What can individuals do to reduce personal health risks from air pollution?
Introduction
Air pollution is a serious global public health problem that is managed most effectively by collective (societal) action to control emissions of both primary air pollutants and precursors that react to form secondary air pollutants. Unfortunately, in many areas of the world, concentrations of ambient air pollutants currently exceed levels believed to substantially increase risks of acute and chronic adverse human health effects. Affected areas include many of the urban communities where a majority of the world’s population now lives and works (1). While waiting for governments to act, or controls to be implemented, are there personal actions that can be taken by individuals to effectively reduce the risks of adverse health effects from air pollution?
As reviewed elsewhere in this issue, scientific studies provide strong evidence for a growing number of adverse health effects of exposure to air pollutants. Given the evidence of harm, the benefits of acting to reduce personal exposure to air pollution may seem self-evident. Indeed, studies have shown that reductions in exposure at the population level, either due to natural experiments or long-term trends, improve health outcomes (2,3). However, personal-level interventions may have varying degrees of effectiveness for reducing exposure and/or reducing risk, and there has been a dearth of research on actual health outcomes after personal interventions. This is due, at least in part, to difficulties in evaluating the effects of personal interventions on air pollution-attributable health events, which, despite their public health significance, have relatively low frequencies across broad populations. Also, personal actions to reduce exposure to air pollution are best viewed in the context of total risk, because such actions have the potential to cause unintended health effects by altering other risk factors. Interventions aimed at reducing individual susceptibility, or increasing resilience, which may be complementary to actions to reduce exposure, are promising but as yet unproven approaches to reducing risk.
Here, we review and evaluate various individual-level strategies for reducing risk, based on the available evidence to date. The scope of this review is limited to ambient (outdoor-source) air pollution, including exposure to outdoor-source air pollution that occurs indoors, where many individuals spend the majority of their time. The association of indoor and outdoor air pollution is governed by mass balance equations (4), which are modified by many of the interventions to reduce indoor exposure to air pollutants that are reviewed below. Our goal is not to systematically review alternative approaches to reducing exposure and risk from outdoor-source air pollutants, but rather to provide a broad perspective on what we know and what we don’t know about individual-level interventions to mitigate health risks from air pollution.
Reducing personal exposure to ambient air pollution
Staying indoors
Personal exposure to ambient air pollutants occurs in both indoor and outdoor environments, and the levels of exposure depend on the fractions of time an individual spends in various indoor and outdoor environments, as well as the concentrations of outdoor-source air pollutants in those indoor and outdoor environments. In the developed world, people spend about 90% of their daily time indoors on average, with about 70% of their daily time in residential homes (5). There is a lack of information on personal activity patterns in the developing world. Although ambient air pollutants such as particulate matter, ozone, and other gases infiltrate indoors from outdoors, concentrations are generally lower indoors compared to outdoors, and spending time indoors generally reduces exposure to ambient air pollutants. Indeed, environmental protection agencies in a number of countries advise members of the public to remain indoors as part of guidance to reduce exposure and thus acute health risk on high air pollution days (6). However, it is worth noting that infiltration rates vary widely due to differences in building structures, indoor surface materials, air handling systems, building operating conditions, and ambient environmental conditions (e.g., wind speed and direction, temperature, and air pollutant constituents). Concentrations of indoor air pollutants of ambient origin are primarily determined by the process of outdoor-to-indoor transport, which is a function of air exchange rate (building ventilation). Closed windows, usually associated with use of air conditioning in the developed world, can reduce air exchange rates by about 50% (7), leading to reduced infiltration of ambient air pollutants to the indoor environment.
Personal exposure to ambient pollutants in the indoor environment is complicated by indoor air chemistry, through which some ambient pollutants are degraded (e.g., O3 and nitrate particles) and other new air pollutants are formed (e.g., aldehydes and ammonia) (8). Concentrations of ozone indoors have been found to range widely from 10% to 80% of outdoor concentrations, with means of 40-50%, due to loss of ozone by chemical reactions that occur primarily on interior surfaces (9). The effectiveness of staying indoors to reduce exposure to outdoor-source PM is more limited due to typical penetration factors which can approach unity in the absence of air conditioning (10), and relatively little loss of particles to surface deposition. Evidence that closing windows reduces penetration of PM and associated cardiovascular health risk came from a recent study of 300 healthy adults in Taipei who alternately opened and closed windows at home for 2-week periods. Lin et al. [2013] found associations between PM levels and adverse changes in markers of cardiovascular disease risk (increased plasma CRP and fibrinogen, and decreased heart rate variability) after periods with windows open, but no changes with windows closed (11).
Recommendations to spend more time indoors or make buildings “tighter” to reduce penetration of ambient pollutants are further complicated by variable indoor sources of air pollutants and the theoretical net risk from the different air pollutants that may be encountered indoors from both indoor and outdoor sources (Figure 1). Staying indoors and decreasing home ventilation reduces personal exposures to pollutants of outdoor origin, but at the same time may potentially increase personal exposures and health risks from a variety of indoor-generated primary and secondary air pollutants, including volatile organic compounds from consumer products and building materials, and nitrogen oxides, carbon monoxide and particulate matter from indoor combustion activities such as cooking, wood burning, and smoking tobacco products. For example, Huang et al. [2014] reported that levels of indoor PM were associated with decreased heart rate variability (HRV) among housewives. After adjustment for confounders, an interquartile range increase in PM2.5 was associated with statistically significant 1.25-4.31% decreases in standard deviation of normal to normal (SDNN) and 0.12-3.71% decreases in root mean squared of successive differences (rMSSD) HRV, and these effects were stronger during stir-frying, cleaning with detergent, and burning incense (12).
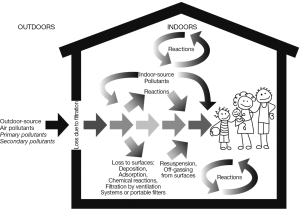
Cleaning indoor air
Portable or central air cleaning systems can reduce concentrations of indoor air pollutants, of either outdoor or indoor origin. MacIntosh et al. [2008] conducted an indoor air quality study to characterize particle removal efficiencies of several types of central, in-duct air filters/cleaners (13). The authors observed that indoor particles with diameters 0.3-0.5 µm were effectively removed by either placing a 5-inch pleated media filter (model BAYFTAH26M, Trane Residential Systems) or an electrostatic air cleaner in the ventilation duct. The application of the 5-inch pleated media filter reduced the indoor/outdoor (I/O) ratio of 0.3-0.5 µm particles 0.8 to 0.2 (75% decrease, 95% CI: 74-76%), and the electrostatic air cleaner reduced the I/O ratio from 0.8 to 0.05 (a 94% decrease, 95% CI: 93-95%) under typical indoor settings specified in Meng et al., [2009] (7). Macintosh et al. [2008] further observed that PM2.5 can also be removed effectively by 1-inch and 5-inch pleated media filters (model BAYFTAH26M, Trane Residential Systems) in the ventilation duct (13). Under typical indoor settings, the 1-inch and 5-inch pleated media filters reduced I/O ratio of PM2.5 from 0.40 to 0.27 (a 32.5% decrease, 95% CI: 29-36%) and from 0.40 to 0.08 (an 80% decrease, 95% CI: 79-81%), respectively (7). Practical considerations that may limit the use of increased filtration include added energy costs, noise, and wear and tear to the ventilation system.
Macintosh et al. [2010] modeled the health benefits of using a whole house in-duct air cleaner (14). The indoor-outdoor ratio of PM2.5 will decrease from 0.57 with natural ventilation (passive air exchange through windows and other openings), to 0.35 with conventional in-duct filtration, to 0.1 with HEPA (high efficiency particle air) in-duct filtration. Based on modeling of the metropolitan areas of Cincinnati, Cleveland, and Columbus, Ohio, reduction in PM2.5 I/O ratio from 0.57 to 0.1 after adoption of in-duct HEPA filtration would lead to estimated annual decreases of 700 (0.014%) premature deaths, 940 (0.019%) hospital and ER visit, and 130,000 (2.6%) asthma attacks
In addition to filtration in heating ventilation and air conditioning (HVAC) systems, portable filter-based air cleaners have also been used to reduce indoor levels of PM2.5 and assess potential impacts of these reductions on acute health-related biomarkers in controlled experiments. Macintosh et al. [2008] reported that the PM2.5 can also be effectively removed with a single portable air cleaner with HEPA filter (13). Under typical conditions (7), the operation of a single portable air cleaner with HEPA filter led to a decrease of I/O ratio from 0.4 to 0.14 (a 65% decrease, 95% CI: 63-67%). The actual removal rate is expected to be dependent upon the size of interior space, the ventilation rate, and the flow rate of the portable air cleaner. Bräuner et al. [2008] conducted a randomized double-blind, crossover study to quantify the impact of a portable HEPA filter-based indoor air intervention on microvascular function for healthy elderly individuals in Copenhagen (15). The HEPA filter intervention reduced both indoor PM2.5 mass concentrations (from 12.6 to 4.7 µg/m3) and particle number concentrations (from 10,016 to 3,206 particles/cm3), leading to an 8.1% (95% CI: 0.4-26.3% improvement in microvascular function. Another study in an area with prevalent wood smoke (Vancouver, BC area) used a similar HEPA filter intervention and reported similar declines in indoor PM levels as well as improved microvascular function (16).
Reducing the effective inhaled dose of air pollution
In addition to staying indoors, with or without further efforts to reduce indoor pollutant levels, reducing exertion can reduce the amount (dose) of air pollutants that are inhaled (17), and can modify the fraction of pollutant deposited or absorbed in different regions of the respiratory tract. For example, an experimental study of healthy adults showed that total respiratory tract deposition of ultrafine particles (diameter 18). Compared to the mouth, the nose is a more effective filter for preventing particles and water-soluble gases and vapors from reaching the lung (19). Thus, breathing through the mouth at higher levels of exertion further increases the dose of pollutants that reach the lower respiratory. Another study showed that children 6-10 years old had less nasal deposition of fine particles during light exercise compared to adults, suggesting that limiting exertion in children may be especially important for reducing their exposure to PM (20).
Public health messages in different locales usually refer to avoiding vigorous, extended outdoor activity during air pollution episodes (21). Trade-offs between the health benefits of reduced inhalation exposure to air pollutants and the health benefits of physical activity per se need to be factored into individual recommendations and choices for reducing exercise at certain locations or times in order to mitigate health risks from reduce exposure to air pollution. Physical inactivity is a major risk factor for mortality and morbidity from cardiopulmonary and other diseases, and exercise has been shown to have powerful protective effects for a number chronic disease states (22). European risk assessments showed that, on average, the cardiovascular disease benefits of exercise outweigh the cardiovascular disease risks of increased exposure to air pollution associated with commuting by bicycle alongside urban roadways (23,24). Reviewing available studies that were mostly European, Hartog et al. found that, although average levels of exposure to particulate matter were higher during car driving than bicycle riding within the same study, inhaled dose was estimated to be higher during cycling due to increased minute ventilation (23). They conducted a risk assessment based on estimated street-level pollution levels in Amsterdam (in-vehicle and roadway PM2.5 of about 35-40 µg/m3), and found that the cardiovascular benefits of replacing short car trips with cycling greatly outweighed the risk from increased exposure to outdoor air pollution. Similar assessments have not been done to compare exercising indoors to exercising outdoors, or avoiding exercise both indoors and outdoors, either regularly or on high pollution days. Comprehensive evaluations would take into account differential individual risk and benefit profiles based on susceptibility to adverse effects of air pollution, relative benefits from exercise, dose of air pollution, intensity of exercise, and other factors.
Avoiding outdoor activity when and where air pollutant levels are higher
Ambient air pollution levels vary seasonally, day-to-day, and by time-of-day. For example, ultraviolet light from the sun activates the chemical reactions that form ozone, generally leading to higher concentrations in late morning through early evening (25). Alternatively, ozone concentrations may peak later in the evening or at night in locations that are downwind of ozone formation (25). Levels of air pollutants also vary in different microenvironments, such as outdoors in variable proximity to sources, at home, at workplaces, in schools, in vehicles, etc. Individuals can know when air pollution levels are likely to be elevated either by sensing poor air quality (odor, irritation, symptoms), having knowledge of conditions that tend to lead to higher air pollutant levels in their area, or via public communications based on measured or predicted levels at air monitoring stations. In order to most effectively adjust behavior to reduce exposure and risk, individuals must be able to anticipate when and where air pollutant levels are likely to be elevated above levels thought to confer increased risk.
Ambient air pollutant concentrations are measured by air pollution monitoring networks in a number of countries around the world. These measurements are combined with mathematical models to forecast air pollutant levels over 24 to 48 hours. Both measured concentrations and predicted levels are disseminated to the public in various ways. At present, there is no accepted consensus standardization of approaches or methods, but in general, most authorities convert increasing concentrations of major air pollutants (ozone, PM2.5, PM10, carbon monoxide, nitrogen dioxide, and sulfur dioxide) into severity bands labeled with progressive degrees of risk. For example, the US EPA’s Air Quality Index (AQI) includes band ratings of ‘good, moderate, unhealthy for sensitive individuals, unhealthy, very unhealthy, and hazardous’. The Common Air Quality Index (CAQI), used on the European Union’s Air Quality Now website, labels band ratings as ‘very low, low, medium, high, and very high’. The AQI, CAQI and other systems use different air pollutant cut-off values to define bands. Therefore, the severity bands are not directly comparable from country to country, even though the severity terms may be the same, because ratings are generally based on how a pollutant concentration compares to national or other regulatory pollutant thresholds that vary from country to country. All of these indices simplify complex air quality information into relatively straightforward communications to the public, at the expense, to some degree, of precision and accuracy. For example, most consider each air pollutant separately, and many report a single index value based on the pollutant with the highest index value, ignoring poorly-understood, but likely important, interactive effects between different pollutants. The values of most daily indices correspond to standards for daily (24-hour) or shorter averaging times, however, long-term (annual) average standards for the pollutant may be exceeded even if the shorter-term standards are exceeded only infrequently. Aggregate indices that consider the conjoint effects of a number of monitored air pollutants, over various averaging times, have been proposed but have not been incorporated into (26,27).
It is generally assumed that levels of air pollutants that trigger air quality alerts are below thresholds of human detection by odor, irritation, or specific symptomatic responses. However, levels or air pollutants at central monitors can misrepresent local conditions, especially during transient, local air pollution episodes that humans may sense by odor, irritation, or other responses (28). Some studies have found correlations between perceptions of air quality and monitoring data (29-32), but other studies have not (33,34).
The extent to which individuals in different communities are aware of air quality indices or alerts has varied greatly in surveys and focus groups conducted in the US, Canada, and UK (28). There is little data on the extent to which individuals change behavior to reduce exposure either in response to air quality data or perceptions of exposure. In a study of Portland and Houston in the US in 2005-2006, a third of 1,962 participants were aware of air quality alerts, but only 10-15% of individuals reported changing behavior in response to predicted poor air quality, and cited perceptions of poor air quality as driving their behavior, not official advisories (34). Similarly, in a cross-sectional study of 33,888 adult participants, in six states, in the 2005 Behavioral Risk Factor Surveillance System (BRFSS), about a third of adults with asthma and 16% without asthma reported change in outdoor activity due to media alerts (35). Individual perception of poor air quality and health professional advice greatly increased the prevalence of reported behavior change. We could find no studies that have assessed associations between health outcomes and exposure to public health advisories, physician recommendations, or actual personal behavior change to reduce exposure to air pollutants.
Reducing exposure in microenvironments near sources such as traffic
Air pollutant levels in specific microenvironments are highly variable, and direct measurements or estimates of these levels are rarely available to aid individuals in making decisions about reducing exposure, but some generalizations about expected relative levels of air pollutants under different types of conditions in particular types of microenvironments can be useful. For example, traffic-related air pollution, may present increased risk of adverse health effects to broad populations in many urban areas of the world. Traffic-related pollutants consist of particles and gases emitted from internal combustion engines, their reaction products, tire and vehicle wear, and resuspended road dust. Concentrations of these pollutants decline in steep gradients with distance from roadways, but large urban populations living and/or working in proximity to roadways, as well as commuters on roadways, are among those most likely to be exposed (36). Traffic-related air pollutants have become relatively more important in areas of the world where increased industrial air pollution controls have reduced the contribution of stationary sources to total air pollution emissions. Although per-vehicle emissions have been drastically reduced in many parts of the world, and a recent decline in total vehicle miles travelled (VMT) in the developed world, there has been a rapid increase in motor vehicle ownership and VMT travelled in developing countries (37).
Individuals can reduce exposure to air pollutants and potential adverse health effects by avoiding regular physical activity alongside high-traffic roadways or near other sources of combustion such as burning of wood, biomass, or other materials. Exposure to traffic pollutants can be a rational consideration in choosing walking, biking, or exercise routes. In general, traffic pollution concentrations fall rapidly at distances from roadways, approaching background within about 500 meters, assuming no other local sources are nearby (36). Various web-based applications can assist individuals in finding alternative routes (e.g., http://www.cyclevancouver.ubc.ca/cv.aspx in Vancouver, Canada).
Individuals who commute to work in personal vehicles or public transportation receive a substantial portion of their daily dose of air pollution during commuting activities (38,39). Pollutants emitted by nearby vehicles are the main source of on-roadway exposure. Most air intake filters in passenger vehicles are relatively low efficiency and air pollutants enter through open windows, leaks in door and window seals, and other openings. Vehicle operating conditions have been shown to strongly influence concentrations of air pollutants in vehicles, with I/O ratios ranging from close to 1.0 with windows open to 0.2 or less with windows closed and ventilation set to recirculate cabin air. Vehicle speed and age also strongly affecting I/O ratios (40). Reductions in I/O ratio are generally greater in vehicles with cabin recirculation filters that are becoming more common in later model passenger vehicles. Reductions of in-cabin PM exposure of up to 40% with cabin filters have been observed (41). Among a panel of 60 healthy adults commuting 2 hours by car in Taipei, Chuang et al. [2013] found that associations between in –vehicle PM2.5 and acute decreases in HRV were modified by keeping the ventilation system in recirculation mode with the air conditioner on (42). An interquartile range increase in PM2.5 was associated with a 4.8% (95% CI: 2.9-6.7%) decrease in SDNN and 6.9% (95% CI: 5.9-7.9%) decrease in RMSSD with air conditioner off, compared to 0.7% (95% CI: 0.3-1.1%) decrease in SDNN and 0.1 (95% CI: −1.4-1.6) decrease in RMSSD with air conditioner on, with P value for the interaction in both comparisons
Personal protective equipment—respirators
In some urban areas around the world, it is not unusual to observe individuals wearing various types of respirators on urban streets in order to reduce exposure to air pollutants. The ability of a respirator to remove contaminants from inhaled air depends on the contaminant, type of filter or adsorbent material, respirator type and conditions of use. Although, relatively inexpensive respirators with filter material for particulate matter are widely available, no single absorbent, or available combination of adsorbents, can efficiently remove the various gas phase air pollutants that may be encountered. Gaseous pollutants can be removed based on their physicochemical properties, such as reactivity, molecular weight, and volatility. Therefore, the removal mechanism for different gaseous pollutant can be quite different, i.e., chemical reaction vs. adsorption; and a particular adsorbent is only suitable for removal one or a groups of pollutants with similar physicochemical properties. In general, assuming that the filter or adsorbent material is appropriate for the type of air pollutant, the efficiency of air pollutant removal by tight-fitting negative pressure respirators depends largely on the quality of the individual’s face seal. With a proper seal, the National Institutes for Occupational Safety and Health (NIOSH), which certifies respirators in the US, assigns a “protection factor” of 10 to the filtering-facepiece respirators (commonly referred to as a facemask) (43). This means that when properly worn by an individual who has been fit-tested, these respirators are expected to reduce the concentration of the air contaminant inside the facepiece to ≤10% of the concentration outside the facepiece. Fit testing and instruction in proper selection and use of respirators is a part of standards and practices for industrial respiratory protection in many countries, but may be unavailable, and perhaps impractical, when respirators are used by large populations in non-industrial settings.
Limited evidence suggests that the use of negative pressure air-purifying respirators under experimental conditions may reduce cardiovascular risks from exposure to urban PM. Langrish et al. [2009] and Langrish et al. [2012] conducted controlled intervention studies with healthy individuals and patients with coronary heart disease, who walked along an assigned route in the center of Beijing for two hours with and without a negative pressure air-purifying respirator (44,45). Among 15 healthy subjects, the authors reported that wearing the facemask was associated with decreased systolic blood pressure during the walk compared to not wearing the facemask (121 mmHg without mask vs. 114 mmHg with mask, Pvs. 65.6 ms with facemask, P44). Among 98 patients with heart disease, similar effects were observed, with the addition of reduced ST-segment depression (−142 vs. −156 µV, P=0.046) over 24 hours comparing walks with the facemask to walks without the facemask (45). This is encouraging, because presumably beneficial cardiovascular effects were nonetheless observed despite the added work of breathing imposed on the wearer by this type of negative-pressure, air-purifying respirator.
However, wearing this type of respirator has physiological effects that may confound cardiovascular effects that might be attributed to reductions in exposure to PM. For example, a study of healthy men wearing negative-pressure, air-purifying respirators while exercising at various levels on a treadmill found monotonic increases in heart rate progressing from rest to increasing levels of exercise, but systolic BP showed a biphasic response, being significantly lower at rest and higher at high levels of exercise (46). Thus, net benefit of wearing a respirator, especially in a susceptible individual for whom increased work of breathing is important, may be a complex function that does not translate simply from the actual reduction in particle exposure. Any net benefits of the practice of wearing respirators to reduce risk from ambient particulate matter air pollution will depend on the exposure reduction efficiency of the respirator and the concentration and potency of the particulate matter mixture, as well as any detrimental physiological and/or psychological effects of respiratory use. Results from single studies, like the Langrish et al. studies in Beijing, are not easily generalizable to other locales, populations, and circumstances (44,45). Additional studies are needed to replicate these findings and to clarify conditions of use that will optimize outcomes in different groups.
Regardless of the level of effectiveness at reducing exposure to air pollutants, the use of personal respiratory protection may be limited by individual and public acceptability, based on comfort, appearance, and inhibition of communication and other activities. Many find respirator contact with the face, perceived increased work-of-breathing and thermal discomfort intolerable for more than short periods of time. One study found that air temperatures at the face averaged 7.5 deg C higher during use of respirator at rest and during exercise (46). Some individuals may experience anxiety similar to claustrophobia when wearing a respirator, and facial features and facial hair may make it impossible to achieve an acceptably tight fit (43).
Knowing if one is more or less likely to be susceptible
In addition to knowing when and where exposures are, or are likely to be, more intense, individuals can better optimize the balance of personal risks and benefits by knowing if they are more likely than the general population to be particularly sensitive to harmful effects of different air pollutants. While children and young adults may be highly susceptible to some of the subclinical changes caused by air pollution (47,48), clinical events attributable to air pollution, such as myocardial infarction, stroke, or hospitalization for respiratory failure or heart failure, will of course be much more common in older individuals with advanced underlying disease such as COPD or atherosclerotic plaques. Individuals vary in sensitivity to adverse effects of air pollutants, and more-sensitive individuals are likely to obtain more benefit from efforts to reduce personal exposure (49). Generally, individuals with chronic cardiovascular or respiratory disease, children, fetuses, and the elderly are thought to be most sensitive to the major “criteria” air pollutants. Adverse effects can be distinguished as either chronic disease due to cumulative exposure over time, or acute effects of short-term exposure. For acute effects, individuals with asthma, COPD, diabetes, and underlying atherosclerotic cardiovascular disease are regarded as among the most vulnerable, due to demonstrated risk of exacerbation of these or related conditions with short-term exposure to elevated levels of air pollution (50,51). Emerging evidence suggests that the developing fetus may be especially sensitive to maternal exposure to air pollutants (48,52). In general, children and the elderly are thought to be more susceptible to air pollution effects; children due to increased body-size-adjusted dose, immature detoxifying mechanisms, and developing organ system and the elderly due to increased prevalence of chronic disease or other factors contributing to age-related loss of resilience and increased risk (53). There is some evidence that genetic variants such as polymorphisms in antioxidant genes may confer increased risk from air pollutants [see reviews (54,55) and Chen et al. in this issue (56)]. Genetic, as well as epigenetic, variation holds promise for future tailoring of interventions based on individual susceptibility, but at present there are no clinically applicable tests for varying levels of individual sensitivity to the chronic or acute health effects of air pollutants.
Interventions to modify individual susceptibility
Chronic medical conditions, such as asthma, COPD, and traditional cardiovascular disease risk factors may make individuals more susceptible to the adverse health effects of air pollution. Effective medical treatment and management of these conditions seems to be a logical first step for ameliorating increased risk from ambient or indoor pollutants although no epidemiological or clinical studies have provided direct evidence that such treatment modifies the adverse effects of air pollution. Consensus standards for managing asthma, COPD, and heart disease do include limiting exposure to ambient air pollution among guidelines for preventing exacerbation of these conditions (49,57,58). However, there is currently no direct evidence that improved clinical management reduces risk of adverse health effects from exposure to air pollution.
We know from cohort studies (Women’s Health Initiative, Six Cities) that chronic exposure to higher (not necessarily high in global terms) levels of air pollutants are indisputably associated with development of COPD and atherosclerotic CVD, including mortality (59-61). From panel studies we know that day to day, and even hour to hour changes in particulate pollution levels substantially increase risk for MI, heart failure, and stroke. From other panel studies, we have learned a great deal about the pathophysiology of these clinical outcomes (47,62-64). These studies have confirmed the important, and to some extent reversible roles for pathophysiologic processes such as oxidative stress, pulmonary and systemic inflammation, vascular/endothelial dysfunction, and increased signs of coagulation, as key processes that wax and wane acutely with air pollution and likely trigger acute events and contribute to development of chronic disease such as ASCVD. Critically, these same processes are also invoked in the pathophysiology of heart and lung disease, independent of air pollution. The overlap is remarkable but not surprising because the disease endpoints are the same. Thus, we must ask what we can learn from preventive pulmonary and cardiology methods that are applicable to the special case of air pollution’s effects on heart and lung disease.
Unfortunately, the cupboard is rather bare in terms of proven interventions for the general cardiologic case that can then be applied to the more specific case of air pollution, where no such experiments have been tried. The Mediterranean diet has been shown to both decrease total and cardiovascular mortality and to be associated with improved biomarkers of cardiovascular risk (65). However, multiple randomized controlled tests of antioxidant and vitamin supplements, based on the confirmed high levels of antioxidants in the Mediterranean and other beneficial diets, and confirmed activity in in vitro and in vivo laboratory tests, have not shown benefit and in some randomized trials have proven harmful (66). Thus antioxidant supplementation cannot be confidently recommended to counteract air pollution. Indirect antioxidants such as sulforaphane (in broccoli sprouts) have shown promising acute pilot effects but are not regarded as proven for populations (67). Statins are antioxidant as well as lipid-lowering, but these are again unproven in a population without a primary lipid-lowering indication (68,69).
Fish oil supplementation has shown beneficial effects not only on blood lipids but also on heart rate variability (68,69). Beets, and other foods rich in nitrates, do demonstrate a beneficial effect on blood pressure (70) but there is no outcomes-based evidence that supplementation of dietary nitrates, or pharmacologic control of blood pressure, is protective against cardiopulmonary effects of air pollution.
Aspirin is widely recommended and effective for reducing MI and stoke risk after a primary event and given the data showing over a doubling of MI risk and increased platelet activation with acute exposure to ambient PM, this is an attractive intervention (47,71). However, calculation of risk-benefit and duration of therapy and actual change in health outcomes or biomarkers associated with air pollution are lacking at this time. Thus, despite compelling mechanistic evidence, no specific recommendations for dietary changes or chemoprevention can be made beyond those already made for prevention of heart and lung disease in general.
Conclusions
Limited evidence supports individual actions to reduce cardiopulmonary health risks from personal exposure to ambient air pollutants by staying indoors and limiting physical exertion when air pollutant levels exceed health-based thresholds. Improved management of chronic diseases that are affected by air pollution will decrease overall risk of adverse outcomes. Available evidence is less clear about the benefits of efforts to reduce susceptibility to air pollution by pharmaceutical or chemopreventive approaches. It is clear that the relative contribution of indoor- and outdoor-generated pollutants to personal exposures depends on multiple factors, including the type of pollutants, building structure, indoor sources, and personal activities (7). Health care providers and their patients should consider these factors and tailor interventions to individual circumstances in order to maximize the net exposure reduction based on individual circumstances (53). While it may not be practical to explicitly and reliably quantify these exposures, if indoor pollutant generation can be minimized, then staying indoors makes more sense. In addition to the balance of air pollutant exposures, benefits of any reduction in exposure to air pollutants must be weighed against the physical and mental health benefits of outdoor activity. In addition to reducing outdoor activity on high pollution days, these public health messages may discourage outdoor activity at other times. The benefits of physical activity may be especially great for individuals who are also more sensitive to air pollution, such as those with heart and respiratory disease. On the other hand, the balance will tip more towards limiting activity as air pollution concentrations reach higher levels, on days with particularly poor air quality, or in areas with chronically elevated levels of air pollution. Encouraging individuals to exercise at locations and times when air pollutant levels are lower may help to preserve the benefits of exercise, while minimizing the health risks from exposure to air pollution. To our knowledge, no explicit formulae for calculating and optimizing this risk-benefit ratio are available at this time.
Acknowledgements
Funding: This work was supported by NIH grant ES005022.
Disclosure: The authors declare no conflict of interest.
References
- United Nations, Department of Economic and Social Affairs, Population Division (2014). World Urbanization Prospects: The 2014 Revision, Highlights (ST/ESA/SER.A/352).
- Pope CA 3rd, Ezzati M, Dockery DW. Fine-particulate air pollution and life expectancy in the United States. N Engl J Med 2009;360:376-86. [PubMed]
- Laden F, Schwartz J, Speizer FE, et al. Reduction in fine particulate air pollution and mortality: Extended follow-up of the Harvard Six Cities study. Am J Respir Crit Care Med 2006;173:667-72. [PubMed]
- Nazaroff WW. Indoor particle dynamics. Indoor Air 2004;14 Suppl 7:175-83. [PubMed]
- Klepeis NE, Nelson WC, Ott WR, et al. The National Human Activity Pattern Survey (NHAPS): a resource for assessing exposure to environmental pollutants. J Expo Anal Environ Epidemiol 2001;11:231-52. [PubMed]
- Plaia A, Ruggieri M. Air quality indices: a review. Rev Environ Sci Bio 2011;10:165-79.
- Meng QY, Spector D, Colome S, et al. Determinants of Indoor and Personal Exposure to PM(2.5) of Indoor and Outdoor Origin during the RIOPA Study. Atmos Environ (1994) 2009;43:5750-8. [PubMed]
- Weschler CJ. Ozone in indoor environments: concentration and chemistry. Indoor Air 2000;10:269-88. [PubMed]
- Weschler CJ. Ozone's impact on public health: contributions from indoor exposures to ozone and products of ozone-initiated chemistry. Environ Health Perspect 2006;114:1489-96. [PubMed]
- Thatcher TL, Layton DW. Deposition, Resuspension, and Penetration of Particles within a Residence. Atomspheric Environment 1995;29:1487-97.
- Lin LY, Chuang HC, Liu IJ, et al. Reducing indoor air pollution by air conditioning is associated with improvements in cardiovascular health among the general population. Sci Total Environ 2013;463-464:176-81. [PubMed]
- Huang YL, Chen HW, Han BC, et al. Personal exposure to household particulate matter, household activities and heart rate variability among housewives. PLoS One 2014;9:e89969. [PubMed]
- Macintosh DL, Myatt TA, Ludwig JF, et al. Whole house particle removal and clean air delivery rates for in-duct and portable ventilation systems. J Air Waste Manag Assoc 2008;58:1474-82. [PubMed]
- Macintosh DL, Minegishi T, Kaufman M, et al. The benefits of whole-house in-duct air cleaning in reducing exposures to fine particulate matter of outdoor origin: a modeling analysis. J Expo Sci Environ Epidemiol 2010;20:213-24. [PubMed]
- Bräuner EV, Forchhammer L, Møller P, et al. Indoor particles affect vascular function in the aged: an air filtration-based intervention study. Am J Respir Crit Care Med 2008;177:419-25. [PubMed]
- Allen RW, Carlsten C, Karlen B, et al. An air filter intervention study of endothelial function among healthy adults in a woodsmoke-impacted community. Am J Respir Crit Care Med 2011;183:1222-30. [PubMed]
- Panis LI. Cycling: health benefits and risks. Environ Health Perspect 2011;119:a114; author reply a114-a114; author reply a115.
- Daigle CC, Chalupa DC, Gibb FR, et al. Ultrafine particle deposition in humans during rest and exercise. Inhal Toxicol 2003;15:539-52. [PubMed]
- Heyder J, Gebhart J, Rudolf G, et al. Deposition of particles in the human respiratory tract in the size range 0.005–15 µm. J Aerosol Sci 1986;17:811-25.
- Bennett WD, Zeman KL, Jarabek AM. Nasal contribution to breathing and fine particle deposition in children versus adults. J Toxicol Environ Health A 2008;71:227-37. [PubMed]
- Campbell ME, Li Q, Gingrich SE, et al. Should people be physically active outdoors on smog alert days? Can J Public Health 2005;96:24-8. [PubMed]
- Warburton DE, Nicol CW, Bredin SS. Health benefits of physical activity: the evidence. CMAJ 2006;174:801-9. [PubMed]
- Hartog JJ, Boogaard H, Nijland H, et al. Do the health benefits of cycling outweigh the risks? Cien Saude Colet 2011;16:4731-44. [PubMed]
- Rojas-Rueda D, de Nazelle A, Teixidó O, et al. Replacing car trips by increasing bike and public transport in the greater Barcelona metropolitan area: a health impact assessment study. Environ Int 2012;49:100-9. [PubMed]
- Air Quality Criteria for Ozone and Other Photochemical Oxidants (2006 Final). Available online: http://cfpub.epa.gov/ncea/cfm/recordisplay.cfm?deid=149923
- Ruggieri M, Plaia A. An aggregate AQI: Comparing different standardizations and introducing a variability index. Sci Total Environ 2012;420:263-72. [PubMed]
- Kyrkilis G, Chaloulakou A, Kassomenos PA. Development of an aggregate Air Quality Index for an urban Mediterranean agglomeration: relation to potential health effects. Environ Int 2007;33:670-6. [PubMed]
- Johnson BB. Experience with urban air pollution in Paterson, New Jersey and implications for air pollution communication. Risk Anal 2012;32:39-53. [PubMed]
- Cole DC, Pengelly LD, Eyles J, et al. Consulting the community for environmental health indicator development: the case of air quality. Health Promot Int 1999;14:145-54.
- Day R. Place and the experience of air quality. Health Place 2007;13:249-60. [PubMed]
- Bonnes M, Uzzell D, Carrus G, et al. Inhabitants' and Experts' Assessments of Environmental Quality for Urban Sustainability. J Soc Issues 2007;63:59-78.
- Hunter PR, Bickerstaff K, Davies MA. Potential sources of bias in the use of individual's recall of the frequency of exposure to air pollution for use in exposure assessment in epidemiological studies: a cross-sectional survey. Environ Health 2004;3:3. [PubMed]
- Zeidner M, Shechter M. Psychological responses to air pollution: Some personality and demographic correlates. J Environ Psychol 1988;8:191-208.
- Semenza JC, Wilson DJ, Parra J, et al. Public perception and behavior change in relationship to hot weather and air pollution. Environ Res 2008;107:401-11. [PubMed]
- Wen XJ, Balluz L, Mokdad A. Association between media alerts of air quality index and change of outdoor activity among adult asthma in six states, BRFSS, 2005. J Community Health 2009;34:40-6. [PubMed]
- HEI Panel on the Health Effects of Traffic-Related Air Pollution. 2010. Traffic-Related Air Pollution: A Critical Review of the Literature on Emissions, Exposure, and Health Effects. HEI Special Report 17. Health Effects Institute, Boston, MA.
- The future of driving: Seeing the back of the car. Available online: http://www.economist.com/node/21563280
- de Nazelle A, Nieuwenhuijsen MJ, Antó JM, et al. Improving health through policies that promote active travel: a review of evidence to support integrated health impact assessment. Environ Int 2011;37:766-77. [PubMed]
- Zuurbier M, Hoek G, Oldenwening M, et al. Commuters' exposure to particulate matter air pollution is affected by mode of transport, fuel type, and route. Environ Health Perspect 2010;118:783-9. [PubMed]
- Hudda N, Kostenidou E, Sioutas C, et al. Vehicle and driving characteristics that influence in-cabin particle number concentrations. Environ Sci Technol 2011;45:8691-7. [PubMed]
- Xu B, Zhu Y. Investigation on lowering commuters’ in-cabin exposure to ultrafine particles. Transp Res Part D: Transport Environ 2013;18:122-30.
- Chuang HC, Lin LY, Hsu YW, et al. In-car particles and cardiovascular health: an air conditioning-based intervention study. Sci Total Environ 2013;452-453:309-13. [PubMed]
- Bollinger N. eds. NIOSH Respirator Selection Logic. Cincinnati: Createspace, 2004.
- Langrish JP, Mills NL, Chan JK, et al. Beneficial cardiovascular effects of reducing exposure to particulate air pollution with a simple facemask. Part Fibre Toxicol 2009;6:8. [PubMed]
- Langrish JP, Li X, Wang S, et al. Reducing personal exposure to particulate air pollution improves cardiovascular health in patients with coronary heart disease. Environ Health Perspect 2012;120:367-72. [PubMed]
- Jones JG. The physiological cost of wearing a disposable respirator. Am Ind Hyg Assoc J 1991;52:219-25. [PubMed]
- Rich DQ, Kipen HM, Huang W, et al. Association between changes in air pollution levels during the Beijing Olympics and biomarkers of inflammation and thrombosis in healthy young adults. JAMA 2012;307:2068-78. [PubMed]
- Wright RJ, Brunst KJ. Programming of respiratory health in childhood: influence of outdoor air pollution. Curr Opin Pediatr 2013;25:232-9. [PubMed]
- Brook RD, Rajagopalan S, Pope CA 3rd, et al. Particulate matter air pollution and cardiovascular disease: An update to the scientific statement from the American Heart Association. Circulation 2010;121:2331-78. [PubMed]
- Janghorbani M, Momeni F, Mansourian M. Systematic review and metaanalysis of air pollution exposure and risk of diabetes. Eur J Epidemiol 2014;29:231-42. [PubMed]
- Hussain S, Laumbach R, Coleman J, et al. Controlled exposure to diesel exhaust causes increased nitrite in exhaled breath condensate among subjects with asthma. J Occup Environ Med 2012;54:1186-91. [PubMed]
- Backes CH, Nelin T, Gorr MW, et al. Early life exposure to air pollution: how bad is it? Toxicol Lett 2013;216:47-53. [PubMed]
- Laumbach RJ. Outdoor air pollutants and patient health. Am Fam Physician 2010;81:175-80. [PubMed]
- Peden DB. The epidemiology and genetics of asthma risk associated with air pollution. J Allergy Clin Immunol 2005;115:213-9. [PubMed]
- Bell ML, Zanobetti A, Dominici F. Evidence on vulnerability and susceptibility to health risks associated with short-term exposure to particulate matter: a systematic review and meta-analysis. Am J Epidemiol 2013;178:865-76. [PubMed]
- Chen Z, Salam MT, Eckel SP, et al. Chronic effects of air pollution on respiratory health in Southern California children: findings from the Southern California Children’s Health Study. J Thorac Dis 2014. [Epub ahead of print].
- Global Strategy for the Diagnosis, Management and Prevention of COPD, Global Initiative for Chronic Obstructive Lung Disease (GOLD) 2014. Available online: http://www.goldcopd.org/
- Expert Panel Report 3, Summary Report 2007: Guidelines for the Diagnosis and Management of Asthma. Available online: http://www.nhlbi.nih.gov/guidelines/asthma/asthgdln.htm
- Miller KA, Siscovick DS, Sheppard L, et al. Long-term exposure to air pollution and incidence of cardiovascular events in women. N Engl J Med 2007;356:447-58. [PubMed]
- Dockery DW, Pope CA 3rd, Xu X, et al. An association between air pollution and mortality in six U.S. cities. N Engl J Med 1993;329:1753-9. [PubMed]
- Andersen ZJ, Hvidberg M, Jensen SS, et al. Chronic obstructive pulmonary disease and long-term exposure to traffic-related air pollution: a cohort study. Am J Respir Crit Care Med 2011;183:455-61. [PubMed]
- Rückerl R, Hampel R, Breitner S, et al. Associations between ambient air pollution and blood markers of inflammation and coagulation/fibrinolysis in susceptible populations. Environ Int 2014;70:32-49. [PubMed]
- Huang W, Wang G, Lu SE, et al. Inflammatory and oxidative stress responses of healthy young adults to changes in air quality during the Beijing Olympics. Am J Respir Crit Care Med 2012;186:1150-9. [PubMed]
- Laumbach RJ, Rich DQ, Gandhi S, et al. Acute changes in heart rate variability in subjects with diabetes following a highway traffic exposure. J Occup Environ Med 2010;52:324-31. [PubMed]
- Martinez-Gonzalez MA, Bes-Rastrollo M, Serra-Majem L, et al. Mediterranean food pattern and the primary prevention of chronic disease: recent developments. Nutr Rev 2009;67 Suppl 1:S111-6. [PubMed]
- Fortmann SP, Burda BU, Senger CA, et al. Vitamin and mineral supplements in the primary prevention of cardiovascular disease and cancer: An updated systematic evidence review for the U.S. Preventive Services Task Force. Ann Intern Med 2013;159:824-34. [PubMed]
- Egner PA, Chen JG, Zarth AT, et al. Rapid and sustainable detoxication of airborne pollutants by broccoli sprout beverage: results of a randomized clinical trial in China. Cancer Prev Res (Phila) 2014;7:813-23. [PubMed]
- Schwab U, Lauritzen L, Tholstrup T, et al. Effect of the amount and type of dietary fat on cardiometabolic risk factors and risk of developing type 2 diabetes, cardiovascular diseases, and cancer: a systematic review. Food Nutr Res 2014;58.
- Billman GE. The effects of omega-3 polyunsaturated fatty acids on cardiac rhythm: a critical reassessment. Pharmacol Ther 2013;140:53-80. [PubMed]
- Siervo M, Lara J, Ogbonmwan I, et al. Inorganic nitrate and beetroot juice supplementation reduces blood pressure in adults: a systematic review and meta-analysis. J Nutr 2013;143:818-26. [PubMed]
- Peters A, Dockery DW, Muller JE, et al. Increased particulate air pollution and the triggering of myocardial infarction. Circulation 2001;103:2810-5. [PubMed]