Nutritional support in chronic obstructive pulmonary disease (COPD): an evidence update
Introduction
Chronic obstructive pulmonary disease (COPD) is a progressive, respiratory disease characterised by persistent airflow limitation due to damage caused by significant exposure to noxious particles or gases (1). The chronic airflow limitation is caused by a mixture of small airways disease, such as obstructive bronchitis, and parenchymal destruction seen in emphysema. Although these respiratory phenotypes will differ between individuals with the disease, there are common respiratory symptoms experienced including dyspnoea, cough and/or sputum production. Due to the increased work of breathing in COPD, even the act of eating has been observed to adversely impact haemoglobin saturation and increase dyspnea (2). These symptoms are also frequently accompanied by functional (reduced exercise tolerance) and nutrition impact symptoms (e.g., anorexia and early satiety). The aim of this review is to provide a broad update on the recent evidence for the use of nutritional support in COPD.
One of the hallmark features of COPD is the progressive decline in exercise capacity due to skeletal muscle loss and dysfunction. Metabolic adaptation in COPD has been attributed to muscle fibre-type transitioning leading to a shift in the redistribution of type-1 and type-2a fibres resulting in an increase in the susceptibility to fatigue (3). Therefore, whilst COPD was historically seen as a disease primarily affecting the lungs, the elevated and chronic systemic inflammation, and the accompanying symptomology associated with the disease, are now well known to have significant extra-pulmonary affects. These metabolic adaptations and phenotypes have now been well characterised and related to the traditional respiratory phenotypes observed in COPD (4). Certain metabolic characteristics seen in a sub-group of patients with COPD, such a depleted fat-free mass (FFM), can further negatively impact on individuals’ functional capacity, their nutritional requirements and their ability to consistently consume an adequate diet. In turn, inadequate nutritional intake to meet daily energy expenditure impacts on the availability of energy to complete activities of daily living, resulting in loss of body weight, fat mass (FM) and FFM (disuse atrophy).
Malnutrition in COPD
Unintentional weight loss and disease-related malnutrition are common problems in patients with COPD (5) and have been found to be associated with poorer quality of life (6), increased health care use and health care costs (7). However, the exact causal link between malnutrition and COPD is complicated and likely to be bidirectional, with malnutrition both a cause and consequence of severe respiratory disease. In patients with anorexia nervosa with severe nutritional depletion, diffusing capacity of the lung and respiratory muscle strength have been found to get progressively worse (8,9). Malnourished patients with COPD have been found to have greater hyperinflation, poorer lung diffusing capacity and reduced exercise tolerance when compared to non-malnourished patients with the disease (10). These physical and pathophysiological changes further negatively impact on nutritional status. Conversely, malnutrition is likely to accelerate respiratory function decline, causing loss of lung tissue as well as a reduction in the size and contractility of the muscles associated with breathing, such as the diaphragm. In the presence of malnutrition, respiratory muscles become weaker and fatigue earlier, and this may impair the ability to generate sufficient cough pressure to effectively expectorate and clear the lung of secretions that may be infected. In patients with compromised respiratory function, the combination of reduced respiratory muscle strength and early fatigue can precipitate respiratory failure or delay weaning from mechanical ventilation. In COPD patients requiring mechanical ventilation, those with a body mass index (BMI) <21 kg/m2 were found to require more time on ventilation, a longer period of hospitalisation, over double the re-intubation rate and poorer survival (11).
The aetiology of malnutrition in COPD is very complex and multi-factorial (Figure 1) including a number of metabolic and pathophysiological adaptations, such as the symptomology of COPD as well as age-related factors (e.g., loss of taste, poor dentition, anorexia) and the ability to acquire, prepare and consume an adequate diet. Important social factors such as living alone (12) and deprivation can also play an influential role both in the development of malnutrition and its management. Recently, deprivation in patients with COPD was found to be significantly associated with malnutrition risk independent of confounders such as COPD-disease severity and age (13). In addition, deprivation in COPD was associated with increased emergency hospitalisation, increased health care costs and have poorer survival (14). This highlights the importance of individualised management of malnourished patients with COPD and the consideration of factors including metabolic, pathophysiological, social and behavioural, in order to tailor nutrition interventions.
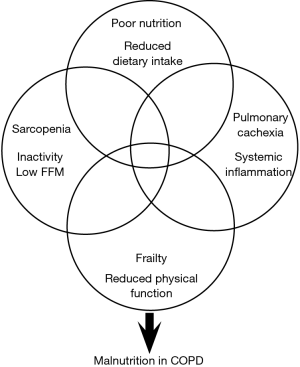
Causes of weight loss and muscle wasting in COPD
Weight loss resulting in depletion of FM occurs when energy intake is insufficient to meet daily requirements (energy imbalance) and inadequate dietary intake has been found to be common in patients with COPD (15) particularly during exacerbations of the disease (16). In contrast, muscle wasting is a consequence of an imbalance between protein synthesis and protein breakdown (negative nitrogen balance). Since weight loss in patients with COPD is typically associated with a loss of both FM and FFM stores, a negative energy balance is normally associated with a negative nitrogen balance. Importantly, during periods of catabolism, such as an infective exacerbation of COPD, inflammatory markers such as TNF-α are known to reduce food intake (17) and have also been suggested to have a role in FFM depletion in cachectic COPD patients (18). Furthermore, during acute phases of the disease, poorer respiratory function, compromised nutritional intake and increased protein breakdown can be accompanied with immobility and the need for corticosteroid medication, both of which have been associated with FFM depletion (19,20). The disease is often characterised by stable periods (non-exacerbating) which are punctuated with acute episodes of the disease that present the perfect catabolic storm, i.e., poor nutritional intake, increased protein breakdown, immobility and pharmacotherapy. This can result in patients with COPD unintentionally losing weight (FM and FFM) which they subsequently fail to regain once stable without significant intervention.
Nutrition support in COPD
Traditionally weight loss in COPD patients was considered an inevitable consequence of severe respiratory disease and as a result not amenable to nutritional intervention. In fact, it was also hypothesised that wasting was an adaptive response to the disease and that nutritional support might even be detrimental with the provision of additional substrate, particularly in the form of carbohydrate, placing a further burden on the respiratory system. However, systematic reviews and meta-analyses investigating the effectiveness of nutritional support in stable (non-exacerbating) COPD outpatients have found that malnutrition is amenable to treatment, resulting in significant improvements in both nutritional intake and nutritional status (21). In addition, improved nutritional status was found to be associated with significant improvements in functional capacity, respiratory muscle strength and quality of life (22).
Patients with COPD have been assessed to be moderately-hypermetabolic at rest, with elevated resting energy expenditure (REE) (23). However, due to the nature of the disease resulting in significant physical impairment, any increase in REE is likely to be more than accounted for by a reduction in physical activity and subsequent daily total energy expenditure (TEE) (24). Nutritional requirements for COPD patients should be assessed individually considering the patient’s clinical state (stable or exacerbation) and disease severity (mild, moderate, severe, very severe) (1) as well as their likely activity levels. Recent recommendations in relation to protein requirements and ageing suggest a daily intake of 1.0–1.2 g protein/kg body weight/day. However, older individuals who are malnourished, or those with a chronic disease, the recommended intake is 1.2–1.5 g protein/kg body weight/day (25). Energy requirements for individuals with COPD for weight maintenance can be approximately 30 kcal/kg body weight/day (26), although daily energy requirements in order to elicit weight gain are likely to be considerably higher (45 kcal/kg body weight/day) (27). Meta-analyses showed nutritional intervention resulted in a significant increase in energy intake above baseline (change in daily energy intake: +318±157 kcal/day) and this was accompanied by a significant increase in body weight (+1.83±0.26 kg, P<0.001) (21). Furthermore, improvements in respiratory (inspiratory and expiratory muscle strength) and non-respiratory (handgrip and quadriceps) muscle strength were associated with an increase in body weight of more than 2 kg (2.1–3.1 kg) (22). Interestingly, this is the magnitude of weight gain (>2 kg over 8 weeks) in depleted COPD patients that has been found to be independently associated with improved survival at 4 years. Therefore, in patients with COPD identified as malnourished, a therapeutic target for nutritional support should be an increase in weight of at least 2 kg which could be facilitated with nutritional targets of at least 45 kcal/kg body weight/day and 1.2 g protein/kg body weight/day (potentially higher if the aim is for improvements in FFM).
In addition to the strong evidence for nutritional support in stable outpatients with COPD, there is also growing evidence demonstrating the effectiveness of nutritional support in exercising patients with the disease. Two randomised controlled trials involving non-malnourished COPD patients found nutrition support, in the form of oral nutritional supplements (ONS), resulted in significant increases in body weight and exercise performance (28,29). This suggests a potential role for nutritional support beyond the treatment of malnutrition, as a way to maximise response to treatment and to treat or prevent the development of a negative energy balance that has been found to be common in those patients participating in pulmonary rehabilitation (15).
Multi-modal interventions and future directions
Due to the complexity of the nutritional and functional impairment experienced by patients with COPD, there is a need for future interventions to look beyond energy imbalance alone as it is unlikely a multi-system disease will respond to unimodal interventions (i.e., nutrition or exercise or pharmacology). It is also important that studies define what they are hoping to treat (Figure 1). There is considerable overlap between the aetiologies of malnutrition, sarcopenia and frailty, all of which can be further precipitated by periods of elevated systemic inflammation (exacerbations of COPD) and the presence of pulmonary cachexia. Cachexia is a complex syndrome that is common in diseases characterised by wasting such as COPD, chronic heart failure, chronic kidney disease and cancer. It has been suggested that pulmonary cachexia is likely in patients with unintentional weight loss or a low BMI and the presence of FFM depletion, anorexia, decreased muscle strength, fatigue and abnormal biochemistry (e.g., elevated CRP, IL-6, anaemia, hypoalbuminaemia) (30). It is frequently present in COPD and the clinical phenotype ranges from minimal or no weight loss with signs of muscle wasting, anorexia and inflammation, through to severe weight loss, significant muscle depletion (sarcopenia), fatigue and reduced physical performance (frailty). To date, nutrition and exercise intervention studies involving malnourished patients with COPD have poorly identified what nutritional aetiologies they are attempting to treat, as effective management of FFM depletion is likely to be different to that of chronic energy imbalance, frailty or cachexia. It is also likely to be heavily influenced by the timing in the delivery of the nutritional support (i.e., stable sedentary outpatients, active (exercising) outpatients, acutely unwell inpatients). Whilst there is evidence to suggest nutritional support in non-malnourished exercising patients with COPD improves outcomes (28,29) the evidence in exercising malnourished patients is far scarcer. This is likely due to firstly, concerns around further increasing negative energy balance, leading to a poor treatment response but also the ability for malnourished patients with COPD to be able to adequately participate in exercise programs. The study by Schols et al., (29) found that nutritional support in exercising malnourished COPD patients did result in a significant increase in body weight (+2.6 kg) but this was primarily due to an expansion of FM. However, the group who received a multi-modal intervention of ONS and anabolic steroids alongside the exercise program went on to gain a similar significant amount of weight but with a greater increase in FFM and improved respiratory function. A more recent study involving a cohort of underweight (BMI <19 kg/m2) COPD patients found a multi-modal intervention of polyunsaturated fatty acids (PUFA) enriched ONS (two serves providing 400 kcal/day) and low-intensity exercise for 12-week resulted in a 1.4 kg increase in weight, increased respiratory and non-respiratory muscle strength and improved quality of life (31). However, the majority of the weight gained was in the form of FM. Therefore, if the aim of the nutritional intervention is weight gain, nutritional support alone is effective but if the aim is to treat FFM depletion commonly seen in the sarcopenic and frail COPD phenotypes, then a combination of therapies is likely to be required (high-energy, high-protein, specific nutrients (e.g., leucine, PUFA), resistance training, anabolic pharmacotherapy).
The aetiology of malnutrition in stable COPD is likely primarily due to an energy imbalance (semi-starvation) and therefore it is not surprising in this patient group that nutritional support is associated with improvements. However, in COPD patients with pulmonary cachexia, more targeted methods of nutritional intervention might be required. In a recent intervention study, malnourished COPD patients were randomised to either receive a targeted intervention, providing ~230 kcal, 2-g PUFA, 10 µg 25-hydroxy-vitamin D3 daily for 12-week, or an isocaloric comparator (32). The researchers found adherence to both nutrition interventions was good (79% versus 77%) and both groups gained weight; however, biomarkers for inflammation were numerically lower in the targeted intervention group and TNF-α increased in the control group but was found to be reduced in the targeted group. In addition, exercise-induced dyspnoea and fatigue improved by a clinically relevant magnitude in the targeted nutrition group. These findings are similar to an earlier study in patients with COPD who received 9 g PUFA per day during an 8-week rehabilitation program and were found to have improved exercise capacity. However, in this study PUFA supplementation was not associated with improved markers for inflammation (33), which have been found to be the case in other studies (34).Interestingly, in the study by Broekhuizen et al. (33) patients who went on to receive additional nutrition support but who did not gain weight were found to have higher levels of TNF-α. This non-response to nutritional intervention associated with increased TNF-α levels has been observed before (35). Therefore, targeted medical nutrition therapy including PUFA might have an important synergistic effect when combined with nutritional support and exercise in improving exercise tolerance and response to nutritional interventions. Further research is needed in order to establish the potential dose-dependent mechanism of PUFA when combined with other tailored strategies for the management of malnutrition, cachexia, sarcopenia and frailty in COPD. It has been suggested that medical nutrition interventions providing adequate protein, the amino acid leucine, vitamin D and PUFA may convey added benefits in the management of sarcopenia and functional decline (36).
Vitamins
There is growing evidence that oxidative stress plays an important role in the pathology of COPD both within the lungs and other body tissues (e.g., skeletal muscle, liver). Several vitamins are able to exert potent anti-inflammatory and antioxidant effects, such as vitamins A, C and E, which are likely to be protective in the progression of COPD. BMI in patients with COPD has been found to significantly correlate with oxidative status, with a low BMI associated with reduced glutathione and increased lipid peroxidation (37). Similar to the aetiology of malnutrition in COPD, the development of micronutrient deficiencies are likely to be due to an imbalance due to increased utilisation (systematic inflammation, oxidative stress) and poor nutritional intake. Increased oxidative stress during exacerbations of the disease is linked to decreased serum concentrations of vitamin A and E (38). Vitamin E (400 IU daily for 12 weeks) has been found to reduce lipid peroxidation in COPD (39) and supplementation with vitamin A resulted in improvements in forced expiratory volume in 1 second (FEV1, +23%) and forced vital capacity (FVC, +25%) (40). Given the high prevalence of osteoporosis in COPD, vitamin D status also appears to be important due to low intakes, limited sun exposure and reduced pre-vitamin D production associated with skin ageing. Vitamin D status has been found to be significantly poorer in those COPD patients who are housebound and associated with poorer quality of life and muscle strength (41). Whilst vitamin D supplementation has not been shown to improve muscle strength, it has been found to reduce COPD exacerbation rates (42).
Essential amino acids
Amino acids are the building blocks of protein and several studies have found plasma amino acids to be reduced in COPD patients with a low body weight or depleted muscle mass (43,44). Leucine has been found to have three-times the potency of other essential amino acids in stimulating anabolic signalling in skeletal muscle (45). Therefore, it is understandable there has been renewed interest in the potential role of leucine in the management of malnutrition, sarcopenia and frailty commonly seen in COPD. The PROVIDE study involving sarcopenic older adults investigated the effectiveness of a vitamin D and leucine enriched whey protein nutritional supplement compared to an isocaloric control and found it to be associated with improved functional capacity (chair-stand test) and appendicular muscle mass (46). The fact these effects were seen with nutrition support alone, not alongside an exercise component, highlights the potential utility in patients with a limited capacity to exercise or experiencing periods of bedrest. The active leucine metabolite, beta-hydroxy-beta-methylbutyrate (HMB), has been found to prevent FFM loss during bedrest (47) and in a cohort of older hospitalised patients HMB was found to result in better odds of malnutrition treatment (48). Unfortunately, nutritional intake data was not reported so it is unclear what effect supplementation had on energy and protein intakes (total and from food) against estimated needs but at 30-day post-discharge adherence was 77% with an additional +539 kcal and +31 g protein on average being consumed via the ONS.
Body composition improvements in COPD
The combination of nutrition support, exercise training and pharmacotherapy (anabolic agents) in patients with respiratory disease has been found to result in improvements in body weight, muscle strength, exercise capacity and quality of life (29,49). Importantly, these studies also achieved increases in FFM. In the study by Pison and colleagues (49), the +1.76 kg improvement in body weight included a FFM gain of +1.47 kg. Studies that have included nutritional support and exercise without the addition of anabolic steroids (28,29,31) have found improvements in body weight, muscle strength, exercise performance and quality of life but no changes in FFM. Whereas the provision of megestrol acetate and testosterone alone in COPD patients with cachexia has been found to be associated with significant weight gain including both FM and FFM (50).
The consistent findings of nutrition support studies demonstrating improvements in functional capacity and quality of life with improved body weight, regardless of the composition of the weight gained (FM or FFM) warrants further exploration. There is also a need for future intervention studies to be explicit in what form of nutritional depletion they are seeking to treat as the current evidence base suggests cachectic COPD patients require anabolic agents in order to ensure weight gain favours improvements in FFM. There also needs to be better reporting of both exercise and nutrition intervention studies in COPD with detail on the types (e.g., resistance), load (e.g., percentage 1-repetition maximum, number of repetitions) and frequency of exercise as well as whether nutritional intervention achieves pre-defined nutritional targets to promote weight gain (e.g., 45 kcal/kg body weight/day and 1.2–1.5 g protein/kg/day). This should encourage nutrition and exercise interventions that are tailored to the individual rather than a one-size-fits-all approach that has been common to date. With this in mind it is very likely we are yet to realise the true effect of nutritional support in managing the many faces of nutritional depletion in COPD.
Conclusions
Nutritional depletion in COPD is common, complex with a number of characterisable phenotypes that to various extents are associated with increased health care use, reduced functional capacity and poorer clinical outcomes. Although nutritional depletion was historically seen as an untreatable epiphenomenon of the disease and therefore not responsive to intervention, both our understanding of the aetiology of the depletion and the evidence for targeted nutritional interventions have improved considerably. The future challenge for the field of clinical nutrition in the management of malnutrition in COPD, is to better identify and characterise the specific targets of nutritional intervention (energy imbalance, sarcopenia, cachexia, and frailty) which can then inform better targeted interventions. COPD is a treatable chronic disease but a dynamic one where periods of stability are punctuated by episodes of acute exacerbations leading to poor nutritional intake, increased inflammation and immobility. Therefore, future studies are needed exploring the effectiveness of targeted multimodal interventions that take a disease-course approach ensuring the right patients, receive the right nutrition support, at the right time, in order to maximise the response to treatment. There is growing scientific evidence that the many faces of malnutrition seen in COPD are treatable, but many treatment questions remain with regards to what, when and how to intervene.
Acknowledgments
Funding: A Vaughan is supported by The Prince Charles Hospital Foundation Research Fellowship and Innovation grant (RF2017-05 and INN2018-30). IA Yang is supported by a National Health and Medical Research Council of Australia Project grant (APP1121740).
Footnote
Conflicts of Interest: The authors have no conflicts of interest to declare.
Ethical Statement: The authors are accountable for all aspects of the work in ensuring that questions related to the accuracy or integrity of any part of the work are appropriately investigated and resolved.
References
- GOLD. Global strategy for the diagnosis, management, and prevention of chronic obstructive pulmonary disease (2019 Report). 2019.
- Schols A, Mostert R, Cobben N, et al. Transcutaneous oxygen saturation and carbon dioxide tension during meals in patients with chronic obstructive pulmonary disease. Chest 1991;100:1287-92. [Crossref] [PubMed]
- Gosker HR, van Mameren H, van Dijk PJ, et al. Skeletal muscle fibre-type shifting and metabolic profile in patients with chronic obstructive pulmonary disease. Eur Respir J 2002;19:617-25. [Crossref] [PubMed]
- Schols AM, Ferreira IM, Franssen FM, et al. Nutritional assessment and therapy in COPD: a European Respiratory Society statement. Eur Respir J 2014;44:1504-20. [Crossref] [PubMed]
- Hogan D, Lan LT, Diep DT, et al. Nutritional status of Vietnamese outpatients with chronic obstructive pulmonary disease. J Hum Nutr Diet 2017;30:83-9. [Crossref] [PubMed]
- Nguyen HT, Collins PF, Pavey TG, et al. Nutritional status, dietary intake, and health-related quality of life in outpatients with COPD. Int J Chron Obstruct Pulmon Dis 2019;14:215-26. [Crossref] [PubMed]
- Hoong JM, Ferguson M, Hukins C, et al. Economic and operational burden associated with malnutrition in chronic obstructive pulmonary disease. Clin Nutr 2017;36:1105-9. [Crossref] [PubMed]
- Gardini Gardenghi G, Boni E, Todisco P, et al. Respiratory function in patients with stable anorexia nervosa. Chest 2009;136:1356-63. [Crossref] [PubMed]
- Coxson HO, Chan IH, Mayo JR, et al. Early emphysema in patients with anorexia nervosa. Am J Respir Crit Care Med 2004;170:748-52. [Crossref] [PubMed]
- Ezzell L, Jensen GL. Malnutrition in chronic obstructive pulmonary disease. Am J Clin Nutr 2000;72:1415-6. [Crossref] [PubMed]
- Li-dong S, Chang-sheng G, Zi-yu Z. Explore the influence of BMI in the optimal time of weaning from sequential mechanical ventilation for severity chronic obstructive pulmonary disease. BMC Emerg Med 2013;13 Suppl 1:S1. [Crossref] [PubMed]
- Hanna KL, Collins PF. Relationship between living alone and food and nutrient intake. Nutr Rev 2015;73:594-611. [Crossref] [PubMed]
- Collins PF, Elia M, Kurukulaaratchy RJ, et al. The influence of deprivation on malnutrition risk in outpatients with chronic obstructive pulmonary disease (COPD). Clin Nutr 2018;37:144-8. [Crossref] [PubMed]
- Collins PF, Stratton RJ, Kurukulaaratchy RJ, et al. Influence of deprivation on health care use, health care costs, and mortality in COPD. Int J Chron Obstruct Pulmon Dis 2018;13:1289-96. [Crossref] [PubMed]
- Holst M, Beck AM, Rasmussen HH, et al. Insufficient intake of energy and protein is related to physical functional capacity among COPD patients referred to municipality based pulmonary rehabilitation. Clin Nutr ESPEN 2019;30:35-41. [Crossref] [PubMed]
- Vermeeren MA, Schols AM, Wouters EF. Effects of an acute exacerbation on nutritional and metabolic profile of patients with COPD. Eur Respir J 1997;10:2264-9. [Crossref] [PubMed]
- Langhans W, Hrupka B. Interleukins and tumor necrosis factor as inhibitors of food intake. Neuropeptides 1999;33:415-24. [Crossref] [PubMed]
- Eagan TM, Gabazza EC, D'Alessandro-Gabazza C, et al. TNF-alpha is associated with loss of lean body mass only in already cachectic COPD patients. Respir Res 2012;13:48. [Crossref] [PubMed]
- Dirks ML, Wall BT, van de Valk B, et al. One Week of Bed Rest Leads to Substantial Muscle Atrophy and Induces Whole-Body Insulin Resistance in the Absence of Skeletal Muscle Lipid Accumulation. Diabetes 2016;65:2862-75. [Crossref] [PubMed]
- Saudny-Unterberger H, Martin JG, Gray-Donald K. Impact of nutritional support on functional status during an acute exacerbation of chronic obstructive pulmonary disease. Am J Respir Crit Care Med 1997;156:794-9. [Crossref] [PubMed]
- Collins PF, Stratton RJ, Elia M. Nutritional support in chronic obstructive pulmonary disease: a systematic review and meta-analysis. Am J Clin Nutr 2012;95:1385-95. [Crossref] [PubMed]
- Collins PF, Elia M, Stratton RJ. Nutritional support and functional capacity in chronic obstructive pulmonary disease: a systematic review and meta-analysis. Respirology 2013;18:616-29. [Crossref] [PubMed]
- Nguyen LT, Bedu M, Caillaud D, et al. Increased resting energy expenditure is related to plasma TNF-alpha concentration in stable COPD patients. Clin Nutr 1999;18:269-74. [Crossref] [PubMed]
- Baarends EM, Schols AM, Westerterp KR, et al. Total daily energy expenditure relative to resting energy expenditure in clinically stable patients with COPD. Thorax 1997;52:780-5. [Crossref] [PubMed]
- Deutz NE, Bauer JM, Barazzoni R, et al. Protein intake and exercise for optimal muscle function with aging: recommendations from the ESPEN Expert Group. Clin Nutr 2014;33:929-36. [Crossref] [PubMed]
- Slinde F, Gronberg AM, Svantesson U, et al. Energy expenditure in chronic obstructive pulmonary disease-evaluation of simple measures. Eur J Clin Nutr 2011;65:1309-13. [Crossref] [PubMed]
- Ganzoni A, Heilig P, Schonenberger K, et al. High-caloric nutrition in chronic obstructive lung disease. Schweiz Rundsch Med Prax 1994;83:13-6. [PubMed]
- Steiner MC, Barton RL, Singh SJ, et al. Nutritional enhancement of exercise performance in chronic obstructive pulmonary disease: a randomised controlled trial. Thorax 2003;58:745-51. [Crossref] [PubMed]
- Schols AM, Soeters PB, Mostert R, et al. Physiologic effects of nutritional support and anabolic steroids in patients with chronic obstructive pulmonary disease. A placebo-controlled randomized trial. Am J Respir Crit Care Med 1995;152:1268-74. [Crossref] [PubMed]
- Evans WJ, Morley JE, Argiles J, et al. Cachexia: a new definition. Clin Nutr 2008;27:793-9. [Crossref] [PubMed]
- Sugawara K, Takahashi H, Kasai C, et al. Effects of nutritional supplementation combined with low-intensity exercise in malnourished patients with COPD. Respir Med 2010;104:1883-9. [Crossref] [PubMed]
- Calder PC, Laviano A, Lonnqvist F, et al. Targeted medical nutrition for cachexia in chronic obstructive pulmonary disease: a randomized, controlled trial. J Cachexia Sarcopenia Muscle 2018;9:28-40. [Crossref] [PubMed]
- Broekhuizen R, Wouters EF, Creutzberg EC, et al. Polyunsaturated fatty acids improve exercise capacity in chronic obstructive pulmonary disease. Thorax 2005;60:376-82. [Crossref] [PubMed]
- Matsuyama W, Mitsuyama H, Watanabe M, et al. Effects of omega-3 polyunsaturated fatty acids on inflammatory markers in COPD. Chest 2005;128:3817-27. [Crossref] [PubMed]
- Creutzberg EC, Schols AM, Weling-Scheepers CA, et al. Characterization of nonresponse to high caloric oral nutritional therapy in depleted patients with chronic obstructive pulmonary disease. Am J Respir Crit Care Med 2000;161:745-52. [Crossref] [PubMed]
- Tessier AJ, Chevalier S. An Update on Protein, Leucine, Omega-3 Fatty Acids, and Vitamin D in the Prevention and Treatment of Sarcopenia and Functional Decline. Nutrients 2018;10. [Crossref] [PubMed]
- Vibhuti A, Arif E, Deepak D, et al. Correlation of oxidative status with BMI and lung function in COPD. Clin Biochem 2007;40:958-63. [Crossref] [PubMed]
- Tug T, Karatas F, Terzi SM. Antioxidant vitamins (A, C and E) and malondialdehyde levels in acute exacerbation and stable periods of patients with chronic obstructive pulmonary disease. Clin Invest Med 2004;27:123-8. [PubMed]
- Daga MK, Chhabra R, Sharma B, et al. Effects of exogenous vitamin E supplementation on the levels of oxidants and antioxidants in chronic obstructive pulmonary disease. J Biosci 2003;28:7-11. [Crossref] [PubMed]
- Paiva SA, Godoy I, Vannucchi H, et al. Assessment of vitamin A status in chronic obstructive pulmonary disease patients and healthy smokers. Am J Clin Nutr 1996;64:928-34. [Crossref] [PubMed]
- Carson EL, Pourshahidi LK, Madigan SM, et al. Vitamin D status is associated with muscle strength and quality of life in patients with COPD: a seasonal prospective observation study. Int J Chron Obstruct Pulmon Dis 2018;13:2613-22. [Crossref] [PubMed]
- Jolliffe DA, Greenberg L, Hooper RL, et al. Vitamin D to prevent exacerbations of COPD: systematic review and meta-analysis of individual participant data from randomised controlled trials. Thorax 2019;74:337-45. [Crossref] [PubMed]
- Engelen MP, Wouters EF, Deutz NE, et al. Factors contributing to alterations in skeletal muscle and plasma amino acid profiles in patients with chronic obstructive pulmonary disease. Am J Clin Nutr 2000;72:1480-7. [Crossref] [PubMed]
- Yoneda T, Yoshikawa M, Fu A, et al. Plasma levels of amino acids and hypermetabolism in patients with chronic obstructive pulmonary disease. Nutrition 2001;17:95-9. [Crossref] [PubMed]
- Atherton PJ, Smith K, Etheridge T, et al. Distinct anabolic signalling responses to amino acids in C2C12 skeletal muscle cells. Amino Acids 2010;38:1533-9. [Crossref] [PubMed]
- Bauer JM, Verlaan S, Bautmans I, et al. Effects of a vitamin D and leucine-enriched whey protein nutritional supplement on measures of sarcopenia in older adults, the PROVIDE study: a randomized, double-blind, placebo-controlled trial. J Am Med Dir Assoc 2015;16:740-7. [Crossref] [PubMed]
- Deutz NE, Pereira SL, Hays NP, et al. Effect of beta-hydroxy-beta-methylbutyrate (HMB) on lean body mass during 10 days of bed rest in older adults. Clin Nutr 2013;32:704-12. [Crossref] [PubMed]
- Deutz NE, Matheson EM, Matarese LE, et al. Readmission and mortality in malnourished, older, hospitalized adults treated with a specialized oral nutritional supplement: A randomized clinical trial. Clin Nutr 2016;35:18-26. [Crossref] [PubMed]
- Pison CM, Cano NJ, Cherion C, et al. Multimodal nutritional rehabilitation improves clinical outcomes of malnourished patients with chronic respiratory failure: a randomised controlled trial. Thorax 2011;66:953-60. [Crossref] [PubMed]
- Casaburi R, Nakata J, Bistrong L, et al. Effect of Megestrol Acetate and Testosterone on Body Composition and Hormonal Responses in COPD Cachexia. Chronic Obstr Pulm Dis 2015;3:389-97. [Crossref] [PubMed]