Carcinogenicity of ambient air pollution: use of biomarkers, lessons learnt and future directions
Exposure to air pollution and lung cancer risk
Environmental pollution encompasses a number of hazardous exposures including air, water, and chemical exposures. Ambient (outdoor) and household (indoor) air pollution is a major environmental health risk, affecting populations in developed and developing countries alike (1).
Ambient air pollution (AAP) consists of emissions of complex mixtures of air pollutants from industries, households, cars and trucks (2). Of these pollutants, fine particulate matter (PM) has been widely shown to have adverse effects on human health. Most fine PM comes from fuel combustion, both from mobile sources such as vehicles and from stationary sources such as power plants, industry, households or biomass burning (2). PM can vary in size from ultra-fine particles (UFP) ≤100 nm in diameter, to fine particles ~100 nm -2.5 μm in diameter (PM2.5), to larger particles up to 10 μm in diameter (PM10) (3), and differential PM sizes can affect pathophysiological pathways independently (3).
On the other hand, household air pollution (HAP) is the result of cooking and heating households using solid fuels (i.e., wood, charcoal, coal, dung, crop wastes) on open fires or traditional stoves. In poorly ventilated dwellings, fine PM concentrations in and around the home can exceed acceptable levels for up to 100-fold (4).
Health risks associated with air pollution include but are not limited to stroke, heart disease, lung cancer, and both chronic and acute respiratory diseases, including asthma (5-8). AAP and its health effects are much more frequently studied compared to HAP.
Of the AAP health effects, lung cancer contributes greatly to air pollution associated mortality. The association between exposure to AAP and lung cancer incidence and/or mortality has been evaluated in a number of prospective studies, which are summarized in Table 1. Despite that formal statistical significance was not always reached, the evidence linking exposure to urban air pollutants, mainly PM2.5 or PM10, and lung cancer is generally consistent. Cohorts from the United States as well as from Europe have found increased risks of lung cancer with higher exposure to PM and other substances present in polluted air, with statistically significant risk ratios (RRs) ranging from 1.14 to 5.21 (Table 1).
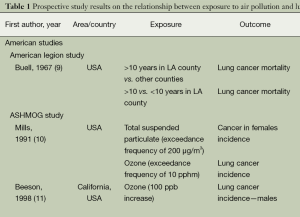
Full table
Based on the available epidemiological and molecular evidence, the International Agency for Research on Cancer (IARC) has recently classified air pollution as Carcinogenic to Humans (Group 1) (37).
Incorporation of biomarkers in measuring exposure and evaluating health effects
Biomarkers were introduced in the study of the carcinogenic effects of AAP under the assumption that they could enhance research on the health effects of air pollution, and other exposures, by improving exposure assessment, increasing the understanding of mechanisms (e.g., by measuring intermediate biomarkers), and enabling the investigation of individual susceptibility.
Biomarkers used in the epidemiology of cancer are usually divided into three categories: markers of internal dose, markers of early response, and markers of susceptibility. In fact, each category includes subcategories. For example, protein adducts and DNA adducts are both markers of internal dose, but their biological significance differs. While protein adducts are not repaired (i.e., they reflect external exposure more faithfully), DNA adducts are influenced by an individual’s repair capacity. If DNA adducts are not eliminated by the DNA repair machinery, they induce a mutation. Also, markers of early response are a heterogeneous category that encompasses DNA mutations and gross chromosomal damage. The main advantage of early response markers is that they are more frequent than the disease and can be recognized sooner, thus allowing researchers to identify earlier effects of potentially carcinogenic exposures. Finally, markers of susceptibility include several subcategories; in particular, a type of genetic susceptibility related to the metabolism of carcinogenic substances, and another type related to DNA repair.
Because of their ability to highlight mechanisms, improve exposure assessment, and reflect individual susceptibility, biomarkers have been and will continue to play a vital role in the investigation of the carcinogenicity of AAP. In this review, we assess DNA adducts as biomarkers of exposure and early biological effect, and DNA methylation as biomarker of early biological change and discuss critical issues arising from their incorporation in health impact evaluations. DNA adducts and DNA methylation are treated here as paradigms, and the lessons learned from their use in the examination of AAP carcinogenicity, can also be applied to investigations of other biomarkers involved in AAP carcinogenicity.
DNA adducts
DNA adducts are covalent bonds arising from the interaction of cancer causing chemicals such as polycyclic aromatic hydrocarbons (PAHs), or metabolites of such chemicals, with sites in DNA (38). Even though adducts can be removed by repair proteins, some can persist, and can contribute to cancer development by causing nucleotide substitutions, deletions and chromosome rearrangements during replication (38).
Several studies have considered DNA adducts as biomarkers of exposure to genotoxic carcinogens, such as PAHs, present in AAP, employing cross-sectional and case-control study designs, some nested within prospective cohorts. Studies which compared the mean DNA adduct levels in individuals with estimated high or low external exposures are summarized in Table 2, whereas studies which carried out correlation and regression analyses on all subjects are summarised in Table 3 (52-66). The majority of studies and two reviews demonstrated positive associations between exposure to air pollution or chemicals in polluted air and the formation of DNA adducts in exposed individuals. Subjects in these studies included a wide range of occupationally and residentially exposed individuals, such as policemen in Bangkok (47), Genova (45), and Prague (49,66), school children in Thailand (50), residents in an industrial area and rural controls in Poland (39), bus and taxi drivers in Stockholm (40), bus drivers in Copenhagen (41), students in Denmark and in Greece (42), as well as street vendors, taxi drivers, gasoline salesmen and road side residents in Benin (51). Only two studies reported no association (54,67).
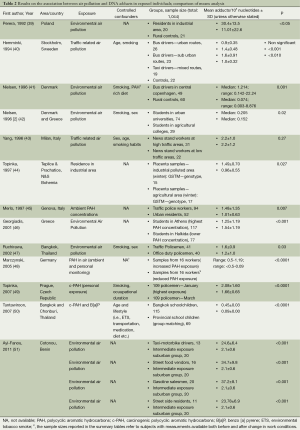
Full table
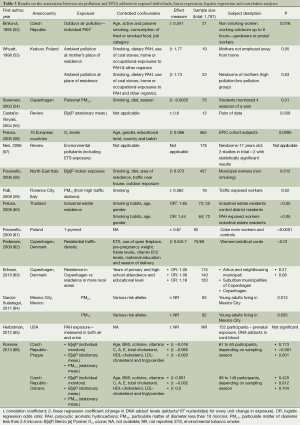
Full table
DNA adducts in children
Fetal exposures and DNA adducts in newborns also showed positive associations (44,53,65). Experimental evidence indicates that developing fetuses are more susceptible than adults to the carcinogenic effects of PAHs. To assess fetal versus adult susceptibility to PAHs and second-hand tobacco smoke, a study compared carcinogen-DNA adducts (a biomarker associated with an increased risk of cancer) and cotinine (a biomarker of exposure to tobacco smoke) in paired blood samples collected from mothers and newborns in New York City, USA. The authors enrolled 265 non-smoking African-American and Latina mother–newborn pairs between 1997 and 2001. Despite the estimated 10-fold lower fetal dose, mean levels of B[a]P-DNA adducts were comparable in paired newborn and maternal samples (0.24 adducts per 108 nucleotides in newborns, with 45% of newborns with detectable adducts, vs. 0.22 per 108 nucleotides in mothers, with 41% of mothers with detectable adducts). These results indicate an increased susceptibility of the fetus to DNA damage (68).
Dose-response relationship
Lewtas et al. [1997] (69) observed that human populations exposed to PAH via air pollution exhibit a nonlinear relationship between levels of exposure and white blood cell-DNA adducts. Among highly exposed subjects, the level of DNA adducts per unit of exposure was significantly lower than those measured after environmental exposures. The observation was confirmed in a meta-analysis of the epidemiological studies (70). The same exposure–dose nonlinearity was observed in lung DNA from rats exposed to PAHs. One interpretation proposed for such an observation is that saturation of metabolic enzymes or induction of DNA repair processes occurs at high levels of exposure (71,72).
DNA methylation
DNA methylation is a biochemical process where a methyl group is added to the cytosine nucleotides mostly found in CpG dinucleotides and this modification influences gene expression (73,74). For example, a high percentage of CpG dinucleotides in repetitive sequences are methylated to inhibit activation and maintain chromosome stability, but CpG sites in CpG islands associated with gene promoters are usually unmethylated. These unmethylated promoter regions allow for active gene transcription (75,76) and also have a role in cell differentiation.
Whole genome methylation is most commonly assessed using surrogate repetitive elements such as long interspersed nuclear element-1 (LINE-1) and Alu repeats (77). Hypomethylation of these endogenous retro-transposons can lead to activation and reposition elsewhere in the genome, causing insertional mutagenesis, transcriptional interference, and genomic instability (77-79). Further to activating repetitive DNA sequences, DNA hypomethylation might also contribute to translocations of these hypomethylated sequences, by loosening chromatin packaging (79-81).
Exposure to AAP, whether short-term or long-term, has been shown to be associated with global hypomethylation. Ten reports (Table 4) have recently investigated the effects of AAP exposure on global methylation and a number of them used repetitive elements such as LINE-1, Satα and Alu elements as proxies to whole genome methylation. LINE-1 methylation was frequently found to be altered by exposure to air pollution (82-84). Alu methylation was also significantly altered in three studies (84,85,87) and Satα in one study (88). Lastly, global methylation in healthy adults was decreased following exposure to AAP (86). Despite the small number of available studies, the replication of findings supports AAP’s influence on global methylation levels, and these epigenetic changes can contribute to carcinogenesis at least as much as genetic changes.
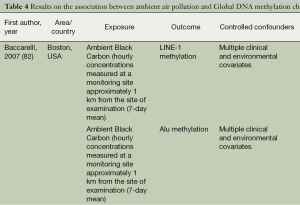
Full table
DNA hypomethylation in children and pre-natal exposures
Two studies investigated global methylation in cord blood and placenta samples and found significant associations with prenatal PAH and PM2.5 exposures (65,89). In addition, when comparing children from the polluted region of Ostrava to children from the non-polluted region of Prachatice, Rossnerova et al. [2013] (90) found 9,916 differentially methylated CpGs of which 58 had methylation differences of >10%. All these sites were found to be hypomethylated in Ostrava children demonstrating a significant impact of AAP on the methylation patterns of children.
Critical issues in evaluating the relationship between AAP and biomarkers
Using the pool of evidence on DNA-adducts and DNA methylation as paradigms, a number of critical issues in health impact evaluations using biomarkers arise and several directions for the future of the field can be drawn. The lessons learnt from the experience are critical since the mechanisms through which AAP causes cancer remain to be elucidated and biomarkers of exposure can be incorporated in more accurate exposure assessments.
Confounding
Only 17 of the studies on DNA adducts reviewed here, adjusted for various potential confounders and not all have adjusted for smoking and PAHs in diet, indicating lack of adequate adjustment for confounding. Dietary habits can affect DNA adduct formation, as studies have demonstrated strong negative associations between DNA adducts and consumption of fresh fruit and vegetables, olive oil, and antioxidants as well as positive associations between consumption of charbroiled food and DNA adducts (91,92). In addition, there is almost complete consensus amongst studies in humans, in animals and in vitro that smoking, whether active or passive, is associated with DNA adduct formation (93). Also, a recent study has evidenced city-specific spatial and temporal environmental inequalities that relate to the historical socioeconomic make-up of the cities (94). These inequalities become especially important in studies comparing subjects from different cities/rural-urban areas. Considering that PAHs in diet, smoking, exposure to second hand smoke, and socioeconomic status are factors that have an impact on DNA adduct and protein formation, inclusion of these exposures as potential confounders is imperative when investigating the association between exposure to AAP and DNA adducts. It is conceivable, therefore, that the next generation of biomarker studies in relation to AAP could and should address confounding in a more systematic way (e.g., by measuring cotinine as a more accurate reflection of exposure to tobacco). In contrast, almost all studies assessing DNA methylation changes, perhaps due to being more recent, have adequately adjusted for a number of clinical and environmental confounders, including smoking. Further highlighting the inadequate adjustment in the DNA adducts reviewed studies, the confounders considered did not address other carcinogenicity pathways such as inflammation and epigenetics. Considering that these are pathways shown to be influenced by AAP (95-97) and are also shown to be implicated in carcinogenicity (96,98), future studies should use in confounder adjustments markers that are relevant to more than one carcinogenicity pathways.
Reversibility of changes and individual susceptibilities
A second issue that arises from the review of the evidence pool on DNA adducts, is the plasticity and reversibility of the biomarker investigated. Whereas protein adducts cannot be repaired and thus better reflect exposure, DNA adducts can be eliminated by DNA repair mechanisms and are therefore more transient indicators of external exposure. DNA methylation changes have also been shown to be reversible. In addition, other markers of AAP exposure have differential response and step transition times varying at each step with half-lives counted in hours for e.g., 1-hydroxypyrene (1-OHP), oxidized nucleobases, and gene expression, whereas bulky adducts show half-lives of weeks and for chromosomal aberrations (CAs) and micronucei the half-life can be years (95). Hence timing of exposure and the kinetics of the carcinogen and biomarker need to be incorporated in the design of future studies.
In addition, one needs to consider inherited and acquired individual susceptibilities, as DNA adduct levels have been found to be dependent on polymorphisms in metabolic genes involved in adduct formation and DNA repair (i.e., the CYP1A1, MspI, and GSTM1 null genotypes, the XRCC3-241Met homozygote variant allele, and the XPD-Lys751Gln polymorphism with at least 1 variant allele) (99-103). Recently, it was demonstrated the even mitochondrial genetic background can modify the relationship between AAP and biomarkers of inflammation, because of the role of mitochondria in reactive oxygen species production (104). Thus, individual susceptibility can influence different carcinogenicity pathways in different and multi-faceted ways, highlighting the importance of its inclusion in such investigations.
Intensity, duration, and timing of exposure
Furthermore, the issues of intensity, duration, and timing of exposure are of primary importance when evaluating the impact of AAP. As previously discussed, studies show that developing fetuses are more susceptible than adults to the carcinogenic effects of PAHs (44,62,68). Exposure at this critical developmental stage may cause subtle changes that may or may not be repaired. If not repaired, these changes can persist and lead to increased risk of dysfunction and disease later in life (105). Similarly, timing of exposure can be of relevance to other air pollution carcinogenicity biomarkers. For example, exposure to coal and wood smoke after the age of 20 was shown to reduce global DNA methylation levels, but exposure before 20 years was not associated with methylation changes (106).
Studies also show that exposure to PAHs and DNA adduct formation are not linearly associated (69). Instead, among highly exposed subjects the level of DNA adducts per unit of exposure was significantly lower than those at lower exposures (70).
There is little evidence in the literature about the impact of duration of exposure on the formation of DNA adducts, since no studies have investigated the impact of short-term exposure on DNA adduct formation. However, with respect to mortality, it has been shown that short-term exposure-mortality associations were substantially lower than equivalent long-term associations, a finding which suggests larger, more persistent cumulative effects from long-term exposures (107).
Lastly, the exact composition of AAP exposures needs to be defined in future studies. According to a recently published study, the size fraction of the particles in air are likely to affect different pathophysiological pathways independently (3), therefore AAP exposures with different fractions of differently sized particles might have different biological effects.
In order to add biological credibility and certainty to the impact assessment of AAP, future studies need to aim in bridging current gaps in knowledge about the timing of air pollution effects, the influence of duration of exposure, and the persistence of effects.
Target vs. surrogate tissues
Another important consideration is that most biomarker studies available to date use surrogate tissues, such as blood. AAP is more likely to have the largest impact on sites of deposition where doses are highest, such as the upper aerodigestive tract and lung. If DNA and protein adducts are investigated in target tissues, the associations observed are likely to be much stronger, more reliable, and more accurate. Biomarkers that show great potential for the assessment of AAP exposure and respiratory effects are biomarkers in exhaled breath. Such biomarkers include but are not limited to exhaled nitric oxide (FeNO), exhaled breath condensate (EBC) pH, 8-isoprostane, and interleukin 1β (108,109). These exhaled biomarkers of airway oxidative stress and inflammation can provide a more reliable indication of biologically effective dose with respect to respiratory effects than biomarkers in surrogate tissues (109). Biomarkers relevant to other carcinogenicity mechanisms in exhaled breath remain to be identified.
Measurement error and other biases in study design and analysis
Even though the use of biomarkers can improve exposure assessment in future investigations, the studies included in this manuscript point to an overall need for better and more carefully designed studies to assess the carcinogenicity of AAP. The majority of studies reviewed here used measurements from stationaly air pollution monitoring stations or residence/occupation in a heavily polluted city as proxies to personal exposure to AAP. However, future studies should rely more on individual exposures with the use of mobile, individual sensors, as some of the more recent studies have (66,88). In addition, studies focusing on the comparison of means can only account for a limited number of confounding factors (Table 2), introducing bias, and thus more sophisticated statistical analyses should be the preferred in future investigations.
Despite the discussed limitations, DNA adducts and DNA methylation are undeniably valuable biomarkers of exposure and early biological effect regarding AAP. A recent review (95) recognized in addition to DNA adducts and DNA methylation, 1-OHP, CAs, micronuclei (MN), and oxidative damage to nucleobases, as valid biomarkers of exposure to air pollution. These biological markers cover the whole spectrum of progression from external exposure to tumour formation. 1-OHP is an excellent marker of internal dose for PAH exposure, and DNA adducts and oxidized nucleobases are markers of the biologically effective dose, whereas MN, CA, and DNA methylation are good markers of early biological effect (95). DNA adducts and DNA methylation have also been suggested to be predictive for the risk of future cancer (56,98,110,111).
Future directions
Application of DNA adducts and DNA methylation as biomarkers of exposure and early biological effect in large prospective studies on AAP has the potential to reduce measurement error and elucidate possible mechanisms of carcinogenesis. In addition, careful consideration of confounders, use of personal air monitors, investigation of different aetiologically relevant time-windows, and use of target tissues where possible can also improve the quality of future studies thus allowing more weight to be placed on their conclusions. Therefore, high quality prospective population studies can strengthen causality assertions and improve understanding, offering possible avenues through which to combat the problem of AAP carcinogenicity.
The genomics era has led to great improvements in the understanding of cancer biology, and together with the development of high-resolution and high-throughput technologies interrogating other -omics (such as epigenomics, transcriptomics, proteomics, and metabolomics) they have yielded an unprecedented perspective on cancer omics. These technologies and the emerging knowledge can be used to identify even more biomarkers of AAP exposure and carcinogenicity. Such biomarkers will enable elucidation of new and better understanding of existing carcinogenesis pathways, thus advancing research and addressing the aforementioned gaps in knowledge. Key to such investigations is a multidimensional approach which will help put markers from a specific -omic level into the broader, cellular and molecular context.
Lastly, future studies can be of a transitional nature, aiming to bridge the gap between lab experimentation and population based epidemiology. Validation of in vitro results and incorporation of in vitro markers in population studies will also strengthen causality inferences, offering multi-level evidence for the carcinogenicity of AAP and the importance of timing, duration, intensity, reversibility of changes and individual susceptibility.
Conclusions
In conclusion, DNA adducts and DNA methylation are important biomarkers that can be used in the investigation of the relationship between AAP and its carcinogenic effects, as they not only improve exposure assessment but also increase our understanding of mechanisms underlying this association. These biomarkers should be used in properly designed future studies of air pollution carcinogenicity. These studies are needed to address current knowledge gaps which would in turn open avenues for prevention, diagnosis, and treatment of cancers and other diseases resulting from air pollution exposure.
Acknowledgements
We are grateful to Ole Raaschou-Nielsen and the reviewers for their thoughtful comments which have helped improve this manuscript.
Funding: Funded with the “Exposomics” grant to PV (Enhanced exposure assessment and omic profiling for high priority environmental exposures in Europe. European Commission FP7 Grant agreement no: 308610). CAD was supported by the European Union [EU-Europeaid grant CRIS 2009/223-507 and the European Union’s Seventh Framework Programme (FP7/2007-2013) under Grant Agreement No 288328].
Disclosure: The authors declare no conflict of interest.
References
- World Health Organization. Ambient (outdoor) air quality and health. Available online: http://www.who.int/mediacentre/factsheets/fs313/en/. Accessed Sep 25, 2014.
- World Health Organization. Ambient air pollution. Available online: http://www.who.int/gho/phe/outdoor_air_pollution/en/. Accessed Sep 25, 2014.
- Gong J, Zhu T, Kipen H, et al. Comparisons of ultrafine and fine particles in their associations with biomarkers reflecting physiological pathways. Environ Sci Technol 2014;48:5264-73. [PubMed]
- World Health Organization. Household (Indoor) Air Pollution. Available online: http://www.who.int/indoorair/en/. Accessed Sept 25, 2014.
- Cohen AJ, Anderson HR, Ostro B, et al. Urban air pollution. In: Ezzati M, Lopez A, Rodgers A, et al. eds. Comparative quantification of health risks: global and regional burden of disease attributable to selected major risk factors. Geneva: World Health Organization, 2004:1353-433.
- Pope CA 3rd, Burnett RT, Turner MC, et al. Lung cancer and cardiovascular disease mortality associated with ambient air pollution and cigarette smoke: shape of the exposure-response relationships. Environ Health Perspect 2011;119:1616-21. [PubMed]
- Straif K, Cohen A, Samet J. eds. Air Pollution and Cancer: IARC Scientific Publication No. 161, 1st ed. Lyon, France: International Agency for Research on Cancer, 2013. Available online: http://www.iarc.fr/en/publications/books/sp161/index.php. Accessed Nov 25, 2013.
- Committee on the Medical Effects of Air Pollutants. Long-Term Exposure to Air Pollution: Effect on Mortality. A Report by the Committee on the Medical Effects of Air Pollutants. Chilton, Oxfordshire, UK: Health Protection Agency, 2009. Available online: https://www.gov.uk/government/uploads/system/uploads/attachment_data/file/304667/COMEAP_long_term_exposure_to_air_pollution.pdf
- Buell P, Dunn JE Jr, Breslow L. Cancer of the lung and Los-Angeles-type air pollution. Prospective study. Cancer 1967;20:2139-47. [PubMed]
- Mills PK, Abbey D, Beeson WL, et al. Ambient air pollution and cancer in California Seventh-day Adventists. Arch Environ Health 1991;46:271-80. [PubMed]
- Beeson WL, Abbey DE, Knutsen SF. Long-term concentrations of ambient air pollutants and incident lung cancer in California adults: results from the AHSMOG study.Adventist Health Study on Smog. Environ Health Perspect 1998;106:813-22. [PubMed]
- Abbey DE, Nishino N, McDonnell WF, et al. Long-term inhalable particles and other air pollutants related to mortality in nonsmokers. Am J Respir Crit Care Med 1999;159:373-82. [PubMed]
- McDonnell WF, Nishino-Ishikawa N, Petersen FF, et al. Relationships of mortality with the fine and coarse fractions of long-term ambient PM10 concentrations in nonsmokers. J Expo Anal Environ Epidemiol 2000;10:427-36. [PubMed]
- Pope CA 3rd, Burnett RT, Thun MJ, et al. Lung cancer, cardiopulmonary mortality, and long-term exposure to fine particulate air pollution. JAMA 2002;287:1132-41. [PubMed]
- Jerrett M, Burnett RT, Ma R, et al. Spatial analysis of air pollution and mortality in Los Angeles. Epidemiology 2005;16:727-36. [PubMed]
- Turner MC, Krewski D, Pope CA 3rd, et al. Long-term ambient fine particulate matter air pollution and lung cancer in a large cohort of never-smokers. Am J Respir Crit Care Med 2011;184:1374-81. [PubMed]
- Dockery DW, Pope CA 3rd, Xu X, et al. An association between air pollution and mortality in six U.S. cities. N Engl J Med 1993;329:1753-9. [PubMed]
- Krewski D, Burnett RT, Goldberg M, et al. Reanalysis of the Harvard Six Cities Study, part I: validation and replication. Inhal Toxicol 2005;17:335-42. [PubMed]
- Laden F, Schwartz J, Speizer FE, et al. Reduction in fine particulate air pollution and mortality: Extended follow-up of the Harvard Six Cities study. Am J Respir Crit Care Med 2006;173:667-72. [PubMed]
- Puett RC, Hart JE, Yanosky JD, et al. Particulate matter air pollution exposure, distance to road, and incident lung cancer in the nurses' health study cohort. Environ Health Perspect 2014;122:926-32. [PubMed]
- Nafstad P, Håheim LL, Oftedal B, et al. Lung cancer and air pollution: a 27 year follow up of 16 209 Norwegian men. Thorax 2003;58:1071-6. [PubMed]
- Filleul L, Rondeau V, Vandentorren S, et al. Twenty five year mortality and air pollution: results from the French PAARC survey. Occup Environ Med 2005;62:453-60. [PubMed]
- Vineis P, Hoek G, Krzyzanowski M, et al. Air pollution and risk of lung cancer in a prospective study in Europe. Int J Cancer 2006;119:169-74. [PubMed]
- Beelen R, Hoek G, van den Brandt PA, et al. Long-term exposure to traffic-related air pollution and lung cancer risk. Epidemiology 2008;19:702-10. [PubMed]
- Brunekreef B, Beelen R, Hoek G, et al. Effects of long-term exposure to traffic-related air pollution on respiratory and cardiovascular mortality in the Netherlands: the NLCS-AIR study. Res Rep Health Eff Inst 2009;(139):5-71; discussion 73-89. [PubMed]
- Raaschou-Nielsen O, Andersen ZJ, Hvidberg M, et al. Lung cancer incidence and long-term exposure to air pollution from traffic. Environ Health Perspect 2011;119:860-5. [PubMed]
- Raaschou-Nielsen O, Andersen ZJ, Beelen R, et al. Air pollution and lung cancer incidence in 17 European cohorts: prospective analyses from the European Study of Cohorts for Air Pollution Effects (ESCAPE). Lancet Oncol 2013;14:813-22. [PubMed]
- Raaschou-Nielsen O, Bak H, Sørensen M, et al. Air pollution from traffic and risk for lung cancer in three Danish cohorts. Cancer Epidemiol Biomarkers Prev 2010;19:1284-91. [PubMed]
- Pope CA 3rd, Thun MJ, Namboodiri MM, et al. Particulate air pollution as a predictor of mortality in a prospective study of U.S. adults. Am J Respir Crit Care Med 1995;151:669-74. [PubMed]
- Yorifuji T, Kashima S, Tsuda T, et al. Long-term exposure to traffic-related air pollution and mortality in Shizuoka, Japan. Occup Environ Med 2010;67:111-7. [PubMed]
- Katanoda K, Sobue T, Satoh H, et al. An association between long-term exposure to ambient air pollution and mortality from lung cancer and respiratory diseases in Japan. J Epidemiol 2011;21:132-43. [PubMed]
- Hales S, Blakely T, Woodward A. Air pollution and mortality in New Zealand: cohort study. J Epidemiol Community Health 2012;66:468-73. [PubMed]
- Carey IM, Atkinson RW, Kent AJ, et al. Mortality associations with long-term exposure to outdoor air pollution in a national English cohort. Am J Respir Crit Care Med 2013;187:1226-33. [PubMed]
- Cesaroni G, Badaloni C, Gariazzo C, et al. Long-term exposure to urban air pollution and mortality in a cohort of more than a million adults in Rome. Environ Health Perspect 2013;121:324-31. [PubMed]
- Heinrich J, Thiering E, Rzehak P, et al. Long-term exposure to NO2 and PM10 and all-cause and cause-specific mortality in a prospective cohort of women. Occup Environ Med 2013;70:179-86. [PubMed]
- Yorifuji T, Kashima S, Tsuda T, et al. Long-term exposure to traffic-related air pollution and the risk of death from hemorrhagic stroke and lung cancer in Shizuoka, Japan. Sci Total Environ 2013;443:397-402. [PubMed]
- Loomis D, Grosse Y, Lauby-Secretan B, et al. The carcinogenicity of outdoor air pollution. Lancet Oncol 2013;14:1262-3. [PubMed]
- Hecht SS. Carcinogen Metabolites as Biomarkers. In: Wild C, Vineis P, Garte S. eds. Molecular Epidemiology of Chronic Diseases, 1st ed. New York: Wiley, 2008:97-110.
- Perera F, Brenner D, Jeffrey A, et al. DNA adducts and related biomarkers in populations exposed to environmental carcinogens. Environ Health Perspect 1992;98:133-7. [PubMed]
- Hemminki K, Zhang LF, Krüger J, et al. Exposure of bus and taxi drivers to urban air pollutants as measured by DNA and protein adducts. Toxicol Lett 1994;72:171-4. [PubMed]
- Nielsen PS, de Pater N, Okkels H, et al. Environmental air pollution and DNA adducts in Copenhagen bus drivers--effect of GSTM1 and NAT2 genotypes on adduct levels. Carcinogenesis 1996;17:1021-7. [PubMed]
- Nielsen PS, Okkels H, Sigsgaard T, et al. Exposure to urban and rural air pollution: DNA and protein adducts and effect of glutathione-S-transferase genotype on adduct levels. Int Arch Occup Environ Health 1996;68:170-6. [PubMed]
- Yang K, Airoldi L, Pastorelli R, et al. Aromatic DNA adducts in lymphocytes of humans working at high and low traffic density areas. Chem Biol Interact 1996;101:127-36. [PubMed]
- Topinka J, Binková B, Mracková G, et al. DNA adducts in human placenta as related to air pollution and to GSTM1 genotype. Mutat Res 1997;390:59-68. [PubMed]
- Merlo F, Bolognesi C, Peluso M, et al. Airborne levels of polycyclic aromatic hydrocarbons: 32P-postlabeling DNA adducts and micronuclei in white blood cells from traffic police workers and urban residents. J Environ Pathol Toxicol Oncol 1997;16:157-62. [PubMed]
- Georgiadis P, Topinka J, Stoikidou M, et al. Biomarkers of genotoxicity of air pollution (the AULIS project): bulky DNA adducts in subjects with moderate to low exposures to airborne polycyclic aromatic hydrocarbons and their relationship to environmental tobacco smoke and other parameters. Carcinogenesis 2001;22:1447-57. [PubMed]
- Ruchirawa M, Mahidol C, Tangjarukij C, et al. Exposure to genotoxins present in ambient air in Bangkok, Thailand--particle associated polycyclic aromatic hydrocarbons and biomarkers. Sci Total Environ 2002;287:121-32. [PubMed]
- Marczynski B, Preuss R, Mensing T, et al. Genotoxic risk assessment in white blood cells of occupationally exposed workers before and after alteration of the polycyclic aromatic hydrocarbon (PAH) profile in the production material: comparison with PAH air and urinary metabolite levels. Int Arch Occup Environ Health 2005;78:97-108. [PubMed]
- Topinka J, Sevastyanova O, Binkova B, et al. Biomarkers of air pollution exposure--a study of policemen in Prague. Mutat Res 2007;624:9-17. [PubMed]
- Tuntawiroon J, Mahidol C, Navasumrit P, et al. Increased health risk in Bangkok children exposed to polycyclic aromatic hydrocarbons from traffic-related sources. Carcinogenesis 2007;28:816-22. [PubMed]
- Ayi-Fanou L, Avogbe PH, Fayomi B, et al. DNA-adducts in subjects exposed to urban air pollution by benzene and polycyclic aromatic hydrocarbons (PAHs) in Cotonou, Benin. Environ Toxicol 2011;26:93-102. [PubMed]
- Binková B, Lewtas J, Misková I, et al. DNA adducts and personal air monitoring of carcinogenic polycyclic aromatic hydrocarbons in an environmentally exposed population. Carcinogenesis 1995;16:1037-46. [PubMed]
- Whyatt RM, Santella RM, Jedrychowski W, et al. Relationship between ambient air pollution and DNA damage in Polish mothers and newborns. Environ Health Perspect 1998;106 Suppl 3:821-6. [PubMed]
- Sørensen M, Autrup H, Hertel O, et al. Personal exposure to PM2.5 and biomarkers of DNA damage. Cancer Epidemiol Biomarkers Prev 2003;12:191-6. [PubMed]
- Castaño-Vinyals G, D'Errico A, Malats N, et al. Biomarkers of exposure to polycyclic aromatic hydrocarbons from environmental air pollution. Occup Environ Med 2004;61:e12. [PubMed]
- Peluso M, Munnia A, Palli D, et al. Bulky DNA adducts and lung cancer risk: a prospective study in EPIC investigation. AACR Meeting Abstracts 2005;2005:512-a.
- Neri M, Ugolini D, Bonassi S, et al. Children's exposure to environmental pollutants and biomarkers of genetic damage. II. Results of a comprehensive literature search and meta-analysis. Mutat Res 2006;612:14-39. [PubMed]
- Pavanello S, Pulliero A, Saia BO, et al. Determinants of anti-benzo[a]pyrene diol epoxide-DNA adduct formation in lymphomonocytes of the general population. Mutat Res 2006;611:54-63. [PubMed]
- Palli D, Saieva C, Munnia A, et al. DNA adducts and PM(10) exposure in traffic-exposed workers and urban residents from the EPIC-Florence City study. Sci Total Environ 2008;403:105-12. [PubMed]
- Peluso M, Srivatanakul P, Munnia A, et al. DNA adduct formation among workers in a Thai industrial estate and nearby residents. Sci Total Environ 2008;389:283-8. [PubMed]
- Pavanello S, Bollati V, Pesatori AC, et al. Global and gene-specific promoter methylation changes are related to anti-B[a]PDE-DNA adduct levels and influence micronuclei levels in polycyclic aromatic hydrocarbon-exposed individuals. Int J Cancer 2009;125:1692-7. [PubMed]
- Pedersen M, Wichmann J, Autrup H, et al. Increased micronuclei and bulky DNA adducts in cord blood after maternal exposures to traffic-related air pollution. Environ Res 2009;109:1012-20. [PubMed]
- Eriksen KT, Sørensen M, Autrup H, et al. Lifestyle, environmental, and genetic predictors of bulky DNA adducts in a study population nested within a prospective Danish cohort. J Toxicol Environ Health A 2010;73:583-95. [PubMed]
- García-Suástegui WA, Huerta-Chagoya A, Carrasco-Colín KL, et al. Seasonal variations in the levels of PAH-DNA adducts in young adults living in Mexico City. Mutagenesis 2011;26:385-91. [PubMed]
- Herbstman JB, Tang D, Zhu D, et al. Prenatal exposure to polycyclic aromatic hydrocarbons, benzo[a]pyrene-DNA adducts, and genomic DNA methylation in cord blood. Environ Health Perspect 2012;120:733-8. [PubMed]
- Rossner P Jr, Svecova V, Schmuczerova J, et al. Analysis of biomarkers in a Czech population exposed to heavy air pollution. Part I: bulky DNA adducts. Mutagenesis 2013;28:89-95. [PubMed]
- Yang K, Airoldi L, Pastorelli R, et al. Aromatic DNA adducts in lymphocytes of humans working at high and low traffic density areas. Chem Biol Interact 1996;101:127-36. [PubMed]
- Perera FP, Tang D, Tu YH, et al. Biomarkers in maternal and newborn blood indicate heightened fetal susceptibility to procarcinogenic DNA damage. Environ Health Perspect 2004;112:1133-6. [PubMed]
- Lewtas J, Walsh D, Williams R, et al. Air pollution exposure-DNA adduct dosimetry in humans and rodents: evidence for non-linearity at high doses. Mutat Res 1997;378:51-63. [PubMed]
- Peluso M, Ceppi M, Munnia A, et al. Analysis of 13 (32)P-DNA postlabeling studies on occupational cohorts exposed to air pollution. Am J Epidemiol 2001;153:546-58. [PubMed]
- Lutz WK. Dose-response relationship and low dose extrapolation in chemical carcinogenesis. Carcinogenesis 1990;11:1243-7. [PubMed]
- Garte S, Zocchetti C, Taioli E. Gene--environment interactions in the application of biomarkers of cancer susceptibility in epidemiology. IARC Sci Publ 1997;251-64. [PubMed]
- Jovanovic J, Rønneberg JA, Tost J, et al. The epigenetics of breast cancer. Mol Oncol 2010;4:242-54. [PubMed]
- Pfeifer GP, Besaratinia A. Mutational spectra of human cancer. Hum Genet 2009;125:493-506. [PubMed]
- De Smet C, Loriot A, Boon T. Promoter-dependent mechanism leading to selective hypomethylation within the 5' region of gene MAGE-A1 in tumor cells. Mol Cell Biol 2004;24:4781-90. [PubMed]
- Stearns V, Zhou Q, Davidson NE. Epigenetic regulation as a new target for breast cancer therapy. Cancer Invest 2007;25:659-65. [PubMed]
- Brennan K, Flanagan JM. Is there a link between genome-wide hypomethylation in blood and cancer risk? Cancer Prev Res (Phila) 2012;5:1345-57. [PubMed]
- Veeck J, Esteller M. Breast cancer epigenetics: from DNA methylation to microRNAs. J Mammary Gland Biol Neoplasia 2010;15:5-17. [PubMed]
- Ehrlich M. DNA methylation in cancer: too much, but also too little. Oncogene 2002;21:5400-13. [PubMed]
- Garrick D, Fiering S, Martin DI, et al. Repeat-induced gene silencing in mammals. Nat Genet 1998;18:56-9. [PubMed]
- Nguyen C, Liang G, Nguyen TT, et al. Susceptibility of nonpromoter CpG islands to de novo methylation in normal and neoplastic cells. J Natl Cancer Inst 2001;93:1465-72. [PubMed]
- Baccarelli A, Wright RO, Bollati V, et al. Genomic DNA methylation, cardiovascular disease, and short-term exposure to traffic air pollution. Epidemiology 2008;19:S141-2.
- Baccarelli A, Wright RO, Bollati V, et al. Rapid DNA methylation changes after exposure to traffic particles. Am J Respir Crit Care Med 2009;179:572-8. [PubMed]
- Tarantini L, Bonzini M, Apostoli P, et al. Effects of particulate matter on genomic DNA methylation content and iNOS promoter methylation. Environ Health Perspect 2009;117:217-22. [PubMed]
- Madrigano J, Baccarelli A, Mittleman MA, et al. Prolonged exposure to particulate pollution, genes associated with glutathione pathways, and DNA methylation in a cohort of older men. Environ Health Perspect 2011;119:977-82. [PubMed]
- De Prins S, Koppen G, Jacobs G, et al. Influence of ambient air pollution on global DNA methylation in healthy adults: a seasonal follow-up. Environ Int 2013;59:418-24. [PubMed]
- Bellavia A, Urch B, Speck M, et al. DNA hypomethylation, ambient particulate matter, and increased blood pressure: findings from controlled human exposure experiments. J Am Heart Assoc 2013;2:e000212. [PubMed]
- Guo L, Byun HM, Zhong J, et al. Effects of short-term exposure to inhalable particulate matter on DNA methylation of tandem repeats. Environ Mol Mutagen 2014;55:322-35. [PubMed]
- Janssen BG, Godderis L, Pieters N, et al. Placental DNA hypomethylation in association with particulate air pollution in early life. Part Fibre Toxicol 2013;10:22. [PubMed]
- Rossnerova A, Tulupova E, Tabashidze N, et al. Factors affecting the 27K DNA methylation pattern in asthmatic and healthy children from locations with various environments. Mutat Res 2013;741-742:18-26. [PubMed]
- Palli D, Vineis P, Russo A, et al. Diet, metabolic polymorphisms and dna adducts: the EPIC-Italy cross-sectional study. Int J Cancer 2000;87:444-51. [PubMed]
- Rothman N, Poirier MC, Haas RA, et al. Association of PAH-DNA adducts in peripheral white blood cells with dietary exposure to polyaromatic hydrocarbons. Environ Health Perspect 1993;99:265-7. [PubMed]
- Wiencke JK. DNA adduct burden and tobacco carcinogenesis. Oncogene 2002;21:7376-91. [PubMed]
- Padilla CM, Kihal-Talantikite W, Vieira VM, et al. Air quality and social deprivation in four French metropolitan areas-A localized spatio-temporal environmental inequality analysis. Environ Res 2014;134:315-24. [PubMed]
- Demetriou CA, Raaschou-Nielsen O, Loft S, et al. Biomarkers of ambient air pollution and lung cancer: a systematic review. Occup Environ Med 2012;69:619-27. [PubMed]
- Borm PJ, Schins RP, Albrecht C. Inhaled particles and lung cancer, part B: paradigms and risk assessment. Int J Cancer 2004;110:3-14. [PubMed]
- Alexeeff SE, Coull BA, Gryparis A, et al. Medium-term exposure to traffic-related air pollution and markers of inflammation and endothelial function. Environ Health Perspect 2011;119:481-6. [PubMed]
- Esteller M. Epigenetics in cancer. N Engl J Med 2008;358:1148-59. [PubMed]
- Shields PG, Bowman ED, Harrington AM, et al. Polycyclic aromatic hydrocarbon-DNA adducts in human lung and cancer susceptibility genes. Cancer Res 1993;53:3486-92. [PubMed]
- Ryberg D, Skaug V, Hewer A, et al. Genotypes of glutathione transferase M1 and P1 and their significance for lung DNA adduct levels and cancer risk. Carcinogenesis 1997;18:1285-9. [PubMed]
- Pastorelli R, Guanci M, Cerri A, et al. Impact of inherited polymorphisms in glutathione S-transferase M1, microsomal epoxide hydrolase, cytochrome P450 enzymes on DNA, and blood protein adducts of benzo(a)pyrene-diolepoxide. Cancer Epidemiol Biomarkers Prev 1998;7:703-9. [PubMed]
- Matullo G, Guarrera S, Carturan S, et al. DNA repair gene polymorphisms, bulky DNA adducts in white blood cells and bladder cancer in a case-control study. Int J Cancer 2001;92:562-7. [PubMed]
- Palli D, Russo A, Masala G, et al. DNA adduct levels and DNA repair polymorphisms in traffic-exposed workers and a general population sample. Int J Cancer 2001;94:121-7. [PubMed]
- Wittkopp S, Staimer N, Tjoa T, et al. Mitochondrial genetic background modifies the relationship between traffic-related air pollution exposure and systemic biomarkers of inflammation. PLoS One 2013;8:e64444. [PubMed]
- Barouki R, Gluckman PD, Grandjean P, et al. Developmental origins of non-communicable disease: implications for research and public health. Environ Health 2012;11:42. [PubMed]
- Tao MH, Zhou J, Rialdi AP, et al. Indoor air pollution from solid fuels and peripheral Blood DNA methylation: Findings from a population study in Warsaw, Poland. Environ Res 2014;134:325-30. [PubMed]
- Beverland IJ, Cohen GR, Heal MR, et al. A comparison of short-term and long-term air pollution exposure associations with mortality in two cohorts in Scotland. Environ Health Perspect 2012;120:1280-5. [PubMed]
- Patel MM, Chillrud SN, Deepti KC, et al. Traffic-related air pollutants and exhaled markers of airway inflammation and oxidative stress in New York City adolescents. Environ Res 2013;121:71-8. [PubMed]
- De Prins S, Dons E, Van Poppel M, et al. Airway oxidative stress and inflammation markers in exhaled breath from children are linked with exposure to black carbon. Environ Int 2014;73:440-6. [PubMed]
- Veglia F, Loft S, Matullo G, et al. DNA adducts and cancer risk in prospective studies: a pooled analysis and a meta-analysis. Carcinogenesis 2008;29:932-6. [PubMed]
- Bak H, Autrup H, Thomsen BL, et al. Bulky DNA adducts as risk indicator of lung cancer in a Danish case-cohort study. Int J Cancer 2006;118:1618-22. [PubMed]