Bronchoscopic biopsy of peripheral pulmonary lesions in 2020: a review of existing technologies
Introduction
There have been significant advances in diagnostic bronchoscopy techniques over the last two decades. Since the advent of linear endobronchial ultrasound (EBUS) in 2002, there has been an explosion of new technologies entering the field (1). With these advances comes the hope of improving diagnostic yields. This was certainly true in the case of EBUS, which is now widely viewed as the gold-standard for mediastinal staging in newly diagnosed lung cancer (2). Lung nodules, however, remain a significant challenge in many cases. With an estimated annual incidence of 1.5 million, they are common, and when malignant, represent the earliest and more curable stage of lung cancer (3).
Differentiating benign from malignant nodules requires thorough risk-of-malignancy stratification. Factors associated with increased risk include location and size of the lesion, age, smoking status, exposure to other known carcinogens (e.g., radium), gender, race and co-existing lung disease such as emphysema or idiopathic pulmonary fibrosis. Decision making can be further guided by existing societal guidelines such as those promoted by the Fleischner society for incidentally found nodules or lung-RADS for nodules discovered while performing lung cancer screening (4,5). In addition to these guidelines, risk stratification calculators can be utilized (6,7).
A tissue diagnosis is often indicated following risk stratification (8). There are three major modalities for tissue acquisition of lung nodules: surgical resection, CT-guided transthoracic needle biopsy (CT-TTNB), and bronchoscopic biopsy. Clinicians are faced with the challenge of determining the least invasive and most efficacious way to obtain a tissue sample, weighing the risks of a procedure against the diagnostic yield. Of the two minimally-invasive diagnostic modalities, CT-TTNB has been touted for its impressive diagnostic yields. A recent meta-analysis of 48 TTNB case series, for example reported a pooled diagnostic accuracy of 92.1% (9). High diagnostic yields persisted even with small nodules <1 cm. Despite this high diagnostic yield, TTNB complications, most notably pneumothorax rates of 20–25% (3,10,11) have generated interest in alternative modalities. In addition, TTNB lacks the ability to perform simultaneous staging. Quality comparative trials are lacking in the CT-TTNB literature. There may be patient selection bias in the available reports. Additionally, data for nodules <2 cm is limited.
Bronchoscopic biopsy of peripheral pulmonary lesions has been associated with a wide variety of diagnostic yields, with some as high as 94% (12). A 2012 meta-analysis including multiple advanced bronchoscopic technologies reported a pooled diagnostic yield of 70%, with favorable complication rate compared to TTNB of 1.6% pneumothorax and 0.7% major bleeding. Additionally, the platform of bronchoscopy may allow for the targeting of multiple legions during the same procedure and the ability to perform simultaneous mediastinal staging, which has generated considerable interest in optimizing timely triage of patients with indeterminate pulmonary nodules (13).
A variety of novel bronchoscopic tools have emerged over recent years. In this review we will focus on several of these technologies, discussing their technical aspects and any available clinical data. Many of these technologies have only recently been introduced into clinical use, limiting available data, and many of these technologies are viewed as complementary and not studied in isolation making each component’s contribution to diagnostic yield and safety difficult to ascertain. Robotic bronchoscopy will not be covered in this review as it is being covered in an accompanying article in this journal edition. The technologies reviewed include: radial probe endobronchial ultrasound (REBUS), thin/ultrathin bronchoscopes, virtual bronchoscopic navigation (VBN), electromagnetic navigational bronchoscopy (ENB), cone beam CT (CBCT)-assisted bronchoscopy, digital tomosynthesis-assisted bronchoscopy, and bronchoscopic transparenchymal nodule access (BTPNA) and transbronchial access tools (TBAT).
REBUS
REBUS is a thin flexible ultrasound probe which comes in three sizes, the smallest of which has an outer diameter of 1.4 mm (UM-S20-17S, Olympus, Toyko, Japan) allowing it pass through the working channel of nearly all bronchoscopes. These can also be used through straight or curved guide sheaths advanced through the larger working channel of therapeutic bronchoscopes, allowing for more specific targeting in the peripheral lung. These probes provide a 360-degree view in a two-dimensional (2D) plane radiating outward from the spinning probe located near the tip of the device. They allow real-time localization of lesions distal to the physical reach of the bronchoscope or where direct visibility would otherwise be limited or not possible (a nodule located adjacent to an airway, for example). Solid lesions are often detected as a circle which completely surrounds the central probe (a concentric view, seen when the REBUS probe is advanced into an airway which leads directly into a peripheral lesion, Figure 1A) or a semicircle only visible on part of the screen (eccentric view, seen when the REBUS probe is directed into an airway which makes only peripheral contact with a lesion, Figure 1B). Ground glass opacities (GGOs) can sometimes be detected by the more subtle “blizzard” pattern (Figure 1C).
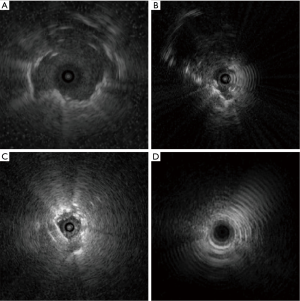
A 2011 meta-analysis of REBUS-guided bronchoscopy pooling the results of 16 studies including 1,420 patients with at least 6 months follow-up data reported a pooled diagnostic sensitivity of 73% (14). A larger meta-analysis which also included complementary technologies such as guide sheaths, ultrathin bronchoscopes, electromagnetic navigation, and virtual navigation, showed similar pooled sensitivities of 73% with GS and 71% without GS (13). A concentric REBUS signature is associated with higher diagnostic yield compared to an eccentric view, 87% vs. 42%, respectively (15). Complication rates are similar to those seen with standard non-guided transbronchial biopsy, with pneumothorax rate of 1%, of which 0.4% required chest tube placement (14).
While REBUS provides the proceduralist an opportunity to visualize lung nodules which may not be otherwise be visible with other modalities, it does have limitations. GGOs are visualized as a “blizzard” pattern (Figure 1C); in practice this pattern is often difficult to appreciate, especially with small lesions. Additionally, false positives are frequent as bleeding, saline, and atelectasis occur during the procedure and may be mistaken for the lung nodule, perhaps explaining the substantial drop-off rate between identification of lung nodules and actual diagnostic yield (16). Finally, these probes do not assist in more precise localization of a nodule located eccentrically to the probe/airway. An eccentric REBUS nodule signature tells the operator that the nodule is next to the airway but provides no information about where the nodule is in three-dimensional (3D) space in relation to the airway. The operator must use other means to decide which way to manipulate the bronchoscope or guide sheath to accomplish successful access of the eccentric peripheral lesion.
Thin and ultrathin bronchoscopy
Thin or ultra-thin bronchoscopes do not have consensus definitions. When referenced in existing literature, they typically imply bronchoscopes with an outer diameter <4.0 and <3.0 mm, respectively (17,18). This is in contrast to the approximate 6.0 mm outer diameter of therapeutic or treatment bronchoscopes (19,20). The narrow outer diameter of thin and ultrathin bronchoscopes allow for improved access to subsegmental airways, and, presumably, to peripheral lung nodules themselves. While standard bronchoscopes may be able to reach 3rd or 4th generation airways, UTBs are reported to reach up to the 9th generation (21).
A 2015 prospective multicentered randomized controlled trial examined the diagnostic yield of a thin bronchoscope with a guide sheath and an ultrathin bronchoscope which have an out diameter of 4 and 3 mm respectively (18). This study enrolled 310 patients with lung nodules <30 mm in size, of which 305 underwent bronchoscopy. Procedures were additionally guided by fluoroscopy, VBN, and REBUS in both groups. Despite only a 1 mm difference in diameter, ultrathin bronchoscopy was shown to be superior to thin bronchoscopy, with histological diagnostic yield in the ultrathin group of 74% (111 of 150 patients) vs. 59% (92 of 155 patients) in the thin bronchoscope with guide sheath group (absolute difference 14.6%, 95% CI: 5.8–23.4%). Importantly, unconfirmed but suspect benign diagnoses were confirmed by radiologic size stability and clinical compatibility during the follow-up period for at least 1 year after bronchoscopy. A recently published RCT examined the diagnostic yield between thin and ultrathin bronchoscopy. Three hundred and fifty-six patients with pulmonary nodules of a median size of 19 mm were randomized to bronchoscopy with a thin or ultrathin scope. The diagnostic yield was significant better in the ultrathin group (70.1% vs. 598.7%) (22).
This diagnostic yield has not been universally reported in studies of thin and ultrathin bronchoscope, however. A prospective 2018 trial comparing traditional fluoroscopically-guided transbronchial forceps biopsy using a standard 5.0 mm OD bronchoscope vs. thin bronchoscopy with REBUS ± guide sheath and fluoroscopic guidance demonstrated decidedly lower yields of 49% and 37%, respectively, despite expert bronchoscopists and a generous average lesion size of 31 mm (23). This study has been criticized for its use of cytobrushes instead of needle biopsies for rapid on-site cytology, fairly rigid methodology allowing for only 5 forceps transbronchial biopsies at the target site, and lack of sampling via transbronchial needle aspiration suggested by prior work to be the highest yield diagnostic tool in the peripheral lung (24,25), all of which might have unfavorably impacted diagnostic yield.
Overall, studies of thin and ultrathin bronchoscope suggest that narrower bronchoscopes used in conjunction with complementary technologies such as fluoroscopy, REBUS, guide sheaths, and navigational systems improves the ability to successfully access peripheral lung lesions, though this has not been a universal finding. Complication rates were infrequently reported in these studies but case reports of pleural perforation have been made (26).
VBN
Accessing lung nodules necessitates accurate selection of branching bronchi, which represents a significant technical and spatial-reasoning challenge. VBN utilizes a helical CT scan to generate 3D images of the tracheobronchial tree (27). During bronchoscopy, the computerized navigational system recognizes the visual appearance of airways during bronchoscopic maneuvering and guides the user to make correct turns into subsequent subsegmental branches on the way to the operator pre-specified peripheral lesion. There are several VBN systems commercially available worldwide, including Bf-NAVI® (Cybernet System Inc., Tokyo, Japan), LungPoint® (Broncus Medical Inc., Mountain View, CA, USA), and DirectPath® (Cybernet System Inc.) (28).
A multicenter prospective study randomized 199 patients with lung nodules <30 mm to either VBN-assisted (VBNA) or non-VBN-assisted (NVBNA) groups. Guide sheaths were used in all procedures and sampling was also guided by REBUS probes and fluoroscopy. VBNA had significantly higher diagnostic yield than the NVBNA group (80% vs. 67%, respectively, P=0.03) (29). A 2014 systematic review of VBN showed an average diagnostic yield of 74% in all lesions and 67% in small lesions <20 mm. Other complementary technologies used in these studies included UTB, fluoroscopy, GS, and REBUS (28).
VBN appears helpful in planning procedures and aids operators in approximating the bronchoscope and the peripheral lesion by facilitating selection of correct subsegmental bronchi on the pathway to the lesion. VBN does not include a mechanism for making real time adjustments for CT-to-body divergence (the difference in nodule location between the pre-operative CT and real-time imaging) or provide electronic tool tip location feedback to the operator.
ENB
ENB systems utilize a pre-procedure CT scan-derived virtual tracheobronchial tree, on which target lesions are designated by the operator, similarly to VBN. During the procedure, an electromagnetic field is generated by a board placed under or above the patient’s thorax and electromagnetic sensors are placed on the patient. These sensors triangulate electromagnetic catheters or biopsy tools within the patient in real time. Currently there are two commercially-available ENB systems: superDimension (Medtronic, Minneapolis, MN, USA) and SPiNDrive (Veran Medical Technologies, Inc, St Louis, MO, USA). Within the superDimension platform, a blunt-tip electromagnetic probe affixed to the distal aspect of a curved guide sheath (with multiple degrees of curvature available) is used to direct the sheath toward the target lesion. Once in position, the electromagnetic guide is removed, leaving the guide sheath in place as a channel for biopsy instruments to be introduced. SPiNDrive, by contrast, utilizes tools with electromagnetically-tracked tips allowing for real time tracking as biopsy samples are collected, and also offers support for transthoracic needle aspiration via an electromagnetic tip-tracked transthoracic biopsy needle. A multicenter case series was recently published showing a diagnostic yield of 73.7% in 129 cases with an average nodule size of 27.31 mm. This increased to 81.1% when bronchoscopy was also performed (30).
A 2014 review showed pooled diagnostic yield from 15 studies containing results on 1,033 lung nodules demonstrated a 64.9% diagnostic rate for ENB (31). Eberhardt and colleagues reported a yield of 59% for ENB without assistance of fluoroscopy, increasing to 88% when REBUS probes were additionally used to guide the procedure (32). Complication rates similar to other bronchoscopic modalities have been reported, with a pooled pneumothorax rate of 3.1%, of which 1.6% required chest tube insertion, in the aforementioned meta-analysis (31). Several factors have been associated with higher yield such as nodule size and presence of a bronchus sign (33-35).
In more real-world use settings, the diagnostic yield of ENB has been mixed. The multicenter prospective NAVIGATE trial evaluating superDimension was recently published (36). This study included 1,157 lung lesions with an average size of 20 mm, with diagnostic yield of 73% (37). This is contrast to data from the AQuIRE (ACCP Quaility Improvement Registry, Evaluation, and Education) registry, in which a yield of 47.1% was noted when ENB and REBUS were used to biopsy lung nodules (25). There are many potential reasons for the discrepancy in these yields, including patient/nodule selection, operator/institution experience (median of 14 ENB cases per year per physician in AQuIRE vs. 45% of operators in NAVIGATE reporting 5–10 ENB cases per month and another 45% reported >10 per month), utilization of rapid on-site cytology during the procedure (31% in AQuIRE vs. 69% in NAVIGATE), a high degree of interinstitutional practice variability in the AQuIRE registry, and underuse of transbronchial needle aspiration in the AQuIRE registry (only 16% of cases, with four or more needle passes in only 24% when actually utilized). The discrepancy in diagnostic yield centering on operator experience and appropriate biopsy tool selection also highlights the fact that advanced bronchoscopic technologies may have significant learning curves, implying the technologies themselves are not sufficient in guaranteeing high diagnostic yields.
There are some limitations to ENB systems, particularly CT-to-body divergence (35), which occurs due to respiration, body positioning, atelectasis, and cardiac motion. Nodules in the lower lobes, for example, have been demonstrated to move up to 2.5 cm between inspiratory and expiratory CT scans (38). Additionally, catheter-to-nodule deflection occurs as a result of stiff tools being placed through relatively pliable guide sheaths. For example, a curved guide sheath may be precisely aligned with the nodule without a tool inside it, but be significantly deflected when a biopsy tool is advanced through it.
Potential solutions to minimize CT-to-body divergence in ENB and VBN procedures include optimization of ventilator strategies to reduce the risk of atelectasis and use of near-real-time imaging with intraprocedural CBCT and/or digital tomosynthesis fluoroscopy. Instrument or catheter-based deflection has been addressed with continuous electromagnetic tracking of catheters and biopsy instruments.
ENB with fluoroscopic digital tomosynthesis [fluoroscopic navigation (F-Nav)]
One major limitation of all ENB and VNB platforms is the reliance on a pre-operative CT for planning and navigation as opposed to real-time imaging guidance. Though ENB is often used with 2D fluoroscopy, the resolution needed to see small or semi-solid lung nodules is frequently lacking. Digital tomosynthesis, also referred to as F-Nav, is a method for performing high-resolution limited-angle tomography at radiation dose levels comparable with standard projection radiography (39).
F-Nav consists of a traditional ENB, followed by a limited angle spin of the standard 2D fluoroscopic C-arm, with images recorded and processed to yield a digital tomosynthesis image of the nodule. This enhances the visual appearance of the lesion, revealing nodules not otherwise visible on standard 2D fluoroscopy, including pure ground glass and cavitary lesions. Additionally, by marking the tip of the guide sheath and the lesion, the ENB system can update the real-time location of the nodule in the virtual workspace, directing the operator to manipulate the catheter in an optimal manner to direct biopsy tools into the lesion (Figure 2). This technology was the subject of a retrospective before-and-after study in which 168 consecutive nodules were analyzed at a single center. Standard ENB (ENB) with 2D fluoroscopy was used on 101 of the nodules. F-Nav was subsequently introduced and the remaining 67 cases were analyzed. ENB had a diagnostic yield of 54% while F-Nav had a diagnostic yield of 79% (P=0.0019), with comparable nodule sizes in both groups (median 15 vs. 16 mm). Complications rates were equivalent between the two groups with a pneumothorax rate of 1.5% and no significant bleeding (35). This emerging technology, currently commercially available as a component of the superDimension ENB system, will require additional study to confirm these results.
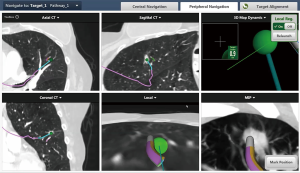
CBCT-guided bronchoscopy
CBCT-guided bronchoscopy offers near real-time high-resolution imaging which demonstrates the relationship between the target lesion and bronchoscope or guide sheath (Figure 3). The target lesion can subsequently be segmented at a workstation, creating a virtual lesion which is subsequently overlaid upon 2D fluoroscopy (resulting in so-called augmented fluoroscopy) during actual biopsy passes (Figure 3B). There limited existing data on this emerging technique. In one retrospective 2019 study, 75 consecutive patients with a total of 93 Lung nodules were evaluated after undergoing CBCT-guided bronchoscopy, with diagnostic yield of 83.7% and pneumothorax rate of 4% (40). Previously observed associations of diagnostic yield with the location, size, and presence of a bronchus sign were not observed. Another small feasibility trial reported achieving a diagnosis in 17 of 22 cases (77%), which included a transbronchial access to TBAT in seven cases (41). Another 20 case pilot study combining TB/UTB with cone beam guidance reported a diagnostic yield of 70% (42).
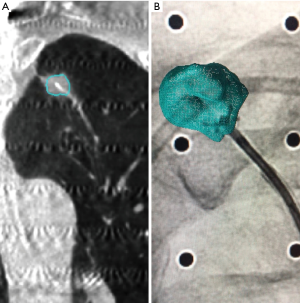
BTPNA/TBAT
The presence of bronchus sign has been a positive predictor for diagnostic yield (33,35). A bronchus sign refers to the presence of a bronchus leading directly to the nodule (43). BTPNA and TBAT are new modalities designed to overcome the limitations of the airways in which bronchoscopes travel, specifically for nodules without a bronchus sign or without a nearby airway present. BTPNA generates a route through the lung parenchyma to a lung nodule while avoiding blood vessels. BTPNA is part of the VBN system Archimedes (Broncus Medical, Inc, San Jose, CA, USA). The first-in-human BTPNA report was in 2015, in which 12 patients underwent BTPNA with adequate biopsies in 83% and no significant complications (44). Much more research is required before making an informed assessment of it safety profile.
TBAT, a component of the CrossCountry system (Medtronic, Minneapolis, MN, USA), is another technology being investigated to access peripheral lesions without a bronchus sign. CrossCountry utilizes the superDimension ENB to generate a virtual pathway to the lesion. A needle guidewire advanced through the working channel of the bronchoscope is used to puncture the bronchial wall at a specified location, creating an entry point for a cone-shaped dilator followed by a GS. The first in human procedures using this technology were in 2015. In this report, 10 out 12 nodules were successfully accessed using Archimedes and fluoroscopy (83% yield) (44). A large multicenter trial involving TBAT (EAST-2 trial: ClinicalTrial.gov registration no. NCT02867371) is currently recruiting patients and will hopefully to provide further data on this technique’s safety and diagnostic yield.
Conclusions
Advanced bronchoscopy for the diagnosis of peripheral lung lesions involves many complementary technologies, many of which seem to improve diagnostic yield. The bulk of extant data regarding advanced peripheral lung diagnostic techniques is derived from prospective observational or retrospective data, which has resulted in wide ranges of reported diagnostic efficacy. Advancements in lung cancer screening require safe, efficacious diagnostic tools for the pulmonology community to appreciate the long sought-after stage shift in lung cancer diagnosis. The modalities reviewed here had similar complication rates though many lack rigorous studies to best inform their safety profile. As the field continues to mature there will be increasing demand for high-quality multicenter randomized controlled trials to validate diagnostic yield and safety results to inform the pulmonology community how best to identify and characterize lung nodules.
Acknowledgments
Funding: None.
Footnote
Provenance and Peer Review: This article was commissioned by the Guest Editors (Fabien Maldonado and Robert Lentz) for the series “Novel Diagnostic Techniques for Lung Cancer” published in Journal of Thoracic Disease. The article was sent for external peer review organized by the Guest Editors and the editorial office.
Conflicts of Interest: All authors have completed the ICMJE uniform disclosure form (available at http://dx.doi.org/10.21037/jtd.2020.02.36). The series “Novel Diagnostic Techniques for Lung Cancer” was commissioned by the editorial office without any funding or sponsorship. RJL served as an unpaid Guest Editor for the series. FM served as the unpaid Guest Editor of the series and serves as an unpaid editorial member of Journal of Thoracic Disease from Aug 2019 to Jul 2021. FM reports grants and personal fees from Medtronic and personal fees from Erbe, outside the submitted work. OBR reports grants and personal fees from Medtronic, personal fees from Olympus America, personal fees from Auris, personal fees from Intuitive, personal fees from VERAN, outside the submitted work. JMK has no other conflicts of interest to declare.
Ethical Statement: The authors are accountable for all aspects of the work in ensuring that questions related to the accuracy or integrity of any part of the work are appropriately investigated and resolved.
Open Access Statement: This is an Open Access article distributed in accordance with the Creative Commons Attribution-NonCommercial-NoDerivs 4.0 International License (CC BY-NC-ND 4.0), which permits the non-commercial replication and distribution of the article with the strict proviso that no changes or edits are made and the original work is properly cited (including links to both the formal publication through the relevant DOI and the license). See: https://creativecommons.org/licenses/by-nc-nd/4.0/.
References
- Yang H, Zhang Y, Wang KP, et al. Transbronchial needle aspiration: development history, current status and future perspective. J Thorac Dis 2015;7:S279-86. [PubMed]
- Czarnecka-Kujawa K, Yasufuku K. The role of endobronchial ultrasound versus mediastinoscopy for non-small cell lung cancer. J Thorac Dis 2017;9:S83-97. [Crossref] [PubMed]
- Gould MK, Tang T, Liu IL, et al. Recent trends in the identification of incidental pulmonary nodules. Am J Respir Crit Care Med 2015;192:1208-14. [Crossref] [PubMed]
- McKee BJ, Regis SM, McKee AB, et al. Performance of ACR Lung-RADS in a clinical CT lung screening program. J Am Coll Radiol 2015;12:273-6. [Crossref] [PubMed]
- MacMahon H, Naidich DP, Goo JM, et al. Guidelines for management of incidental pulmonary nodules detected on CT images: from the Fleischner society 2017. Radiology 2017;284:228-43. [Crossref] [PubMed]
- McWilliams A, Tammemagi MC, Mayo JR, et al. Probability of cancer in pulmonary nodules detected on first screening CT. N Engl J Med 2013;369:910-9. [Crossref] [PubMed]
- Swensen SJ, Silverstein MD, Ilstrup DM, et al. The probability of malignancy in solitary pulmonary nodules. Application to small radiologically indeterminate nodules. Arch Intern Med 1997;157:849-55. [Crossref] [PubMed]
- Gould MK, Donington J, Lynch WR, et al. Evaluation of individuals with pulmonary nodules: when is it lung cancer? Diagnosis and management of lung cancer, 3rd ed: American College of Chest Physicians evidence-based clinical practice guidelines. Chest 2013;143:e93S-120S.
- DiBardino DM, Yarmus LB, Semaan RW. Transthoracic needle biopsy of the lung. J Thorac Dis 2015;7:S304-16. [PubMed]
- Heerink WJ, de Bock GH, de Jonge GJ, et al. Complication rates of CT-guided transthoracic lung biopsy: meta-analysis. Eur Radiol 2017;27:138-48. [Crossref] [PubMed]
- Schreiber G, McCrory DC. Performance characteristics of different modalities for diagnosis of suspected lung cancer: summary of published evidence. Chest 2003;123:115S-28S. [Crossref] [PubMed]
- Loo FL, Halligan AM, Port JL, et al. The emerging technique of electromagnetic navigation bronchoscopy-guided fine-needle aspiration of peripheral lung lesions: promising results in 50 lesions. Cancer Cytopathol 2014;122:191-9. [Crossref] [PubMed]
- Wang Memoli JS, Nietert PJ, Silvestri GA. Meta-analysis of guided bronchoscopy for the evaluation of the pulmonary nodule. Chest 2012;142:385-93. [Crossref] [PubMed]
- Steinfort DP, Khor YH, Manser RL, et al. Radial probe endobronchial ultrasound for the diagnosis of peripheral lung cancer: systematic review and meta-analysis. Eur Respir J 2011;37:902-10. [Crossref] [PubMed]
- Kurimoto N, Miyazawa T, Okimasa S, et al. Endobronchial ultrasonography using a guide sheath increases the ability to diagnose peripheral pulmonary lesions endoscopically. Chest 2004;126:959-65. [Crossref] [PubMed]
- Gildea TR. Lung Lesion Localization and the Diagnostic Drop. Ann Am Thorac Soc 2016;13:1450-2. [Crossref] [PubMed]
- Ishiwata T, Gregor A, Inage T, et al. Bronchoscopic navigation and tissue diagnosis. Gen Thorac Cardiovasc Surg 2019. [Epub ahead of print]. [Crossref] [PubMed]
- Oki M, Saka H, Ando M, et al. Ultrathin bronchoscopy with multimodal devices for peripheral pulmonary lesions. A randomized trial. Am J Respir Crit Care Med 2015;192:468-76. [Crossref] [PubMed]
- Fujifilm USA. Video bronchoscopes. Available online: https://www.fujifilmusa.com/products/medical/endoscopy/endoscopes/video-bronchoscopes/index.html#treatment. Accessed November 23, 2019.
- Olympus America. Therapeutic Bronchoscope (BF-1TH190). Available online: https://medical.olympusamerica.com/products/bronchoscope/therapeutic-bronchoscope-bf-1th190. Accessed November 23, 2019.
- Asano F, Shinagawa N, Ishida T, et al. Virtual bronchoscopic navigation combined with ultrathin bronchoscopy. A randomized clinical trial. Am J Respir Crit Care Med 2013;188:327-33. [Crossref] [PubMed]
- Oki M, Saka H, Asano F, et al. Use of an ultrathin vs thin bronchoscope for peripheral pulmonary lesions: a randomized trial. Chest 2019;156:954-64. [Crossref] [PubMed]
- Tanner NT, Yarmus L, Chen A, et al. Standard bronchoscopy with fluoroscopy vs thin bronchoscopy and radial endobronchial ultrasound for biopsy of pulmonary lesions: a multicenter, prospective, randomized trial. Chest 2018;154:1035-43. [Crossref] [PubMed]
- Katis K, Inglesos E, Zachariadis E, et al. The role of transbronchial needle aspiration in the diagnosis of peripheral lung masses or nodules. Eur Respir J 1995;8:963-6. [PubMed]
- Ost DE, Ernst A, Lei X, et al. Diagnostic Yield and Complications of Bronchoscopy for Peripheral Lung Lesions. Results of the AQuIRE Registry. Am J Respir Crit Care Med 2016;193:68-77. [Crossref] [PubMed]
- Oki M, Saka H, Kitagawa C, et al. Visceral pleural perforation in two cases of ultrathin bronchoscopy. Chest 2005;127:2271-3. [Crossref] [PubMed]
- Vining DJ, Liu K, Choplin RH, et al. Virtual bronchoscopy. Relationships of virtual reality endobronchial simulations to actual bronchoscopic findings. Chest 1996;109:549-53. [Crossref] [PubMed]
- Asano F, Eberhardt R, Herth FJ. Virtual bronchoscopic navigation for peripheral pulmonary lesions. Respiration 2014;88:430-40. [Crossref] [PubMed]
- Ishida T, Asano F, Yamazaki K, et al. Virtual bronchoscopic navigation combined with endobronchial ultrasound to diagnose small peripheral pulmonary lesions: a randomised trial. Thorax 2011;66:1072-7. [Crossref] [PubMed]
- Mallow C, Lee H, Oberg C, et al. Safety and diagnostic performance of pulmonologists performing electromagnetic guided percutaneous lung biopsy (SPiNperc). Respirology 2019;24:453-8. [Crossref] [PubMed]
- Gex G, Pralong JA, Combescure C, et al. Diagnostic yield and safety of electromagnetic navigation bronchoscopy for lung nodules: a systematic review and meta-analysis. Respiration 2014;87:165-76. [Crossref] [PubMed]
- Eberhardt R, Anantham D, Ernst A, et al. Multimodality bronchoscopic diagnosis of peripheral lung lesions: a randomized controlled trial. Am J Respir Crit Care Med 2007;176:36-41. [Crossref] [PubMed]
- Seijo LM, de Torres JP, Lozano MD, et al. Diagnostic yield of electromagnetic navigation bronchoscopy is highly dependent on the presence of a Bronchus sign on CT imaging: results from a prospective study. Chest 2010;138:1316-21. [Crossref] [PubMed]
- Bowling MR, Kohan MW, Walker P, et al. The effect of general anesthesia versus intravenous sedation on diagnostic yield and success in electromagnetic navigation bronchoscopy. J Bronchology Interv Pulmonol 2015;22:5-13. [Crossref] [PubMed]
- Aboudara M, Roller L, Rickman O, et al. Improved diagnostic yield for lung nodules with digital tomosynthesis-corrected navigational bronchoscopy: Initial experience with a novel adjunct. Respirology 2020;25:206-13. [Crossref] [PubMed]
- Folch EE, Bowling MR, Gildea TR, et al. Design of a prospective, multicenter, global, cohort study of electromagnetic navigation bronchoscopy. BMC Pulm Med 2016;16:60. [Crossref] [PubMed]
- Folch EE, Pritchett MA, Nead MA, et al. Electromagnetic navigation bronchoscopy for peripheral pulmonary lesions: one-year results of the prospective, multicenter NAVIGATE study. J Thorac Oncol 2019;14:445-58. [Crossref] [PubMed]
- Chen A, Pastis N, Furukawa B, et al. The effect of respiratory motion on pulmonary nodule location during electromagnetic navigation bronchoscopy. Chest 2015;147:1275-81. [Crossref] [PubMed]
- Thompson W, Argaez C. Digital breast tomosynthesis for the screening and diagnosis of breast cancer: a review of the diagnostic accuracy, cost-effectiveness and guidelines [Internet]. Ottawa: Canadian Agency for Drugs and Technologies in Health, 2019.
- Pritchett MA, Schampaert S, de Groot JAH, et al. Cone-Beam CT With Augmented Fluoroscopy Combined With Electromagnetic Navigation Bronchoscopy for Biopsy of Pulmonary Nodules. J Bronchology Interv Pulmonol 2018;25:274-82. [Crossref] [PubMed]
- Sobieszczyk MJ, Yuan Z, Li W, et al. Biopsy of peripheral lung nodules utilizing cone beam computer tomography with and without trans bronchial access tool: a retrospective analysis. J Thorac Dis 2018;10:5953-9. [Crossref] [PubMed]
- Casal RF, Sarkiss M, Jones AK, et al. Cone beam computed tomography-guided thin/ultrathin bronchoscopy for diagnosis of peripheral lung nodules: a prospective pilot study. J Thorac Dis 2018;10:6950-9. [Crossref] [PubMed]
- Ernst A, Anantham D. Bronchus sign on CT scan rediscovered. Chest 2010;138:1290-2. [Crossref] [PubMed]
- Herth FJ, Eberhardt R, Sterman D, et al. Bronchoscopic transparenchymal nodule access (BTPNA): first in human trial of a novel procedure for sampling solitary pulmonary nodules. Thorax 2015;70:326-32. [Crossref] [PubMed]