Adrenergic regulation of the rapid component of delayed rectifier K+ currents in guinea pig cardiomyocytes
Introduction
Cardiac action potential repolarization is carried out by different potassium currents. The rapid component of the delayed rectifier potassium current (Ikr) is the most important component for phase 3 repolarization and is required to control orderly repolarization at the end of each cardiac action potential, particularly near the threshold potential of early afterdepolarizations that can trigger tachyarrhythmias (1). The human ether-a-go-go-related gene (hERG) encodes the alpha subunit of Ikr current (2-5). HERG channels are a primary target for the pharmacological management of cardiac arrhythmias using class III antiarrhythmic agents, such as dofetilide and amiodarone (6-8). Reduced hERG currents due to hERG mutations or excessive blockade of hERG channels by antiarrhythmic or non-antiarrhythmic drugs may lead to congenital or acquired long QT syndrome (9,10). The sympathetic nervous system modulates the Ikr current though both α1 and β-adrenoceptors (ARs) (11-14). Separate stimulation of α1-ARs or β-ARs decreased Ikr current and prolonged action potential. In recent years, considerable progress has been made toward a detailed understanding of these two signaling pathways. Bian and colleagues revealed that hERG potassium channels or the Ikr current of rabbit cardiomyocytes can be regulated by the α1-adrenoreceptor agonist phenylephrine (PE), which is consistent with our previous work in guinea pig (12,15). In an early report by Heath and Terrar, a concentration-independent increase in Ikr currents was observed at low concentrations of the β-adrenergic agonist isoprenaline (16), whereas Karle found activation of β-adrenoceptors elicits an inhibitory effect on Ikr or hERG via a cAMP/PKA-dependent pathway (17). Morever, at least three α1 adrenoceptor subtypes (α1A, α1B, and α1D) and three β adrenoceptor subtypes (β1, β2, and β3) have been pharmacologically identified, it is unclear which AR subtypes primarily regulate Ikr. In addition, the interaction between the α1 and β-adrenergic components during sympathetic regulation of the cardiac Ikr current were not examined, and their functional importance was not determined.
Methods
Cardiomyocyte preparation and whole-cell patch clamp
Guinea pigs (male, weighing 300±50 g) were purchased from Nanjing Medical University Institutional Animal Care. Single left ventricular myocytes were isolated from the heart of guinea pigs using the Langendorff apparatus as described previously (12). All experiments were performed in accordance with animal care protocols approved by the Nanjing Medical University Institutional Animal Care and Use Committee.
Cells were transferred to a recording chamber that was continuously perfused with bath solution. Whole-cell patch-clamp recordings were performed with an EPC-9 amplifier (HEKA, Germany) at 37±0.5 °C. Pipettes, filled with the pipette solution, had resistances of 3-6 MΩ. The flow rate of bath solution through the chamber was maintained at 2-3 mL/min.
Data analyses
Currents were acquired with Pulse + Pulsefit V8.53 and analyzed using SPSS 13.0 software. Statistical data are expressed as the mean ± standard error of the mean (S.E.M). Paired-sample t-tests were used to determine significant differences before and after PE or ISO intervention. One-way analyses of variance (ANOVA), with post-hoc comparisons using Newman-Keuls tests, were performed to compare differences among groups. Differences were considered significant if P<0.05.
Solutions and drug administration
For Ikr recordings, the solution was prepared as described by Wang et al. (12). The pipette solution contained 140 mM KCl, 1 mM CaCl2, 2 mM MgCl2, 10 mM HEPES, 11 mM EGTA, 5 mM Na2-ATP, and 5 mM creatine phosphate (disodium salt). The pH was adjusted to 7.4 using 8 M KOH. The bath solution contained 140 mM NaCl, 3.5 mM KCl, 1.5 mM CaCl2, 1.4 mM MgSO4, and 10 mM HEPES. The pH was adjusted to 7.4 using 10 M NaOH. Nifedipine (10 μM) was added to the bath solution to block calcium currents and 10 μM chromanol 293B was added to ablate the slow component of delayed rectifier potassium currents (IKs). Na2-ATP, EGTA, creatine phosphate, L-glutamic acid, HEPES, taurine, bovine serum albumin (BSA), nifedipine, chromanol 293B, dofetilide, PE, and ISO were purchased from Sigma (St. Louis, MO). Collagenase II was purchased from Worthington (Lakewood, NJ). All other reagents were obtained from Amresco.
For stock solutions, nifedipine and chromanol 293B were dissolved in dimethyl sulfoxide (DMSO) to a concentration of 10 mM. ISO was dissolved in distilled water to 10 mM, and PE and dofetilide were dissolved in distilled water to 1 mM. All stock solutions were stored at –20 °C, except nifedipine, which was stored at 4 °C.
Results (Figures 1-3)
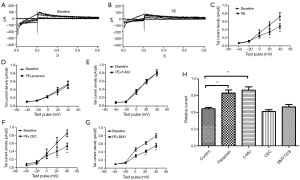
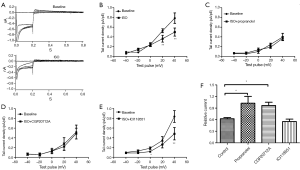
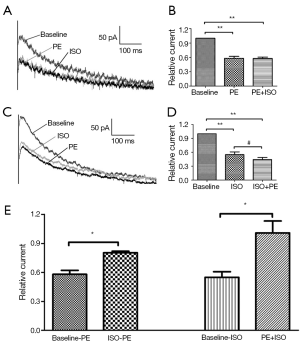
Ikr tail currents are inhibited by PE and α1A subtype mediates this effect
When cell chambers were perfused with a bath solution containing 0.1 μM of the specific α1-adrenereceptor agonist PE, the tail current amplitude significantly decreased (Figure 1A,B). The mean Ikr current density-voltage relations before and after PE are shown in Figure 1C. Ikr tail current density at +40 mV decreased to 0.66±0.02 upon the addition of 0.1 μM PE (first column of Figure 1H). When cells were pretreated with the non-selective α1-adrenergic antagonist prazosin (1 μM), PE does not decrease Ikr current (Figure 1D, second column of Figure 1H). To further evaluate the α1-adrenergic receptor (AR) subtype involved, selective α1-AR blockers, α1A-selective 5-methylurapidil (5-MU; 1μM), α1B-selective chloroethylclonidine (CEC; 10 μM), or α1D-selective BMY7378 (BMY; 1 nM) were applied together with PE (0.1 μM). When 5-MU, CEC, or BMY7378 was applied together with PE, the Ikr amplitudes decreased to 0.99±0.07, 0.62±0.03, and 0.70±0.04, respectively, indicating the α1A-receptor antagonist 5-MU prevented PE-induced suppression of Ikr, whereas the α1B and α1D-receptor blockers did not (Figure 1E-H). These results suggested that stimulation of the α1 adrenereceptor may reduce Ikr tail current, and that α1A, rather than the α1B or α1D receptor subtypes, is involved in this effect.
Ikr tail currents are inhibited by isoproterenol and β1 subtype mediates this effect
When cell chambers were perfused with a bath solution containing 10 μM of the non-specific β-adrenereceptor agonist isoproterenol (ISO), the tail current amplitude significantly decreased (Figure 2A). The mean Ikr current density-voltage relations before and after ISO are shown in Figure 2B. Ikr tail current density at +40 mV decreased to 0.62±0.03 upon the addition of ISO (first column of Figure 2F). Propranolol blocked this effect because when cells were pretreated with the non-specific β-adrenereceptor blocker propranolol (10 μM), ISO no longer decreased the Ikr tail current (Figure 2C, second column of Figure 2F). To evaluate the β-AR subtype involved, we added selective β-AR blockers along with ISO: β1-selective CGP-20712A (10 μM) and β2-selective ICI-118551 (10 μM). The β1-receptor blocker CGP completely prevented the ISO-induced reduction in Ikr (the Ikr tail current density at +40 mV decreased to 0.97±0.08), whereas the β2-receptor blocker ICI did not alter the action of ISO (the Ikr tail current density at +40 mV decreased to 0.55±0.06) (Figure 2D,E; first, third, and fourth columns of Figure 2F). These results suggested that stimulation of the β-adrenereceptor reduced Ikr tail current, and that the β1 rather than β2 receptor subtype is involved in the regulation of Ikr.
PE prevents the inhibitory effect of ISO on Ikr
We evaluated the effects of ISO in the presence of PE (0.1 μM). ISO (10 μM) was applied after the PE-induced changes stabilized (approximately 8-10 min after PE application). Changes in current amplitude are expressed relative to the amplitude before application of any drug (PE or ISO). Figure 3A shows a typical current trace of cells without drugs, then followed by PE and the last ISO. Figure 3B shows the relative current at test pulse +40 mV in each group. When cells were acutely stimulated with PE, the Ikr tail currents did not decrease with ISO addition (10 μM ISO after 0.1 μM PE). The relative current amplitudes were 1.01±0.12, which was significantly different from the ISO effect on Ikr without PE (third and fourth columns of Figure 3E). Thus, ISO does not reduce Ikr tail currents in cardiomyocytes pretreated with PE, which significantly differed from cardiomyocytes not acutely stimulated by PE. These results suggested that the PE prevents the current reduction effects of ISO on Ikr.
ISO attenuates the inhibitory effect of PE on Ikr
We also evaluated the effects of PE in the presence of ISO. PE (10 μM) was applied after the ISO-induced changes had stabilized. A typical current trace and the relative current at +40 mV test pulse in each group are shown in Figure 3C,D. When cardiomyocytes were acutely stimulated with ISO, the Ikr tail currents only decreased to 0.80±0.02 upon PE addition. This result significantly differed from cardiomyocytes that were not acutely stimulated by ISO (0.58±0.04, P<0.05) (first and second columns of Figure 3E). These data suggested that the inhibitory effects of PE on Ikr can be attenuated by ISO.
Discussion
In the present study, we report that the β1 and α1A-AR systems modulate Ikr. The current reduction effects of ISO can be prevented by preactivation of α1-adrenoceptors whereas the inhibitory effects of PE can be attenuated by preactivation of β-adrenoceptors. Signaling crosstalk between the α1A- and β1-adrenergic cascades might be involved in Ikr tail current regulation in guinea pig ventricular myocytes.
ARs bind and are activated by the endogenous catecholamine hormones epinephrine and norepinephrine (NE). Epinephrine is primarily produced and released into circulation from the adrenal gland. However, NE is synthesized and released by sympathetic nerve terminals in the peripheral nervous system and brain. In the heart, the two main ARs are the β-ARs, which comprise roughly 90% of total cardiac ARs, and α1-ARs, which account for approximately 10% (18). In this study, α1-adrenergic activation reduced Ikr in guinea pig ventricular cardiomyocytes, which are similar results to those reported by Thomas et al. (19) and Wang et al. (12). At least three α1-AR subtypes (α1A, α1B, α1D) have been described using molecular and pharmacological techniques (20,21). The ventricular α1-adrenoceptor densities did not significantly differ between guinea pig and human (22). A previous study found that different adrenoceptor subtypes have different effects on Ito (23) and Ica-L (24). We found that α1 adrenergic action on Ikr was via α1A. Currently, there are no data regarding the effects of α1B or α1D on Ikr. Moreover, we also found that in addition to α1, β-adrenergic activation also reduced Ikr, which is consistent with the study by Karle et al. (17). These results differ from the data of Heath and Terrar who reported that isoprenaline increased Ikr (16). This discrepancy could be related to ISO concentrations, different techniques inherent to whole-cell perforated patch clamp and switched electrode voltage clamp (SEVC), and/or different effects of β adreneoceptor subtypes on Ikr. The β1 and β2 adreneoceptor subtypes have different effects in regulating Ica-L (25) and cardiac function (26,27). Our study found that β-adrenoceptor action is mediated by the β1-adrenoceptor subtype rather than the β2-adrenoceptor subtype.
It is noteworthy that α1-AR activation-induced inhibition of Ikr tail current in the presence of β-AR activation differs significantly from α1-AR activation alone-induced inhibition of Ikr tail current. These data suggests that pre-activation of β-ARs suppresses the effect of α1-AR activation on Ikr tail current, and pre-activation of α1-ARs inhibits the effect of β-AR activation on Ikr tail current. Thus, pre-activation of one subtype adrenoceptor restrains the effect of another adrenoceptor subtype on Ikr tail current. That is, crosstalk exists in regulating Ikr current via acute adrenergic stimulation in physical circumstances. We propose that this is a protective regulatory mechanism against severe inhibition of Ikr tail current that occurs during excessive release of catecholamines in pathological circumstances.
Conclusions
Ikr in guinea pig cardiomyocyte can be regulated by both the α1 adrenergic system through the α1A subtype and the β adrenergic system through the β1 subtype. The absence of an inhibitory effect on Ikr by ISO was noted in cells pretreated with PE. The PE effect on Ikr was also attenuated in cells pretreated with ISO. Our results suggest a negative feedback loop intrinsic to norepinephrine stimulation in the heart and signaling crosstalk between α1A- and β1-adrenergic cascades, which might be involved in Ikr regulation in guinea pig ventricular myocytes. Signaling crosstalk may have a crucial role when plasma levels of both endogenous regulators are elevated.
Limitations
Our study found that crosstalk between α1A-ARs and β1-ARs in Ikr regulation exists. However, the precise adrenergic effects require further study to elucidate the molecular mechanisms that underlie the functional crosstalk as well as to identify putative intermediate proteins in the signal transduction pathways involved in modulation of Ikr current via adrenergic activation.
Acknowledgements
We thank Xiang-Jian Chen, Yang-Di, and Dao-Wu Wang for their assistance, and the Research Institute of Cardiovascular Disease of the First Affiliated Hospital of Nanjing Medical University.
Funding: This study was supported by the National Scientific Foundation of China (NSFC No. 81100123).
Disclosure: The authors declare no conflict of interest.
References
- Sanguinetti MC, Tristani-Firouzi M. hERG potassium channels and cardiac arrhythmia. Nature 2006;440:463-9. [PubMed]
- Keating MT, Sanguinetti MC. Molecular and cellular mechanisms of cardiac arrhythmias. Cell 2001;104:569-80. [PubMed]
- Sanguinetti MC, Jiang C, Curran ME, et al. A mechanistic link between an inherited and an acquired cardiac arrhythmia: HERG encodes the IKr potassium channel. Cell 1995;81:299-307. [PubMed]
- Tseng GN. I(Kr): the hERG channel. J Mol Cell Cardiol 2001;33:835-49. [PubMed]
- Charpentier F, Mérot J, Loussouarn G, et al. Delayed rectifier K(+) currents and cardiac repolarization. J Mol Cell Cardiol 2010;48:37-44. [PubMed]
- Spector PS, Curran ME, Keating MT, et al. Class III antiarrhythmic drugs block HERG, a human cardiac delayed rectifier K+ channel. Open-channel block by methanesulfonanilides. Circ Res 1996;78:499-503. [PubMed]
- Busch AE, Eigenberger B, Jurkiewicz NK, et al. Blockade of HERG channels by the class III antiarrhythmic azimilide: mode of action. Br J Pharmacol 1998;123:23-30. [PubMed]
- Kiehn J, Lacerda AE, Wible B, et al. Molecular physiology and pharmacology of HERG. Single-channel currents and block by dofetilide. Circulation 1996;94:2572-9. [PubMed]
- van Noord C, Sturkenboom MC, Straus SM, et al. Non-cardiovascular drugs that inhibit hERG-encoded potassium channels and risk of sudden cardiac death. Heart 2011;97:215-20. [PubMed]
- Vandenberg JI, Perry MD, Perrin MJ, et al. hERG K(+) channels: structure, function, and clinical significance. Physiol Rev 2012;92:1393-478. [PubMed]
- Thomas D, Karle CA, Kiehn J. The cardiac hERG/IKr potassium channel as pharmacological target: structure, function, regulation, and clinical applications. Curr Pharm Des 2006;12:2271-83. [PubMed]
- Wang S, Xu DJ, Cai JB, et al. Rapid component I(Kr) of cardiac delayed rectifier potassium currents in guinea-pig is inhibited by alpha(1)-adrenoreceptor activation via protein kinase A and protein kinase C-dependent pathways. Eur J Pharmacol 2009;608:1-6. [PubMed]
- Tutor AS, Delpón E, Caballero R, et al. Association of 14-3-3 proteins to beta1-adrenergic receptors modulates Kv11.1 K+ channel activity in recombinant systems. Mol Biol Cell 2006;17:4666-74. [PubMed]
- Wang H, Chen Y, Zhu H, et al. Increased response to β2-adrenoreceptor stimulation augments inhibition of IKr in heart failure ventricular myocytes. PLoS One 2012;7:e46186. [PubMed]
- Bian JS, Kagan A, McDonald TV. Molecular analysis of PIP2 regulation of HERG and IKr. Am J Physiol Heart Circ Physiol 2004;287:H2154-63. [PubMed]
- Heath BM, Terrar DA. Protein kinase C enhances the rapidly activating delayed rectifier potassium current, IKr, through a reduction in C-type inactivation in guinea-pig ventricular myocytes. J Physiol 2000;522:391-402. [PubMed]
- Karle CA, Zitron E, Zhang W, et al. Rapid component I(Kr) of the guinea-pig cardiac delayed rectifier K(+) current is inhibited by beta(1)-adrenoreceptor activation, via cAMP/protein kinase A-dependent pathways. Cardiovasc Res 2002;53:355-62. [PubMed]
- Brodde OE, Michel MC. Adrenergic and muscarinic receptors in the human heart. Pharmacol Rev 1999;51:651-90. [PubMed]
- Thomas D, Wu K, Wimmer AB, et al. Activation of cardiac human ether-a-go-go related gene potassium currents is regulated by alpha(1A)-adrenoceptors. J Mol Med (Berl) 2004;82:826-37. [PubMed]
- Price DT, Lefkowitz RJ, Caron MG, et al. Localization of mRNA for three distinct alpha 1-adrenergic receptor subtypes in human tissues: implications for human alpha-adrenergic physiology. Mol Pharmacol 1994;45:171-5. [PubMed]
- Jensen BC, Swigart PM, De Marco T, et al. {alpha}1-Adrenergic receptor subtypes in nonfailing and failing human myocardium. Circ Heart Fail 2009;2:654-63. [PubMed]
- Steinfath M, Chen YY, Lavický J, et al. Cardiac alpha 1-adrenoceptor densities in different mammalian species. Br J Pharmacol 1992;107:185-8. [PubMed]
- Martínez ML, Delgado C. Methoxamine inhibits transient outward potassium current through alpha1A-adrenoceptors in rat ventricular myocytes. J Cardiovasc Pharmacol 2000;35:212-8. [PubMed]
- O-Uchi J, Sasaki H, Morimoto S, et al. Interaction of alpha1-adrenoceptor subtypes with different G proteins induces opposite effects on cardiac L-type Ca2+ channel. Circ Res 2008;102:1378-88. [PubMed]
- Nagykaldi Z, Kem D, Lazzara R, et al. Conditioning of beta(1)-adrenoceptor effect via beta(2)-subtype on L-type Ca(2+) current in canine ventricular myocytes. Am J Physiol Heart Circ Physiol 2000;279:H1329-37. [PubMed]
- Devic E, Xiang Y, Gould D, et al. β-Adrenergic Receptor Subtype-Specific Signaling in Cardiac Myocytes from β1 and β2 Adrenoceptor Knockout Mice. Mol Pharmacol 2001;60:577-83. [PubMed]
- Ecker PM, Lin CC, Powers J, et al. Effect of targeted deletions of beta1- and beta2-adrenergic-receptor subtypes on heart rate variability. Am J Physiol Heart Circ Physiol 2006;290:H192-9. [PubMed]