Cardiovascular magnetic resonance assessment of biventricular changes during vacuum bell correction of pectus excavatum
Introduction
Pectus excavatum (PE), the inward displacement of the sternum and adjacent costal cartilages—is the most common congenital chest wall deformity with an incidence of 1:400 and a male-to-female ratio of 5:1 (1). Symptoms associated with PE include dyspnea, loss of endurance, chest pain during exercise in addition to psychological complaints (2). During exercise, patients with PE have demonstrated poorer cardiopulmonary performance as compared with healthy controls (3-5). Furthermore, when evaluated with cardiovascular magnetic resonance imaging (CMRI), cardiac function is restricted, and seen as reduced right ventricular ejection fraction (RV EF) (6,7). In recent decades, corrective surgery performed for PE has increased considerably; nevertheless, the indications for surgery remain ill defined (8,9).
A growing number of studies have been published describing the physiological benefits of PE correction, both in the short and long-term (6,10-14). Among physiological parameters reported to improve post operatively, the most significant are size and volumes of cardiac chambers and self-reported improvement in exercise tolerance. Nonetheless, surgical correction of PE is still regarded by many as a purely cosmetic procedure.
Possible explanations for the lack of consensus regarding surgical indications could be ascribed to the nature of the clinical studies, often reporting both on pulmonary and cardiac parameters (15). While several authors have reported improvements, albeit relatively small, in cardiac function after PE correction both at rest and during exercise, and independent of the surgical technique employed (11,13,14,16), the evidence on pulmonary function improvement remains inconclusive.
It was further observed that the results of several clinical studies reporting on cardiac function following surgery may have been confounded by factors such as growth during the investigation period, psychological changes and lifestyle modifications, with changing physical activity levels.
The golden standard for assessing cardiac parameters such as biventricular volumes and function (17,18) is CMR, occasional reports have been published on the use of MRI to assess cardiac function after PE correction (6,7), however chest metal implants may cause MRI artifacts and patient discomfort.
A strategy to assess the immediate effects of the PE-correction on heart function in a non-invasive way is by applying the vacuum bell (VB), which is a CMR-safe, silicon device, designed to correct the deformity with immediate effect (19) (Figure 1). The VB was originally developed for the conservative treatment of PE; however, long term outcomes have suggested that it was not a reliable treatment option for all patients, particularly in the adult population (19-23). Nonetheless, the device can be successfully used during the minimally invasive repair of pectus excavatum (MIRPE) to elevate the sternum, facilitating bar introduction and reducing intraoperative risk of heart injury (24,25). In this prospective, clinical study, the immediate, temporary effect of PE-correction on cardiac function was evaluated with CMRI, using the VB on patients with PE.
Methods
Study population
This study was a prospective, interventional, single-center study carried out at Humanitas Research Hospital, Rozzano, Milan, Italy. Patients who were scheduled for surgical correction of PE with the Nuss procedure between January 2015 and January 2016 were included. Regional health system criteria for reimbursement of surgical correction of PE included: age <40 years; presence of psychological impairment; a Haller index (HI) ≥3.5 as verified on computed tomography (8,26). Patients were excluded if they suffered from claustrophobia or had other clinical contraindications to undergoing CMRI or VB application, including: osteogenesis imperfecta, Glisson’s disease, Marfan syndrome, and coagulopathies (23). A healthy control group, matched for age and body surface area (BSA), was included. The controls were excluded if they had chest wall deformities or any cardiovascular or pulmonary diseases.
This study was conducted in accordance with the amended Helsinki declaration and received Approval of the local ethics committee. All participants provided written informed consent.
Cardiovascular magnetic resonance imaging
CMRI was performed using a 1.5 Philips Achieva magnetic resonance scanner (Philips, Amsterdam, The Netherlands) equipped with 30 mT/m gradients, a slew rate of 80 mT/m/msec, software release 5.17, and a 16-channel torso surface coil. During the entire session, the heart rhythm was monitored with a four-electrode vectorcardiography system. Electrodes were placed on the lateral or posterior chest wall to allow correct adhesion of the VB device. The CMR protocol was designed according to the Society of Cardiovascular Magnetic Resonance image acquisition protocols (27): first, standard scout images of the heart and surroundings were obtained followed by axial single-shot steady state free precession (SSFP) images and pilots to line-up cines. SSFP cine images of four-chamber, two-chamber and short axis stacks were obtained, ensuring that both ventricles were covered using slice thickness of 8 mm and slice gap of 2 mm. Flow measurement for the calculation of stroke index (SI) was acquired using phase-contrast scans. Transverse images of the ascending aorta and the pulmonary trunk were obtained covering 40 cardiac phases. Two identical series of CMRI were performed on all participants: Baseline CMR and CMR during VB application, where SSFP and phase contrast sequences were repeated using the same protocol. Both studies were performed using the same initial localizer.
VB application
The VB was applied to the thorax at the end of baseline CMRI. The device covered the thoracic depression and the superior borders of the sternum in healthy controls; ensuring that the internal contours of the suction cup adhered firmly to the skin. Once applied, a negative pressure of up to 15% below atmospheric pressure was manually created using the pump of the device. Following the second CMRI during VB application, the effect of the VB was terminated, relieving the negative pressure within the suction cup immediately.
Data analysis
A single independent, non-blinded observer performed offline CMRI analysis using dedicated software (CVI42, Circle Cardiovascular Imaging Inc., Calgary, Canada). Manual tracing of the left and right endocardium was performed according to current guidelines to calculate end-diastolic volume index (EDVI), end-systolic volume index (ESVI), EF, SI and cardiac output (CO) of both right and left ventricles (LV) (28). All measurements were normalized to BSA and a comparative analysis between baseline and VB values was performed. The SI was calculated on phase contrast sequences and was measured in the ascending aorta and pulmonary trunk before and during VB application. The possible changes between baseline measurements and following VB application were measured in all participants as biventricular mean percentage change ± standard deviation (± SD) using the following formula:
(VB mean value-baseline mean value)/baseline mean value × 100
Investigations were undertaken as to whether baseline biventricular parameters could predict the hemodynamic response to VB application, in terms of final change in biventricular volumes, EF and SI, respectively.
Statistical analysis
Continuous, normally distributed data were reported as means ± SD. Wilcoxon test was used for comparisons between measurements of patients and controls, and the paired Mann Whitney test was used for comparisons between baseline and VB values. Prior to study start, power calculations were conducted for the hemodynamic indices to ensure the number of participants
We expected a variation of 20% as measured with CMR. Considering a type-one error of 5%, a 2 cohort of 16 patients was considered enough to reveal the expected 20% change with a power of 90%, exemplified with cardiac index improving from 6.5 to 8 L/min, considering a common ± SD of 1.7 L/min. A P value <0.05 was considered statistically significant. Statistical analyses were performed using StataIC 13 (StataCorp LP, College Station, TX, USA).
Results
Study population
A total of 21 patients and 10 healthy controls were included in the study. One patient with PE suffering from claustrophobia was excluded from the study. The 20 remaining patients and 10 controls exhibited similar clinical characteristics. The demographic parameters are displayed in Table 1.
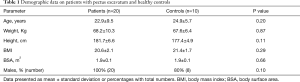
Full table
CMR before and during VB application
All CMR scans of the participants were included for analysis, the biventricular volumes and function are displayed in Table 2. Baseline cardiac parameters revealed lower values in RV SI for patients (P<0.05) and a tendency for a lower LV SI (P=0.05) as compared with controls. Other baseline cardiac parameters showed no significant difference. Following VB application, patients demonstrated increases in RV EF 7%±9% and SI 11%±9% (both P<0.01). No changes were noted in controls. The LV parameters of the patients similarly revealed improvement with increasing EDVI 8%±7%, SI 11%±9%, and EF 3%±4% (all P<0.05). No changes were found in either RV or LV parameters for the controls except for a 5% decrease in LV SI following VB application (P=0.04). The cardiac parameters changes of the RV and LV are displayed in Figures 2 and 3. Examples of CMR images displaying changes in RV volumes from baseline position of the sternum to VB application are presented in Figure 4, demonstrating relief of the sternal compression at the level of the right atrioventricular groove in two patients.
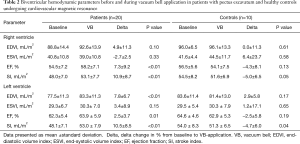
Full table
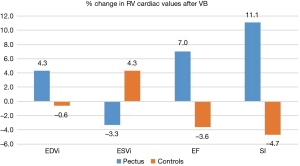
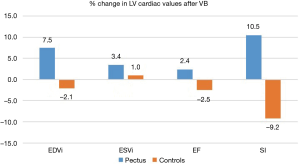
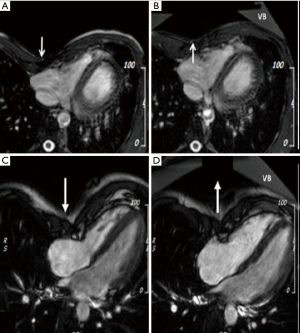
Adverse events
The brief VB-application of 20-to-30 minutes presented few adverse events. The most common was local reddening of the skin below the suction cup, observed in six patients and three controls, which resolved spontaneously in all cases. Chest discomfort was also described by a few participants, with complete relief following device removal. No participant reported pain.
Discussion
To the best of our knowledge, this is the only study that shows an immediate improvement in cardiac function using a simple non-invasive device to obtain temporary sternal elevation in PE patients scheduled for surgical correction. The study was designed to simulate the effects of surgical correction with the VB, a CMRI-safe noninvasive device.
Data obtained from each patient prior to the application of the VB served as their own control versus post-application parameters; confounding factors such as imaging artifacts due to the presence of metal implants, patients growth or lifestyle changes after surgery are avoided and the sole influence of lifting the depressed sternum was assessed. A group of healthy subjects underwent the same procedure, in order to assess unforeseen cardiovascular effects of sternal elevation, or of the negative pressure application per se. Our data show an immediate improvement in biventricular SI and in biventricular EF in PE patients during temporary relief of chest wall-depression. No significant variation was observed in healthy subjects undergoing the same procedure.
The study demonstrated an immediate measurable change in cardiac parameters similar to those previously described by both short- and long-term follow-up studies post-surgical repair of PE. In a long-term follow-up study from 2013, O’Keefe et al. investigated the effects of surgical correction of PE on cardiac function at rest and found increases in both CO and stroke volume (29). The authors reported that the mean preoperative stroke volume increased from 68.1 to 75.0 mL three months after Nuss-bar removal. Surgical correction carried an estimated change of 10%, in line with the right and left ventricular mean SI changes of 11% in our non-invasive study. Other Authors have also noted improvements in cardiac function following corrective surgery for PE. In a study from 2008, Hu et al. saw a 23% increase in LV stroke volume in 40 children, 4.2 years post PE-surgery (30). This was compared with a group of 38 healthy children without PE, investigated in the same period of time, in whom a 17% increase in stroke volume was observed, which could be attributed to growth. Hence, approximately 5% may be credited the corrective surgery. In a recent study with CMRI, Töpper et al. looked at the surgical impact on cardiac function in 38 adult patients with PE (6). The patients demonstrated significant increases in their RV stroke volume (approximately 6%) and LV stroke volume (5%), more than one-year post-surgery. Another important finding from this study was a significant improvement in both RV and LV systolic function of the patients, but not the controls who displayed a 5% decrease in LV SI following VB application, likely due to the excessive displacement of the sternum impeding optimal cardiac contraction.
Gürkan et al. performed transthoracic echocardiography on a group of 16 male adults before and one month after PE repair (14) and observed significant increases in RV diastolic diameter, systolic excursion velocity and tricuspid annular plane systolic excursion, suggesting improved systolic function of the RV (14). This was in line with the findings of improved RV EF of our study. In the Topper study, the authors also reported on EF of the 38 adult PE-patients and found a reduced RV EF prior to surgery, with normalized, significantly increased RV EF more than a year after surgery (6). RV EF was approximately increased by 6% one year after surgery, whereas the LV EF increased significantly by approximately 3%. These improvements in EF are reflected in our acute study, where RV EF was increased by 7% and LV EF by 3% with sternal elevation. Immediate changes in cardiac parameters following surgical correction of PE have been reported previously (13,31). Cardiac parameters were examined by intraoperative transesophageal echocardiography and the authors noted increases in RV volume, area and diameter as well as LV EF.
The accuracy and reproducibility of CMRI is well established (17), making it the gold standard, cost effective technique. According to a seminal study (32), the adequate sample size to detect a 3% change in EF with CMRI is as few as 15 patients. Our observed change in LVEF was 7%, beyond the variability of the adopted diagnostic approach: therefore, it should be attributed to a measurable effect of the intervention.
We compared the cardiac measurements with that of a recently published study on CMR reference values, determined from a population of healthy, Italian adults (33). Ten patients (50%) showed lower-than-reference LV EDVI measures before VB application, while only 4 patients did so following VB application. In addition, 7 patients showed lower-than-reference RV EF at baseline, while only 1 patient did so during VB application.
Comparing PE patient measurements with those of our healthy group, neither EDVI nor EF were different between the two groups at baseline, however, patients still had lower cardiac measurements on all baseline parameters compared with the healthy group.
As in previous studies on cardiac function at rest in PE patients, cardiac function is only mildly restricted, whereas reduced function is evident during exercise (15). Although the patients are in the lower limit of normal according to different sets of proposed reference values, the improvements brought by chest wall correction are markedly greater in PE patients when compared with the healthy controls.
Limitations and future perspectives
Several limitations should be mentioned. The analysis of the CMR images was performed in an un-blinded fashion, as blinding was technically impossible due to the degree of deformity in these patients (the Mean HI being 5.7±1.7) and the VB phase would have unavoidably become evident. The cohort was small, however the number of patients studied was comparable to other similar studies of cardiac function after PE repair. A larger validation cohort would be appropriate to confirm these observations. Variations of similar magnitude under different conditions have been reported before by other authors (6,13)
Cardiac Function variations, albeit statistically significant, were limited. It may be argued that such variations per se do not constitute a sufficient reason to recommend surgical correction. Conversely, most adult patients request PE correction because of psychological issues, rather than any cardiovascular impairment (34).
As previously reported (6,7,11) we could not find a correlation between the HI and cardiac function modifications following VB application. The HI did not fully account for the degree of cardiac compression, which can vary according to the shape of the chest and any displacement of the heart.
The depressed sternum was not sufficiently elevated in all patients (Figure 4), thus the effect of a corrected PE may be underestimated in this study.
Application of the VB is technically possible in patients while they are conducting strenuous exercise, hence this technique may be useful to observe physiological changes of sternal elevation under physical strain for example by transthoracic echocardiography. We are planning to perform the same CMRI analysis at 3 years follow-up, after bar removal. These results will reveal whether the VB device was a reliable predictor of cardiac improvement after permanent correction of PE.
Acknowledgments
The authors thank Dr. Michele Torre for his conceptual contribution to this study. Furthermore, the authors extend their gratitude to Dr. Ing. Eckart Klobe who supported this study by providing the Vacuum Bell devices. The authors are grateful for the continuous support to Prof. Gianluigi Condorelli and Prof. Isabella Barajon; finally, thanks to all the Humanitas University’ students who agreed to be part of the healthy control cohort.
Footnote
Conflicts of Interest: Dr. Hans Pilegaard is a consultant for Biomet. The other authors have no conflicts of interest to declare.
Ethical Statement: The authors are accountable for all aspects of the work in ensuring that questions related to the accuracy or integrity of any part of the work are appropriately investigated and resolved. The study was approved by the local ethics committee at Humanitas Research Hospital with registration number ICH-1347 and performed in accordance with the amended Declaration of Helsinki. All participants gave written informed consent with respect to study participation. Written informed consent was obtained from all the participants for publication of their individual details and images in this manuscript.
References
- Fokin AA, Steuerwald NM, Ahrens WA, Allen KE. Anatomical, histologic, and genetic characteristics of congenital chest wall deformities. Semin Thorac Cardiovasc Surg 2009;21:44-57. [Crossref] [PubMed]
- Kelly RE Jr, Cash TF, Shamberger RC, et al. Surgical repair of pectus excavatum markedly improves body image and perceived 8 ability for physical activity: multicenter study. Pediatrics 2008;122:1218-22. [Crossref] [PubMed]
- Lesbo M, Tang M, Nielsen HH, et al. Compromised cardiac function in exercising teenagers with pectus excavatum. Interact Cardiovasc Thorac Surg 2011;13:377-80. [Crossref] [PubMed]
- Malek MH, Fonkalsrud EW, Cooper CB. Ventilatory and cardiovascular responses to exercise in patients with pectus excavatum. Chest 2003;124:870-82. [Crossref] [PubMed]
- Tardy MM, Filaire M, Patoir A, et al. Exercise Cardiac Output Limitation in Pectus Excavatum. J Am Coll Cardiol 2015;66:976-7. [Crossref] [PubMed]
- Töpper A, Polleichtner S, Zagrosek A, et al. Impact of surgical correction of pectus excavatum on cardiac function: insights on the right ventricle. A cardiovascular magnetic resonance study. Interact Cardiovasc Thorac Surg 2016;22:38-46. [Crossref] [PubMed]
- Saleh RS, Finn JP, Fenchel M, et al. Cardiovascular magnetic resonance in patients with pectus excavatum compared with normal controls. J Cardiovasc Magn Reson 2010;12:73. [Crossref] [PubMed]
- Nuss D, Kelly RE Jr. Indications and technique of Nuss procedure for pectus excavatum. Thorac Surg Clin 2010;20:583-97. [Crossref] [PubMed]
- Koumbourlis AC. Pectus excavatum: pathophysiology and clinical characteristics. Paediatr Respir Rev 2009;10:3-6. [Crossref] [PubMed]
- Tang M, Nielsen HH, Lesbo M, et al. Improved cardiopulmonary exercise function after modified Nuss operation for pectus excavatum. Eur J Cardiothorac Surg 2012;41:1063-7. [Crossref] [PubMed]
- Maagaard M, Tang M, Ringgaard S, et al. Normalized cardiopulmonary exercise function in patients with pectus excavatum three years after operation. Ann Thorac Surg 2013;96:272-8. [Crossref] [PubMed]
- Neviere R, Benhamed L, Duva Pentiah A, et al. Pectus excavatum repair improves respiratory pump efficacy and cardiovascular function at exercise. J Thorac Cardiovasc Surg 2013;145:605-6. [Crossref] [PubMed]
- Krueger T, Chassot PG, Christodoulou M, et al. Cardiac function assessed by transesophageal echocardiography during pectus excavatum repair. Ann Thorac Surg 2010;89:240-3. [Crossref] [PubMed]
- Gürkan U, Aydemir B, Aksoy S, et al. Echocardiographic assessment of right ventricular function before and after surgery in patients with pectus excavatum and right ventricular compression. Thorac Cardiovasc Surg 2014;62:231-5. [PubMed]
- Maagaard M, Heiberg J. Improved cardiac function and exercise capacity following correction of pectus excavatum: a review of current literature. Ann Cardiothorac Surg 2016;5:485-92. [Crossref] [PubMed]
- Chao CJ, Jaroszewski DE, Kumar PN, et al. Surgical repair of pectus excavatum relieves right heart chamber compression and improves cardiac output in adult patients--an intraoperative transesophageal echocardiographic study. Am J Surg 2015;210:1118-24. [Crossref] [PubMed]
- Grothues F, Smith GC, Moon JC, et al. Comparison of interstudy reproducibility of cardiovascular magnetic resonance with two dimensional echocardiography in normal subjects and in patients with heart failure or left ventricular hypertrophy. Am J Cardiol 2002;90:29-34. [Crossref] [PubMed]
- Mooij CF, de Wit CJ, Graham DA, et al. Reproducibility of MRI measurements of right ventricular size and function in patients with normal and dilated ventricles. J Magn Reson Imaging 2008;28:67-73. [Crossref] [PubMed]
- Schier F, Bahr M, Klobe E. The vacuum chest wall lifter: an innovative, nonsurgical addition to the management of pectus excavatum. J Pediatr Surg 2005;40:496-500. [Crossref] [PubMed]
- Haecker FM, Sesia S. Vacuum bell therapy. Ann Cardiothorac Surg 2016;5:440-9. [Crossref] [PubMed]
- Lopez M, Patoir A, Costes F, et al. Preliminary study of efficacy of cup suction in the correction of typical pectus excavatum. J Pediatr Surg 2016;51:183-7. [Crossref] [PubMed]
- Haecker FM. The vacuum bell for conservative treatment of pectus excavatum: the Basle experience. Pediatr Surg Int 2011;27:623-7. [Crossref] [PubMed]
- Haecker FM, Mayr J. The vacuum bell for treatment of pectus excavatum: an alternative to surgical correction? Eur J Cardiothorac Surg 2006;29:557-61. [Crossref] [PubMed]
- Obermeyer RJ, Goretsky MJ. Chest wall deformities in pediatric surgery. Surg Clin North Am 2012;92:669-84. ix. [Crossref] [PubMed]
- Steinmann C, Krille S, Mueller A, et al. Pectus excavatum and pectus carinatum patients suffer from lower quality of life and impaired body image: a control group comparison of psychological characteristics prior to surgical correction. Eur J Cardiothorac Surg 2011;40:1138-45. [PubMed]
- Haller JA Jr, Kramer SS, Lietman SA. Use of CT scans in selection of patients for pectus excavatum surgery: a preliminary report. J Pediatr Surg 1987;22:904-6. [Crossref] [PubMed]
- Kramer CM, Barkhausen J, Flamm SD, et al. Society for Cardiovascular Magnetic Resonance Board of Trustees Task Force on Standardized P. Standardized cardiovascular magnetic resonance (CMR) protocols 2013 update. J Cardiovasc Magn Reson 2013;15:91. [Crossref] [PubMed]
- Schulz-Menger J, Bluemke DA, Bremerich J, et al. Standardized image interpretation and post processing in cardiovascular magnetic resonance: Society for Cardiovascular Magnetic Resonance (SCMR) board of trustees task force on standardized post processing. J Cardiovasc Magn Reson 2013;15:35. [Crossref] [PubMed]
- O'Keefe J, Byrne R, Montgomery M, et al. Longer term effects of closed repair of pectus excavatum on cardiopulmonary status. J Pediatr Surg 2013;48:1049-54. [Crossref] [PubMed]
- Hu TZ, Li Y, Liu WY, et al. Surgical treatment of pectus excavatum: 30 years 398 patients of experiences. J Pediatr Surg 2008;43:1270-4. [Crossref] [PubMed]
- Jaroszewski DE, Ewais MM, Chao CJ, et al. Success of minimally invasive pectus excavatum procedures (modified Nuss) in adult patients (30 years). Ann Thorac Surg 2016;102:993-1003. [Crossref] [PubMed]
- Bellenger NG, Davies LC, Francis JM, et al. Reduction in sample size for studies of remodelling in heart failure by the use of cardiovascular magnetic resonance. J Cardiovasc Magn Reson 2000;2:271-8. [Crossref] [PubMed]
- Aquaro GD, Camastra G, Monti L, et al. Reference values of cardiac volumes, dimensions, and new functional parameters by MR: A multicenter, multivendor study. J Magn Reson Imaging 2017;45:1055-67. [Crossref] [PubMed]
- Grothues F, Smith GC, Moon JC, et al. Comparison of interstudy reproducibility of cardiovascular magnetic resonance with two-dimensional echocardiography in normal subjects and in patients with heart failure or left ventricular hypertrophy. Am J Cardiol 2002;90:29-34. [Crossref] [PubMed]