: mechanism and functions. Immunity 2010;32:593-604. [Crossref] [PubMed]
- Gordon S. Alternative activation of macrophages. Nat Rev Immunol 2003;3:23-35. [Crossref] [PubMed]
- Okuma T, Terasaki Y, Kaikita K, et al. C-C chemokine receptor 2 (CCR2) deficiency improves bleomycin-induced pulmonary fibrosis by attenuation of both macrophage infiltration and production of macrophage-derived matrix metalloproteinases. J Pathol 2004;204:594-604. [Crossref] [PubMed]
- Oishi S, Takano R, Tamura S, et al. M2 polarization of murine peritoneal macrophages induces regulatory cytokine production and suppresses T-cell proliferation. Immunology 2016;149:320-8. [Crossref] [PubMed]
- Gong D, Shi W, Yi SJ, et al. TGFβ signaling plays a critical role in promoting alternative macrophage activation. BMC Immunol 2012;13:31. [Crossref] [PubMed]
- Murray LA, Chen Q, Kramer MS, et al. TGF-beta driven lung fibrosis is macrophage dependent and blocked by Serum amyloid P. Int J Biochem Cell Biol 2011;43:154-62. [Crossref] [PubMed]
- Pohlers D, Brenmoehl J, Loffler I, et al. TGF-beta and fibrosis in different organs - molecular pathway imprints. Biochim Biophys Acta 2009;1792:746-56. [Crossref] [PubMed]
- Wahl SM, Chen W. Transforming growth factor-beta-induced regulatory T cells referee inflammatory and autoimmune diseases. Arthritis Res Ther 2005;7:62-8. [Crossref] [PubMed]
- Komohara Y, Ohnishi K, Kuratsu J, et al. Possible involvement of the M2 anti-inflammatory macrophage phenotype in growth of human gliomas. J Pathol 2008;216:15-24. [Crossref] [PubMed]
- Fabriek BO, Dijkstra CD, van den Berg TK. The macrophage scavenger receptor CD163. Immunobiology 2005;210:153-60. [Crossref] [PubMed]
- Philippidis P, Mason JC, Evans BJ, et al. Hemoglobin scavenger receptor CD163 mediates interleukin-10 release and heme oxygenase-1 synthesis: antiinflammatory monocyte-macrophage responses in vitro, in resolving skin blisters in vivo, and after cardiopulmonary bypass surgery. Circ Res 2004;94:119-26. [Crossref] [PubMed]
- Weaver LK, Hintz-Goldstein KA, Pioli PA, et al. Pivotal advance: activation of cell surface Toll-like receptors causes shedding of the hemoglobin scavenger receptor CD163. J Leukoc Biol 2006;80:26-35. [Crossref] [PubMed]
- Kazankov K, Moller HJ, Bibby BM, et al. Soluble CD163, a macrophage activation marker, is independently associated with fibrosis in patients with chronic viral hepatitis B and C. Hepatology 2014;60:521-30. [Crossref] [PubMed]
- Hassan WA, Baraka EA, Elnady BM, et al. Serum Soluble CD163 and its association with various disease parameters in patients with systemic sclerosis. Eur J Rheumatol 2016;3:95-100. [Crossref] [PubMed]
- Ohnishi K, Komohara Y, Fujiwara Y, et al. Suppression of TLR4-mediated inflammatory response by macrophage class A scavenger receptor (CD204). Biochem Biophys Res Commun 2011;411:516-22. [Crossref] [PubMed]
- Kaku Y, Komohara Y, Ohnishi K, et al. Overexpression of CD163, CD204 and CD206 on alveolar macrophages in the lungs of patients with severe chronic obstructive pulmonary disease. PLoS One 2014;9:e87400. [Crossref] [PubMed]
- Haudek SB, Trial J, Xia Y, et al. Fc receptor engagement mediates differentiation of cardiac fibroblast precursor cells. Proc Natl Acad Sci USA 2008;105:10179-84. [Crossref] [PubMed]
- Murray LA, Rosada R, Moreira AP, et al. Serum amyloid P therapeutically attenuates murine bleomycin-induced pulmonary fibrosis via its effects on macrophages. PLoS One 2010;5:e9683. [Crossref] [PubMed]
- Pilling D, Roife D, Wang M, et al. Reduction of bleomycin-induced pulmonary fibrosis by serum amyloid P. J Immunol 2007;179:4035-44. [Crossref] [PubMed]
- Raghu G, van den Blink B, Hamblin MJ, et al. Effect of Recombinant Human Pentraxin 2 vs Placebo on Change in Forced Vital Capacity in Patients With Idiopathic Pulmonary Fibrosis: A Randomized Clinical Trial. JAMA 2018;319:2299-307. [Crossref] [PubMed]
- Kolb M, Collard HR. Staging of idiopathic pulmonary fibrosis: past, present and future. Eur Respir Rev 2014;23:220-4. [Crossref] [PubMed]
- Kohno N, Awaya Y, Oyama T, et al. KL-6, a mucin-like glycoprotein, in bronchoalveolar lavage fluid from patients with interstitial lung disease. Am Rev Respir Dis 1993;148:637-42. [Crossref] [PubMed]
- Takahashi H, Fujishima T, Koba H, et al. Serum surfactant proteins A and D as prognostic factors in idiopathic pulmonary fibrosis and their relationship to disease extent. Am J Respir Crit Care Med 2000;162:1109-14. [Crossref] [PubMed]
- Ishikawa N, Hattori N, Yokoyama A, et al. Utility of KL-6/MUC1 in the clinical management of interstitial lung diseases. Respir Investig 2012;50:3-13. [Crossref] [PubMed]
- Gay SE, Kazerooni E, Toews G, et al. Idiopathic pulmonary fibrosis: predicting response to therapy and survival. Am J Respir Crit Care Med 1998;157:1063-72. [Crossref] [PubMed]
- Imaoka H, Hoshino T, Kawayama T, et al. Interleukin-18 production and pulmonary function in COPD. Eur Respir J 2008;31:287-97. [Crossref] [PubMed]
- Bedrossian CW, Kuhn C, Luna MA, et al. Desquamative Interstitial Pneumonia-Like Reaction Accompanying Pulmonary Lesions. Chest 1977;72:166-9. [Crossref] [PubMed]
- Caminati A, Harari S. Smoking-related interstitial pneumonias and pulmonary Langerhans cell histiocytosis. Proc Am Thorac Soc 2006;3:299-306. [Crossref] [PubMed]
- Loveridge CJ, Mui EJ, Patel R, et al. Increased T-cell Infiltration Elicited by Erk5 Deletion in a Pten-Deficient Mouse Model of Prostate Carcinogenesis. Cancer Res 2017;77:3158-68. [Crossref] [PubMed]
- Miyasato Y, Shiota Y, Iwase H, et al. High density of CD204-positive macrophages predicts worse clinical prognosis in patients with breast cancer. Cancer Sci 2017;108:1693-700. [Crossref] [PubMed]
- Tajiri M, Okamoto M, Fujimoto K, et al. Serum level of periostin can predict long-term outcome of idiopathic pulmonary fibrosis. Respir Investig 2015;53:73-81. [Crossref] [PubMed]
- Kowal K, Silver R, Sławińska E, et al. CD163 and its role in inflammation. Folia Histochem Cytobiol 2011;49:365-74. [Crossref] [PubMed]
- Weaver LK, Pioli PA, Wardwell K, et al. Up-regulation of human monocyte CD163 upon activation of cell-surface Toll-like receptors. J Leukoc Biol 2007;81:663-71. [Crossref] [PubMed]
- Travis WD, Hunninghake G, Martinez FJ, et al. Idiopathic nonspecific interstitial pneumonia: report of an American Thoracic Society project. Am J Respir Crit Care Med 2008;177:1338-47. [Crossref] [PubMed]
- Yamashita M, Fukuda Y, Sasamo H, et al. Distinct Profiles of CD163-Positive Macrophages in Idiopathic Interstitial Pneumonias. J Immunol Res 2018;2018:1436236. [Crossref] [PubMed]
- Beamer CA, Holian A. Scavenger receptor class A type I/II (CD204) null mice fail to develop fibrosis following silica exposure. Am J Physiol Lung Cell Mol Physiol 2005;289:L186-95. [Crossref] [PubMed]
- Stahl M, Jager B, Schmid M, et al. Lung collagens perpetuate pulmonary fibrosis via CD204 and M2 macrophage activation. PLoS One 2013;8:e81382. [Crossref] [PubMed]
- Prasse A, Kollert F, Goldmann T, et al. A vicious circle of alveolar macrophages and fibroblasts perpetuates pulmonary fibrosis via CCL18. Am J Respir Crit Care Med 2006;173:781-92. [Crossref] [PubMed]
- Prasse A, Zissel G, Toews GB, et al. Serum CC-chemokine ligand 18 concentration predicts outcome in idiopathic pulmonary fibrosis. Am J Respir Crit Care Med 2009;179:717-23. [Crossref] [PubMed]
- Schupp JC, Jager B, Cillis G, et al. Macrophage activation in acute exacerbation of idiopathic pulmonary fibrosis. PLoS One 2015;10:e0116775. [Crossref] [PubMed]
- Scott MKD, Quinn K, Li Q, et al. Increased monocyte count as a cellular biomarker for poor outcomes in fibrotic diseases: a retrospective, multicentre cohort study. Lancet Respir Med 2019;7:497-508. [Crossref] [PubMed]