Possible role of circulating tumor cells in early detection of lung cancer
Introduction
Lung cancer is the first malignancy worldwide for incidence and mortality, with an estimated 2.1 million diagnoses in 2018 (1). About 80% of lung cancers are classified as non-small cell lung cancer (NSCLC), 15% are small cell lung cancer (SCLC), and 5% are other histological variants (2).
In USA, from 2004 to 2015, only 1 out of 5 patients were diagnosed for lung cancer in stage I, whilst more than 3 out 5 patients were in stage III/IV of disease (3). Similar data were reported in several national and international reports (4,5), and treatment options and prognosis changed depending on stage at diagnosis. In fact, whereas surgery is the main treatment option of stage I disease with a 5-year survival rate close to 90%, in stage IV disease, chemotherapy, tailored therapy and immunotherapy can be used as single agents or in combination, but the 5-year survival rate does not reach 10% (6). Hence, beyond tobacco smoking prevention, a more comprehensive knowledge of lung cancer biology and its early detection represent the main steps in an ideal road map to improve patient prognosis (4,5).
Nowadays, liquid biopsy has emerged as a minimally-invasive tool to address the urgent need for real time monitoring, stratification, and personalized treatment of malignancies, including lung cancer. In principle, liquid biopsy from peripheral blood could provide the genetic landscape of all cancerous lesions at any time, detecting genomic alterations responsive to a specific therapy or associated with drug resistance. Moreover, it would allow a prognostic/predictive biomarker evaluation in patients for whom biopsies are inaccessible or difficult to repeat. Liquid biopsy now comprises a class of biomarkers, including circulating tumor cells (CTCs), cell-free circulating tumor DNA (ctDNA) and tumor-derived extracellular vesicles (tdEV). Among these, CTCs are the most suitable to identify malignancies more prone to give metastasis, only because, until proven otherwise, intact cells, and not their DNA, have the potential to cause metastasis.
Indeed, a high number of CTCs measured at any time during treatment has been associated, in several studies, with a shorter time to progression (7,8) in metastatic breast cancer, whilst a decreasing CTC number during treatment indicated therapeutic success (8,9). The prognostic value of CTCs in early breast cancer has then been proven (10). Similar findings were even reported in lung cancer (11,12) using the CellSearch® (CS) platform that first obtained the IVD mark from FDA, and to date, represents the “gold standard” (13). Data from several authors were gathered in meta-analyses (14-16) and confirmed CTCs as an independent prognostic indicator of progression-free survival (PFS) and overall survival (OS) in advanced NSCLC. Moreover, CTCs have been proposed as surrogate markers of distant metastasis in the early stages of lung cancer (17). Actually, based on the frequency distribution of CTCs detected by CS in 7.5 mL of blood, we could extrapolate that almost all cancer patients should have at least 1 CTC in peripheral blood, if enough blood volume could be analyzed (18). This observation impacts all clinical settings where there is a pressing need to disclose high-risk patients or anticipate a diagnosis of malignancy. From the clinical perspective, the exploitation of liquid biopsy in lung cancer promises to have a real effect on patient possibility of care and quality of life (19), in particular if combined with imaging examination (20).
However, we cannot ignore that the huge number of studies and techniques—often with different levels of validation (13)—that have used CTC enrichment, isolation and characterization, produced controversial results and limited transfer from benchtop to bedside (21). To understand this limited transfer, we reviewed the current scientific literature using the keywords ‘CTC and early lung cancer detection’, ‘CTC and lung cancer surgery’, ‘CTC and pulmonary vein’, ‘high-risk lung cancer patients and CTCs’ and ‘liquid biopsy and lung cancer screening’, in order to include all the references referring to CTC research, independently of the technologies used.
Thus, herein we will highlight different techniques, and different strategies (e.g., source of analysis sample or high-risk groups of patients) that could successfully contribute to implementing liquid biopsy in the clinical routine of non-metastatic lung cancer.
The technological race to detect CTCs in increasingly early stages of lung cancer: the finish line is still far away!
Due to sensitivity constraints in current CTC detection technologies, there is limited published data on CTC prevalence in early-stage lung cancer, or on the correlation of CTCs with disease progression and OS. Most of these studies have been conducted using different enrichment methods and identification techniques, making the results difficult to compare, especially since, for early-stage lung cancer, no reference CTC detection technology is yet available (22-34).
Table 1 shows a list of studies in which CTCs have been recovered from peripheral blood samples of lung cancer patients, even at early stages. Some authors, as expected, have highlighted a greater difficulty in finding positive CTC samples in early-stage patients than in later stage patients (30). This is not always the case, as Lou et al., using CytoploRareH circulating lung cancer cell kit (GenoSaber Biotech Co. Ltd., Shanghai, China) could detect, with the threshold of 8.5 CTC units, 8/10 (80%) of stage I–II, 6/9 (67%) of stage III, and 13/14 (93%) of stage IV initially diagnosed NSCLC (24). Nair et al. using the ‘high definition CTC’ (HD-CTC) technology (23,36) detected more than 2 HD-CTCs/mL in 61% of all TNM stage patients, and in 60% of stage I patients (25).
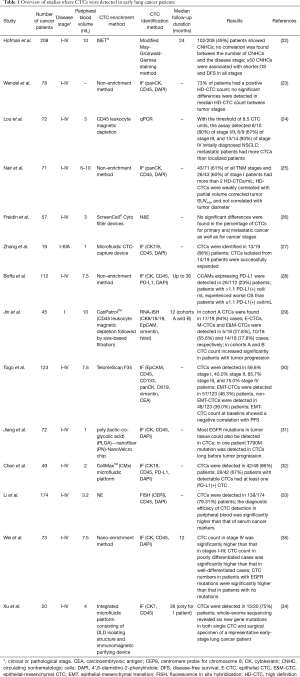
Full table
In some studies, the CTC count has been related to clinical stage, with a higher number of CTCs detected in more advanced stages (24,34,35); in others, no significant difference in the percentage of CTCs between primary and metastatic stage (22,23,26,29,30,33) was observed. Some authors related the number of CTCs to other clinic-pathological parameters; for example, Li et al. noted that, in those patients with adenocarcinomas, the CTC counts differed significantly with tumor depth. On the other hand, Jin et al. did not find any statistically significant correlation between the number of CTCs and tumor size; however, in the same group of patients, they noted that highly abundant mesenchymal CTCs were more prone to be in tumors >2 cm (29). Nair et al. observed that HD-CTCs were weakly correlated with tumor maximum standardized uptake value corrected for partial volume and not correlated with tumor diameter (25).
Some researchers compared the sensitivity of their CTC detection method with the sensitivity of common serum markers used for early detection or screening of cancer (30,33). Togo et al. noted that in early-stage (0–IA) lung adenocarcinoma patients, the TelomeScan F35-based detection sensitivity was 58.2%, whereas the sensitivity of carcinoembryonic antigen (CEA) detection (cut-off value of 5.0 ng/mL) was only 11.7%. However, by combining both assays, they achieved 65.1% sensitivity for the detection of early-stage NSCLC. Notably, 53.5% of early-stage patients tested negative in the CEA assay but positive for TelomeScan CTC detection (30). Li et al. observed that the sensitivity of CTC detection through negative enrichment-fluorescence in situ hybridization (NE-FISH) (37) and of a combination of tumor markers (CEA, CA 125, CYFRA 21-1, and SCC) for lung cancer diagnosis was 68.29% and 63.41%, respectively. However, combining all of those markers for the diagnosis of lung cancer, sensitivity was 82.93%. For patients with early−stage disease (I–II), sensitivity improved from 63.93% to 78.69% using this combination of all tested markers (33).
A few authors have also attempted to find an association between a specific level of CTCs and the survival of early lung cancer patients (22,27-30,35). In the work of Hofman et al., a level of 50 or more circulating nonhematologic cells (CNHCs) was associated with shorter OS and disease-free survival (DFS), independently of disease stage, and with a high-risk of recurrence and death in stage I–II resectable NSCLC (22). Jin et al. noted that a higher CTC level or change range could be found postoperatively in patients with tumor progression, as compared with patients with DFS (29). Togo et al. observed that patients testing positive for epithelial-mesenchymal transition CTCs (EMT-CTCs) at baseline had poor response to chemotherapy and decreased PFS in comparison with those testing negative (30).
Lastly, a limited number of researchers performed genetic analysis of CTCs isolated from patients with early lung cancer (27,31,35). Zhang et al. demonstrated that 9/15 (60%) patient samples examined for the TP53 gene by real-time polymerase chain reaction (RT-PCR) had mutations. Five patient samples had matched mutations in primary tumors and CTCs. In 3 patients, TP53 gene mutations were noted only in the primary tumor and not in the matching CTCs, whereas in 1 patient, the mutation was found only in the CTCs but not in the primary tumor. NGS further revealed additional matched mutations between primary tumor and CTCs of cancer-related genes and highlighted concordance for key genes involved in lung cancer progression (27). Jiang et al. observed that most EGFR mutations in tumor tissue could also be detected in CTCs. They concluded that, through the genetic analysis of the CTCs, it is possible to detect the onset of therapy resistance before tumor progression, as they have shown in the case of a patient treated with gefitinib, in which they detected the presence of the T790M mutation in CTCs long before tumor progression (31).
In conclusion, the use of CTCs in the early detection of lung cancer seems to be a promising, but insidious, route. While attempts are being made to increase the sensitivity of detection techniques, it is essential to understand whether the “CTCs” detected in patients with early-stage lung cancer could be clinically useful. Undoubtedly, the great improvements that are being made in the NGS field may reveal the characteristics of these cells and help us to understand their destiny, but a very long road still lies ahead.
Alternative sources for CTC recovery from early lung cancer patients
Surgical resection is considered the best option for treating patients with early-stage lung cancer; however, a proportion of patients develop recurrence, even after complete resection (38,39). The dissemination of CTCs is a relevant event in the process of metastasis and tumor recurrence (40). Intraoperative manipulation can cause the shedding of CTCs in the blood and accelerate metastases after surgery (41,42). Repeated CTC evaluation during the postoperative period might provide a useful tool for monitoring patients after curative resection (43,44). Several studies have attempted to identify the specific characteristics of CTCs in patients undergoing surgery for early-stage lung cancer. However, CTCs are very rare events (few CTCs among millions of blood cells), especially in early-stage lung cancer, thus requiring alternative strategies for their collection (45,46). In some cases, CTCs were recovered near their origin, i.e., from the pulmonary vein (PV) of the affected lobe, which can be accessed at the time of tumor resection (47-62).
The techniques used for the enrichment and the identification of CTCs from the PV are numerous, blood volumes are different, the number of patients is variable and it is not always possible to obtain significant data on the survival of patients due to too short follow-up periods (48,54,56,62). Despite these initial differences, it is clear that most studies converge towards similar conclusions (Table 2). Indeed, in most cases, a higher number of patients were positive for the presence of CTCs in the PV than in peripheral blood (48,49,52,54,55,57,61), and a higher number of CTCs were detected in the pulmonary vein (pvCTCs) than in peripheral blood (periCTCs) (49,53,55-57,59,61). Moreover, In some cases, a higher number of CTC clusters (55,57,61) or larger clusters (56) were noted in the PV than in peripheral blood.
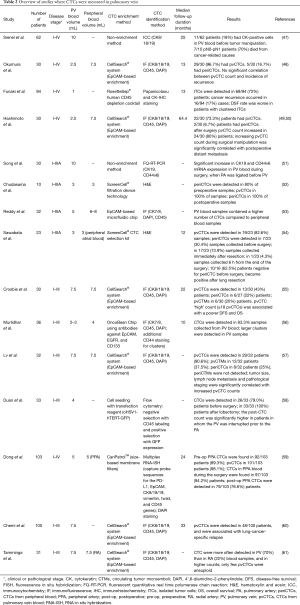
Full table
Some authors observed an increase in the number of samples positive for pvCTCs (58) and periCTCs (52,54) after surgery. Sawabata et al. noted that negative CTC patients before surgery became positive immediately after surgery (54). This increase in the number of CTCs has been attributed to the shedding of cells that can occur in concomitance with the manipulation of the tumor during surgery (47,49-52,54,58). Duan et al. observed that the increase in the number of CTCs occurred most frequently in patients where the PV was interrupted before the pulmonary artery (PA) (58); Song et al. noted an increase of CD44v6 and CK19 expression in patients where the PA was ligated before the PV (51). In other cases the sequence of vessel ligation did not influence CTC counts (49,50,61).
Data in the literature are contradictory about the correlation between the sequence ligation of the vessels before surgery and the increase in CTC number (48-50,58), and the data on the correlation between the number of CTCs detected in the PV and the survival of patients are also discordant (49-51,58,61). In the work of Sienel et al., 7/10 patients with CK+ cells in PV blood died from cancer-related causes (70%), while only 13/39 patients without occult cancer died from cancer-related causes (33%). The multivariate regression analysis demonstrated that cancer cell dissemination into PV blood was an independent significant prognosticator for reduced cancer-related survival in patients with pN0–pN1 lymph nodes. Okumura et al. did not find any significant difference in the incidence of recurrence or death according to pvCTC status; however, the median follow-up time from thoracotomy was only 13 months (48). In Hashimoto et al., the increasing pvCTC count (ΔpvCTC) during surgical manipulation was significantly correlated with postoperative distant metastasis. Significant shorter time to distant metastasis (TDM) was observed in high ΔpvCTC group (ΔpvCTC ≥119 cells/2.5 mL) than low ΔpvCTC group (ΔpvCTC <119 cells/2.5 mL). Neither DFS nor OS was significantly correlated with ΔpvCTC (50). Chudasama et al. did not observe any significant association between the development of distant metastatic deposits and increased levels of CTCs (52). Dong et al. noted that the PFS in the group with pvCTCs ≥16/5 mL was shorter than that in the group with pvCTCs <16/5 mL (11.1 vs. 21.2 months, respectively). The PFS in the group with peripheral arterial CTCs ≥3/5 mL was shorter than that in the group with peripheral arterial CTCs <3/5 mL (14.8 vs. 20.7 months, respectively). Crosbie et al. found an association between the pvCTC ‘high’ count (≥18 pvCTCs/7.5 mL blood) and DFS. The detection of any CTCs (≥1 CTC/7.5 mL blood) in peripheral blood was associated with reduced DFS and OS (55). When Chemi et al. applied the cut-off point of 18 pvCTCs in the TRACERx cohort, a significant association with poorer DFS was found, and this remained an independent predictor in multivariate analysis when adjusting for tumor stage. Moreover, a pvCTC-high status of ≥7 pvCTCs/7.5 mL blood showed a significant association with lung cancer relapse in Kaplan-Meier analysis and remained an independent predictor in multivariate analysis when adjusting for tumor stage (60).
Similarly, the presence of CTC clusters has been related to the survival of early lung cancer patients. Some authors observed that patients without clustered CTCs at the time of surgery had a higher relapse-free survival rate compared with those with clustered CTCs revealed during surgery (52,54). In accordance, Murlidhar et al. noted that patients with CTC clusters in the preoperative peripheral blood showed a trend toward lower PFS than those patients without clusters. Crosbie et al. identified circulating tumor microemboli (CTMs) in 6/7 high-pvCTC (85.7%) patients but not in the low-pvCTC group. The presence of CTMs was associated with a significant reduction in DFS and OS (55).
It is remarkable to note that the single cell whole genome sequencing analyses performed by Tamminga et al. unmasked the purely epithelial and non-malignant nature of several “CTCs” detected in the PV blood of early NSCLC patients. Indeed, only the CTCs isolated by FACS from 4/11 PV blood samples presented genomic aberrations. At this point, it should be asked whether the cells detected in PV during surgery or in peripheral blood immediately after surgery are in fact only non-malignant epithelial cells, released into circulation by surgical procedures, and destined to be rapidly eliminated due to their lower resistance to shear forces and mesenchymal environment (51,61). However, Lv et al. demonstrated the tumorigenicity of pvCTCs and pvCTMs by a xenograft experiment in which an adenocarcinoma developed subcutaneously in 1 out of 3 mice injected with pvCTCs and pvCTMs from 3 different patients.
We can conclude that other studies are needed to determine whether the cells detected in the PV blood of patients undergoing surgery for lung cancer are of tumor origin and whether they are related to a reduced PFS, DFS, TDM and OS. If, as Tamminga et al. sustained, these were largely non-malignant cells, it would be better to direct research towards the use of methods that allow the recovery of a greater number of CTCs from peripheral blood. Among these, methods for example examining a greater volume of blood, as in the case of the diagnostic leukapheresis (DLA) or of the GILUPI device, are discussed in the following section.
Alternative sample collection strategies for CTC recovery from early lung cancer patients
In lung cancer, as in several other cancers, the small number of CTCs per mL of peripheral blood is the major challenge. Although a simple solution may seem evident, it is becoming increasingly clear that it is necessary to increase the volumes of blood to be analyzed and/or treated. To date, two ways to overcome this obstacle are being studied: (I) in vivo CTC collection- and/or count-devices, and (II) apheresis-based procedures. The GILUPI CellCollector® (CC) allows the collection of CTCs in vivo, directly from blood. It is a functionalized medical wire covered with anti EpCAM antibodies which, when inserted in the cubital vein of a patient for 30 minutes, could trap CTCs from up to one liter and a half of blood. Through CC, studies reported an increased number of detected CTCs compared to standard methods (63-65). Recently, Didzdar and colleagues investigated the prognostic impact of CTCs rescued through CC in CRC (66), but contrary to other studies, they did not report any prognostic significance of CC-CTC. The authors estimated that recovering CTCs through CC is similar to extracting tumor cells from a blood volume ranging from 0.33–18 mL, a different volume compared to what was suggested in previous studies (volume range, 1–3 L). To date, CC use is feasible (67) and promising; no in-depth studies showing the real advantage of using this device in early lung cancer have been done.
To avoid the bottleneck due to the low number of CTCs, the CTCTrap consortium and Nick Stoecklein’s group used and validated DLA to enrich a higher number of CTCs. The CTCTrap efforts demonstrated that the procedure allowed improvement in the number of CTCs isolated in metastatic breast and metastatic prostate cancer and could be safely performed in different clinical sites (68).
The DLA product analyzed with CellSearch® demonstrated a 30-fold increase in median CTC numbers detected (69) and an increase in the number of CTC positive patients in M0 breast cancer patients (70). This high number of enriched CTCs was exploited for single cell molecular characterizations (71) and for CTC culture (70). Despite DLA enrichment, the success of cultured CTCs is limited to a few patients, such as in 2 out 8 patients when CTCs were cultured from fresh and cryopreserved DLA (70) and, like for other groups, culture success is generally reached in samples with more than 300 CTCs (72).
Statistically, it has been estimated that in order to find at least one CTC, total blood volume (TBV) must be analyzed, and that no less than 7.5 L of blood are necessary to detect at least 10 CTCs in almost every patient (18). Interestingly, before metastasis onset, the presence of about 9±6 CTC/L of blood has been mathematically predicted even in M0 breast cancer patients (73,74). Based on this mathematical prediction, even in low-CTC-number patients, analyzing up to 72 L of blood would result in finding at least 200 to almost 1,000 CTCs or more.
To date, no studies have yet been published using this approach in lung cancer. Tamminga and colleagues recently presented a study where they analyzed CTCs in the DLA product of NSCLC patients before and after treatment. Before treatment, CTCs were more often detected in DLA (32/55, 58%) compared to blood (pre: 18/55, 33%, P<0.01; post: 13/55, 24%, P<0.01). After treatment, the number of patients with CTCs were similar in DLA and peripheral blood (DLA: 14/34, 41%; pre: 9/34, 26%, P=0.18; post: 7/34, 21%, P=0.02) (75).
We look forward to more studies using DLA in early-stage lung cancer, improvements in its early detection and a better stratification of high-risk patients.
High-risk patient monitoring
The diagnosis of many early-stage lung cancers is delayed, due to the fact that most patients are often asymptomatic. Lung cancer screening aims to reduce lung cancer-related mortality by diagnosing the disease at the earliest stage possible in high-risk cohorts (76).
There is an urgent clinical need to develop biomarkers which can distinguish benign from malignant lesions and to identify biomarkers which can supplement computed tomography (CT) when screening for lung cancer, in order to reduce both the number of false positives and false negatives (77).
Despite many published studies on lung cancer screening biomarkers, none has yet been established in clinical practice. Lung cancer is up to five times more likely to occur in smokers with airflow obstruction than in those with normal lung function, and both chronic obstructive pulmonary disease (COPD) and lung cancer are associated with smoking (76,77).
COPD and lung cancer represent the leading causes of lung disease-related mortality worldwide. Among smokers, COPD is an important risk factor which might lead to lung cancer, and predates lung cancer in up to 70–80% of cases (78). The study by Mannino et al., revealed a distinct linear dose-response relationship between increasing COPD severity and risk of lung cancer (79).
A large retrospective study of patients with lung cancer showed that the coexistence of COPD predicted a significantly worse survival outcome (80-82).
In 2014, Hofman’s group compared the presence of CTC and CT-scan in COPD patients without clinically detectable lung cancer, with the aim to identify a biomarker for early lung cancer diagnosis (83). The study enrolled 168 COPD patients and 35 healthy non-smoker donors. Blood was collected at baseline, after 1 and 5 years. Using the ISET® method, they detected CTCs in 3% of COPD patients, where the presence of CTCs was correlated with the severity of COPD. At baseline, the CTC-positive COPD patients developed lung cancer; the CT scan preformed at the same time point of the CTC analysis confirmed the COPD diagnosis but failed to highlight lung nodules. The CT-scan program revealed the presence of lung nodules with a time delay of about 3.2 years. In these CTC-positive COPD patients, the early cancer diagnosis (IA lung cancer) allowed surgical resection of lung cancer and, in 16 months, the authors documented the absence of both CTCs and tumor recurrence. Recently, by exploiting the Carcinoma Cell Enrichment and Detection Kit (Miltenyi technology), a small study (only 17 COPD patients enrolled), demonstrated the presence of CK+ cells and CD44v6+ markers in the blood; both these markers are present in normal lung tissue but also in lung cancer (84). In COPD patients, due to the chronic inflammation and the relationship between vein and alveolar epithelium, it could be conceivable that lung cells could pass in blood. In our opinion, further study will clarify the origin of these CK+ CD44v6+ cells in the blood and their role as a biomarker (85).
Depending on which study we look at, about 25–50% of early-stage NSCLC patients relapse within 5 years after radical resection; for this reason, such patients are considered high-risk patients. To date, identifying relapsing high-risk patients is a goal we urgently need to meet. Because of this, several studies have analyzed CTCs: (I) their presence in venous or in arterial blood, (II) whether surgical manipulation could increase cancer cell dissemination, and (III) whether CTCs can be used as a prognostic biomarker in the early stages. In 2003, Sienel and colleagues analyzed a cohort of 62 patients with a complete resection of primary NSCLC and without signs of distant metastasis. They obtained PV blood collected at thoracotomy or prior to lung resection. CTC positive patients (18%) showed a reduced survival rate (47). In 2016, Crosbie et al. confirmed that, in the PV, CTCs are more frequent and associated with recurrence after surgical resection (55).
Few papers have analyzed CTCs before and after surgery and unfortunately, all these studies have small cohorts ranging from 30–60 patients. In all these studies, the authors documented a high number of CTCs before surgery compared to after surgery. The patients with persistent CTCs after surgery have a shorter DSF and a higher risk of recurrence.
Tamminga et al. recently analyzed in 31 patients the presence of CTCs in blood collected from both the radial artery (RA) and the PV. CTCs were found in 70% of PV compared to 22% of RA samples, regardless of the type of surgery patients underwent. They also documented CTC-clusters in PV samples only. CTCs isolated from 11 patients after scWGS analysis showed that copy number aberrations were present in few CTCs. The authors postulated that normal epithelial cells could be filtered in the central blood compartment from the PV (61).
All these studies highlighted how CTCs could be used as biomarkers in patients with a high-risk of lung cancer or with a high-risk of tumor recurrence in patients who underwent curative surgery.
CTCs to implement screening of NSCLC
The screening of high-risk patients represents the main course to increase the number of patients diagnosed at early stages of malignancies, significantly improving prognosis, as demonstrated by the long experience of breast, cervical and colorectal cancer. The approach to lung cancer does not vary (86), and according to the National Lung Screening Trial (NLST) (87), annual screening for lung cancer has been recommended for adults ages 55 to 80 years who have a 30 pack year smoking history and currently smoke or have quit within the past 15 years (88).
NLST demonstrated that low-dose helical computed tomography (LDCT) screening for lung cancer significantly reduced mortality rates—more than 20% vs. chest radiography—among high-risk subjects (88), even if uncertainty still exists regarding the harms of LDCT screening and the feasibility to extend this data to the general population (89,90).
However, a consensus exists that a more refined risk assessment would allow identification of high-risk individuals more likely to benefit from screening and determination of whether a screening detected nodule is or is not malignant.
To this purpose, some authors analyzed the feasibility to combine LDCT screening with CTC analysis.
In one study, He and colleagues randomly selected 32 cases out of the 88 ground-glass nodules (GGNs) patients, who were identified among 8,313 volunteers screened by LDCT (91). These 32 selected GGNs underwent a CellCollector procedure, and 6 EpCAM/CK-positive CTCs were identified from 5 patients. Notably, NGS data showed that three cancer-related genes contained mutations in 5 CTC-positive patients, including KIT, SMARCB1 and TP53 genes. Moreover, in 4 patients, 16 mutation genes existed. Therefore, the authors concluded that LDCT combined with CTC analysis in high-risk pulmonary nodule patients was a promising way to screen early-stage lung cancer.
More recently, Manjunath and colleagues (92) compared CTCs and CTC clusters in 29 treatment-naïve NSCLC patients, 31 high-risk LDCT screened patients—with or without lung nodules (lung-RADS 2 and 1, respectively)—and 20 never-smoking healthy controls. The 3 groups differed according to these parameters, since both single CTCs (ranged, 11–40) and CTC clusters were detectable in NSCLC, whilst significantly lower number of single CTCs (ranged, 0–8) and no CTC cluster were reported in LDCT screened patients; as expected the healthy controls resulted negative for both parameters. The findings of this pilot study are promising, even if further confirmation in prospective trials, ad hoc designed, is warranted.
Conclusions
The analysis of CTCs represents a useful tool to monitor the evolution of malignancies, especially when tumor biopsy is uncomfortable for the patient or totally impracticable, as it often is in lung cancer.
Moreover, much evidence has been already reported that the CTC analysis has the potential to anticipate the diagnosis of progressing disease in pulmonary high-risk patients or to discriminate the malignant nature of a screened pulmonary nodule. However, more studies are needed to definitively prove the value of CTCs in the early detection of lung cancer, alone or in combination with screening plans, in order to reduce the risk of over-diagnosis and over-treatment, usually determined by the LDCT screening (93).
Acknowledgments
The authors thank Christina Drace (IOV-IRCCS, Padua, Italy) for English editing.
Funding: The concepts developed in this review derived from the work performed at the CTC-laboratory of IOV-IRCCS, Padua, Italy, and partially funded by Innovative Medicine Initiative Joint Undertaking (115749) CANCER-ID; Intramural 5X1000 SINERGIA, IOV-IRCCS, “CTC/cfDNA” (PI: Rita Zamarchi); Intramural 5X1000 SINERGIA, IOV-IRCCS, “NSCLC: Mutational and metabolic characteristics of Circulating Tumor Cells (CTC)” (PI: Elisabetta Rossi).
Footnote
Provenance and Peer Review: This article was commissioned by the Guest Editor (Alfredo Tartarone) for the Series “Improving Outcomes in Lung Cancer Through Early Diagnosis and Smoking Cessation” published in Journal of Thoracic Disease. The article was sent for external peer review organized by the Guest Editor and the editorial office.
Conflicts of Interest: All authors have completed the ICMJE uniform disclosure form (available at
Ethical Statement: The authors are accountable for all aspects of the work in ensuring that questions related to the accuracy or integrity of any part of the work are appropriately investigated and resolved.
Open Access Statement: This is an Open Access article distributed in accordance with the Creative Commons Attribution-NonCommercial-NoDerivs 4.0 International License (CC BY-NC-ND 4.0), which permits the non-commercial replication and distribution of the article with the strict proviso that no changes or edits are made and the original work is properly cited (including links to both the formal publication through the relevant DOI and the license). See:
References
- Bray F, Ferlay J, Soerjomataram I, et al. Global cancer statistics 2018: GLOBOCAN estimates of incidence and mortality worldwide for 36 cancers in 185 countries. CA Cancer J Clin 2018;68:394-424. [Crossref] [PubMed]
- Hamilton G, Rath B. Detection of circulating tumor cells in non-small cell lung cancer. J Thorac Dis 2016;8:1024-8. [Crossref] [PubMed]
- Cancer Trends Progress Report. Online Summary of Trends in US Cancer Control Measures. Available online: https://progressreport.cancer.gov/
- Blandin Knight S, Crosbie PA, Balata H, et al. Progress and prospects of early detection in lung cancer. Open Biol 2017;7:170070. [Crossref] [PubMed]
- Kapeleris J, Kulasinghe A, Warkiani ME, et al. The prognostic role of circulating tumor cells (CTCs) in lung cancer. Front Oncol 2018;8:311. [Crossref] [PubMed]
- Detterbeck FC, Boffa DJ, Kim AW, et al. The eighth edition lung cancer stage classification. Chest 2017;151:193-203.
- Cristofanilli M, Budd GT, Ellis MJ, et al. Circulating tumor cells, disease progression, and survival in metastatic breast cancer. N Engl J Med 2004;351:781-91. [Crossref] [PubMed]
- Hayes DF, Cristofanilli M, Budd GT, et al. Circulating tumor cells at each follow-up time point during therapy of metastatic breast cancer patients predict progression-free and overall survival. Clin Cancer Res 2006;12:4218-24. [Crossref] [PubMed]
- Bidard FC, Peeters DJ, Fehm T, et al. Clinical validity of circulating tumour cells in patients with metastatic breast cancer: a pooled analysis of individual patient data. Lancet Oncol 2014;15:406-14. [Crossref] [PubMed]
- Janni WJ, Rack B, Terstappen LWMM, et al. Pooled analysis of the prognostic relevance of circulating tumor cells in primary breast cancer. Clin Cancer Res 2016;22:2583-93. [Crossref] [PubMed]
- Allard WJ, Matera J, Miller MC, et al. Tumor cells circulate in the peripheral blood of all major carcinomas but not in healthy subjects or patients with nonmalignant diseases. Clin Cancer Res 2004;10:6897-904. [Crossref] [PubMed]
- Krebs MG, Sloane R, Priest L, et al. Evaluation and prognostic significance of circulating tumor cells in patients with non-small-cell lung cancer. J Clin Oncol 2011;29:1556-63. [Crossref] [PubMed]
- Parkinson DR, Dracopoli N, Petty BG, et al. Considerations in the development of circulating tumor cell technology for clinical use. J Transl Med 2012;10:138. [Crossref] [PubMed]
- Ma XL, Xiao ZL, Liu L, et al. Meta-analysis of circulating tumor cells as a prognostic marker in lung cancer. Asian Pac J Cancer Prev 2012;13:1137-44. [Crossref] [PubMed]
- Wang J, Wang K, Xu J, et al. Prognostic significance of circulating tumor cells in non-small-cell lung cancer patients: a meta-analysis. PLoS One 2013;8:e78070. [Crossref] [PubMed]
- Lindsay CR, Blackhall FH, Carmel A, et al. EPAC-lung: pooled analysis of circulating tumour cells in advanced non-small cell lung cancer. Eur J Cancer 2019;117:60-8. [Crossref] [PubMed]
- Tanaka F, Yoneda K, Kondo N, et al. Circulating tumor cell as a diagnostic marker in primary lung cancer. Clin Cancer Res 2009;15:6980-6. [Crossref] [PubMed]
- Coumans FA, Ligthart ST, Uhr JW, et al. Challenges in the enumeration and phenotyping of CTC. Clin Cancer Res 2012;18:5711-8. [Crossref] [PubMed]
- Tognela A, Spring KJ, Becker T, et al. Predictive and prognostic value of circulating tumor cell detection in lung cancer: a clinician’s perspective. Crit Rev Oncol Hematol 2015;93:90-102. [Crossref] [PubMed]
- Wu Z, Yang Z, Dai Y, et al. Update on liquid biopsy in clinical management of non-small cell lung cancer. Onco Targets Ther 2019;12:5097-109. [Crossref] [PubMed]
- Hofman V, Heeke S, Marquette CH, et al. Circulating tumor cell detection in lung cancer: but to what end? Cancers (Basel) 2019. [Crossref] [PubMed]
- Hofman V, Bonnetaud C, Ilie MI, et al. Preoperative circulating tumor cell detection using the isolation by size of epithelial tumor cell method for patients with lung cancer is a new prognostic biomarker. Clin Cancer Res 2011;17:827-35. [Crossref] [PubMed]
- Wendel M, Bazhenova L, Boshuizen R, et al. Fluid biopsy for circulating tumor cell identification in patients with early-and late-stage non-small cell lung cancer: a glimpse into lung cancer biology. Phys Biol 2012;9:016005. [Crossref] [PubMed]
- Lou J, Ben S, Yang G, et al. Quantification of rare circulating tumor cells in non-small cell lung cancer by ligand-targeted PCR. PLoS One 2013;8:e80458. [Crossref] [PubMed]
- Nair VS, Keu KV, Luttgen MS, et al. An observational study of circulating tumor cells and (18)F-FDG PET uptake in patients with treatment-naive non-small cell lung cancer. PLoS One 2013;8:e67733. [Crossref] [PubMed]
- Freidin MB, Tay A, Freydina DV, et al. An assessment of diagnostic performance of a filter-based antibody-independent peripheral blood circulating tumour cell capture paired with cytomorphologic criteria for the diagnosis of cancer. Lung Cancer 2014;85:182-5. [Crossref] [PubMed]
- Zhang Z, Shiratsuchi H, Lin J, et al. Expansion of CTCs from early stage lung cancer patients using a microfluidic co-culture model. Oncotarget 2014;5:12383-97. [Crossref] [PubMed]
- Boffa DJ, Graf RP, Salazar MC, et al. Cellular expression of PD-L1 in the peripheral blood of lung cancer patients is associated with worse survival. Cancer Epidemiol Biomarkers Prev 2017;26:1139-45. [Crossref] [PubMed]
- Jin XR, Zhu LY, Qian K, et al. Circulating tumor cells in early stage lung adenocarcinoma: a case series report and literature review. Oncotarget 2017;8:23130-41. [Crossref] [PubMed]
- Togo S, Katagiri N, Namba Y, et al. Sensitive detection of viable circulating tumor cells using a novel conditionally telomerase-selective replicating adenovirus in non-small cell lung cancer patients. Oncotarget 2017;8:34884-95. [Crossref] [PubMed]
- Jiang W, Wang H, Cui Y, et al. Polymer nanofiber-based microchips for EGFR mutation analysis of circulating tumor cells in lung adenocarcinoma. Int J Nanomedicine 2018;13:1633-42. [Crossref] [PubMed]
- Chen YL, Huang WC, Lin FM, et al. Novel circulating tumor cell-based blood test for the assessment of PD-L1 protein expression in treatment-naïve, newly diagnosed patients with non-small cell lung cancer. Cancer Immunol Immunother 2019;68:1087-94. [Crossref] [PubMed]
- Li Y, Tian X, Gao L, et al. Clinical significance of circulating tumor cells and tumor markers in the diagnosis of lung cancer. Cancer Med 2019;8:3782-92. [PubMed]
- Xu M, Zhao H, Chen J, et al. An integrated microfluidic chip and its clinical application for circulating tumor cell isolation and single-cell analysis. Cytometry A 2020;97:46-53. [Crossref] [PubMed]
- Wei T, Zhu D, Yang Y, et al. The application of nano-enrichment in CTC detection and the clinical significance of CTCs in non-small cell lung cancer (NSCLC) treatment. PLoS One 2019;14:e0219129. [Crossref] [PubMed]
- Marrinucci D, Bethel K, Kolatkar A, et al. Fluid biopsy in patients with metastatic prostate, pancreatic and breast cancers. Phys Biol 2012;9:016003. [Crossref] [PubMed]
- Li Y, Ma G, Zhao P, et al. Improvement of sensitive and specific detection of circulating tumor cells using negative enrichment and immunostaining-FISH. Clin Chim Acta 2018;485:95-102. [Crossref] [PubMed]
- Taylor MD, Nagji AS, Bhamidipati CM, et al. Tumor recurrence after complete resection for non-small cell lung cancer. Ann Thorac Surg 2012;93:1813-20; discussion 1820-1.
- Uramoto H, Tanaka F. Recurrence after surgery in patients with NSCLC. Transl Lung Cancer Res 2014;3:242-9. [PubMed]
- Pantel K, Brakenhoff RH, Brandt B. Detection, clinical relevance and specific biological properties of disseminating tumour cells. Nat Rev Cancer 2008;8:329-40. [Crossref] [PubMed]
- Yamashita JI, Kurusu Y, Fujino N, et al. Detection of circulating tumor cells in patients with non-small cell lung cancer undergoing lobectomy by video-assisted thoracic surgery: a potential hazard for intraoperative hematogenous tumor cell dissemination. J Thorac Cardiovasc Surg 2000;119:899-905. [Crossref] [PubMed]
- Dong Q, Huang J, Zhou Y, et al. Hematogenous dissemination of lung cancer cells during surgery: quantitative detection by flow cytometry and prognostic significance. Lung Cancer 2002;37:293-301. [Crossref] [PubMed]
- Sawabata N, Okumura M, Utsumi T, et al. Circulating tumor cells in peripheral blood caused by surgical manipulation of non-small-cell lung cancer: pilot study using an immunocytology method. Gen Thorac Cardiovasc Surg 2007;55:189-92. [Crossref] [PubMed]
- Kuwata T, Yoneda K, Kobayashi K, et al. Circulating tumor cells as an indicator of postoperative lung cancer: a case report. Am J Case Rep 2016;17:663-5. [Crossref] [PubMed]
- Nagrath S, Sequist LV, Maheswaran S, et al. Isolation of rare circulating tumour cells in cancer patients by microchip technology. Nature 2007;450:1235-9. [Crossref] [PubMed]
- Murlidhar V, Zeinali M, Grabauskiene S, et al. A radial flow microfluidic device for ultra-high-throughput affinity-based isolation of circulating tumor cells. Small 2014;10:4895-904. [Crossref] [PubMed]
- Sienel W, Seen-Hibler R, Mutschler W, et al. Tumour cells in the tumour draining vein of patients with non-small cell lung cancer: detection rate and clinical significance. Eur J Cardiothorac Surg 2003;23:451-6. [Crossref] [PubMed]
- Okumura Y, Tanaka F, Yoneda K, et al. Circulating tumor cells in pulmonary venous blood of primary lung cancer patients. Ann Thorac Surg 2009;87:1669-75. [Crossref] [PubMed]
- Hashimoto M, Tanaka F, Yoneda K, et al. Significant increase in circulating tumour cells in pulmonary venous blood during surgical manipulation in patients with primary lung cancer. Interact Cardiovasc Thorac Surg 2014;18:775-83. [Crossref] [PubMed]
- Hashimoto M, Tanaka F, Yoneda K, et al. Positive correlation between postoperative tumor recurrence and changes in circulating tumor cell counts in pulmonary venous blood (pvCTC) during surgical manipulation in non-small cell lung cancer. J Thorac Dis 2018;10:298-306. [Crossref] [PubMed]
- Song PP, Zhang W, Zhang B, et al. Effects of different sequences of pulmonary artery and vein ligations during pulmonary lobectomy on blood micrometastasis of non-small cell lung cancer. Oncol Lett 2013;5:463-8. [Crossref] [PubMed]
- Chudasama D, Burnside N, Beeson J, et al. Perioperative detection of circulating tumour cells in patients with lung cancer. Oncol Lett 2017;14:1281-6. [Crossref] [PubMed]
- Reddy RM, Murlidhar V, Zhao L, et al. Pulmonary venous blood sampling significantly increases the yield of circulating tumor cells in early-stage lung cancer. J Thorac Cardiovasc Surg 2016;151:852-8. [Crossref] [PubMed]
- Sawabata N, Funaki S, Hyakutake T, et al. Perioperative circulating tumor cells in surgical patients with non-small cell lung cancer: does surgical manipulation dislodge cancer cells thus allowing them to pass into the peripheral blood? Surg Today 2016;46:1402-9. [Crossref] [PubMed]
- Crosbie PA, Shah R, Krysiak P, et al. Circulating tumor cells detected in the tumor-draining pulmonary vein are associated with disease recurrence after surgical resection of NSCLC. J Thorac Oncol 2016;11:1793-7. [Crossref] [PubMed]
- Murlidhar V, Reddy RM, Fouladdel S, et al. Poor prognosis indicated by venous circulating tumor cell clusters in early-stage lung cancers. Cancer Res 2017;77:5194-206. [Crossref] [PubMed]
- Lv C, Zhao B, Wang L, et al. Detection of circulating tumor cells in pulmonary venous blood for resectable non-small cell lung cancer. Oncol Lett 2018;15:1103-12. [PubMed]
- Duan X, Zhu Y, Cui Y, et al. Circulating tumor cells in the pulmonary vein increase significantly after lobectomy: a prospective observational study. Thorac Cancer 2019;10:163-9. [Crossref] [PubMed]
- Dong J, Zhu D, Tang X, et al. Circulating tumor cells in pulmonary vein and peripheral arterial provide a metric for PD-L1 diagnosis and prognosis of patients with non-small cell lung cancer. PLoS One 2019;14:e0220306. [Crossref] [PubMed]
- Chemi F, Rothwell DG, McGranahan N, et al. Pulmonary venous circulating tumor cell dissemination before tumor resection and disease relapse. Nat Med 2019;25:1534-9. [Crossref] [PubMed]
- Tamminga M, de Wit S, van de Wauwer C, et al. Release of circulating tumor cells during surgery for non-small cell lung cancer: are they what they appear to be? Clin Cancer Res 2019. [Epub ahead of print].
- Funaki S, Sawabata N, Nakagiri T, et al. Novel approach for detection of isolated tumor cells in pulmonary vein using negative selection method: morphological classification and clinical implications. Eur J Cardiothorac Surg 2011;40:322-7. [PubMed]
- Gorges TM, Penkalla N, Schalk T, et al. Enumeration and molecular characterization of tumor cells in lung cancer patients using a novel in vivo device for capturing circulating tumor cells. Clin Cancer Res 2016;22:2197-206. [Crossref] [PubMed]
- Kuske A, Gorges TM, Tennstedt P, et al. Improved detection of circulating tumor cells in non-metastatic high-risk prostate cancer patients. Sci Rep 2016;6:39736. [Crossref] [PubMed]
- Mandair D, Vesely C, Ensell L, et al. A comparison of CellCollector with CellSearch in patients with neuroendocrine tumours. Endocr Relat Cancer 2016;23:L29-32. [Crossref] [PubMed]
- Dizdar L, Fluegen G, van Dalum G, et al. Detection of circulating tumor cells in colorectal cancer patients using the GILUPI CellCollector: results from a prospective, single-center study. Mol Oncol 2019;13:1548-58. [Crossref] [PubMed]
- Gallerani G, Cocchi C, Bocchini M, et al. Characterization of tumor cells using a medical wire for capturing circulating tumor cells: a 3D approach based on immunofluorescence and DNA FISH. J Vis Exp 2017. [Crossref] [PubMed]
- Andree KC, Mentink A, Zeune LL, et al. Toward a real liquid biopsy in metastatic breast and prostate cancer: diagnostic LeukApheresis increases CTC yields in a European prospective multicenter study (CTCTrap). Int J Cancer 2018;143:2584-91. [Crossref] [PubMed]
- Fischer JC, Niederacher D, Topp SA, et al. Diagnostic leukapheresis enables reliable detection of circulating tumor cells of nonmetastatic cancer patients. Proc Natl Acad Sci U S A 2013;110:16580-5. [Crossref] [PubMed]
- Franken A, Driemel C, Behrens B, et al. Label-free enrichment and molecular characterization of viable circulating tumor cells from diagnostic leukapheresis products. Clin Chem 2019;65:549-58. [Crossref] [PubMed]
- Lambros MB, Seed G, Sumanasuriya S, et al. Single-cell analyses of prostate cancer liquid biopsies acquired by apheresis. Clin Cancer Res 2018;24:5635-44. [Crossref] [PubMed]
- Cayrefourcq L, Mazard T, Joosse S, et al. Establishment and characterization of a cell line from human circulating colon cancer cells. Cancer Res 2015;75:892-901. [Crossref] [PubMed]
- Coumans FAW, Siesling S, Terstappen LWMM. Detection of cancer before distant metastasis. BMC Cancer 2013;13:283. [Crossref] [PubMed]
- Stoecklein NH, Fischer JC, Niederacher D, et al. Challenges for CTC-based liquid biopsies: low CTC frequency and diagnostic leukapheresis as a potential solution. Expert Rev Mol Diagn 2016;16:147-64. [Crossref] [PubMed]
- Tamminga M, Andree KC, Van den Bos H, et al. Diagnostic leukapheresis increases circulating tumor cell yield in non-small cell lung cancer patients, which correspond with response and survival. Rochester: Social Science Research Network, 2019.
- Parris BA, O’Farrell HE, Fong KM, et al. Chronic obstructive pulmonary disease (COPD) and lung cancer: common pathways for pathogenesis. J Thorac Dis 2019;11:S2155-72. [Crossref] [PubMed]
- Berg J, Halvorsen AR, Bengtson MB, et al. Levels and prognostic impact of circulating markers of inflammation, endothelial activation and extracellular matrix remodelling in patients with lung cancer and chronic obstructive pulmonary disease. BMC Cancer 2018;18:739. [Crossref] [PubMed]
- Young RP, Hopkins RJ. How the genetics of lung cancer may overlap with COPD. Respirology 2011;16:1047-55. [Crossref] [PubMed]
- Mannino DM, Aguayo SM, Petty TL, et al. Low lung function and incident lung cancer in the United States: data From the First National Health and Nutrition Examination Survey follow-up. Arch Intern Med 2003;163:1475-80. [Crossref] [PubMed]
- Young RP, Hopkins RJ, Christmas T, et al. COPD prevalence is increased in lung cancer, independent of age, sex and smoking history. Eur Respir J 2009;34:380-6. [Crossref] [PubMed]
- Gonzalez J, Marín M, Sánchez-Salcedo P, et al. Lung cancer screening in patients with chronic obstructive pulmonary disease. Ann Transl Med 2016;4:160. [Crossref] [PubMed]
- Wang W, Dou S, Dong W, et al. Impact of COPD on prognosis of lung cancer: from a perspective on disease heterogeneity. Int J Chron Obstruct Pulmon Dis 2018;13:3767-76. [Crossref] [PubMed]
- Ilie M, Hofman V, Long-Mira E, et al. "Sentinel" circulating tumor cells allow early diagnosis of lung cancer in patients with chronic obstructive pulmonary disease. PLoS One 2014;9:e111597. [Crossref] [PubMed]
- Afify AM, Tate S, Durbin-Johnson B, et al. Expression of CD44s and CD44v6 in lung cancer and their correlation with prognostic factors. Int J Biol Markers 2011;26:50-7. [Crossref] [PubMed]
- Romero-Palacios PJ, Alcázar-Navarrete B, Díaz Mochón JJ, et al. Liquid biopsy beyond of cancer: Circulating pulmonary cells as biomarkers of COPD aggressivity. Crit Rev Oncol Hematol 2019;136:31-6. [Crossref] [PubMed]
- Armstrong K, Kim JJ, Halm EA, et al. Using lessons from breast, cervical, and colorectal cancer screening to inform the development of lung cancer screening programs. Cancer 2016;122:1338-42. [Crossref] [PubMed]
- Tanoue LT, Tanner NT, Gould MK, et al. Lung cancer screening. Am J Respir Crit Care Med 2015;191:19-33. [Crossref] [PubMed]
- National Lung Screening Trial Research Team, Aberle DR, Adams AM, et al. Reduced lung-cancer mortality with low-dose computed tomographic screening. N Engl J Med 2011;365:395-409. [Crossref] [PubMed]
- Bach PB, Mirkin JN, Oliver TK, et al. Benefits and harms of CT screening for lung cancer: a systematic review. JAMA 2012;307:2418-29. [Crossref] [PubMed]
- Nanavaty P, Alvarez MS, Alberts WM. Lung cancer screening: advantages, controversies, and applications. Cancer Control 2014;21:9-14. [Crossref] [PubMed]
- He Y, Shi J, Shi G, et al. Using the new CellCollector to capture circulating tumor cells from blood in different groups of pulmonary disease: a cohort study. Sci Rep 2017;7:9542. [Crossref] [PubMed]
- Manjunath Y, Upparahalli SV, Suvilesh KN, et al. Circulating tumor cell clusters are a potential biomarker for detection of non-small cell lung cancer. Lung Cancer 2019;134:147-50. [Crossref] [PubMed]
- Srivastava S, Koay EJ, Borowsky AD, et al. Cancer overdiagnosis: a biological challenge and clinical dilemma. Nat Rev Cancer 2019;19:349-58. [Crossref] [PubMed]