Preoperative lung surface localization for pulmonary wedge resection: a single-center experience
Introduction
Recent advances in computed tomography (CT) screening mean that many small lung tumors can be detected at early stages (1,2). Although early detection of small lung nodules improves the prognosis (1), thoracic surgeons sometimes find it challenging to localize small pulmonary nodules intraoperatively (3,4).
To overcome the difficulties encountered in the surgical resection of tiny pulmonary nodules, we conducted preoperative CT-guided percutaneous marking until 2016, as reported previously (5). However, due to concerns regarding fatal complications such as air embolism (6-8), we recently started using a preoperative marking technique known as virtual-assisted lung mapping (VAL-MAP), which is a novel bronchoscopic dye marking technique (9-12). VAL-MAP comprises multi-spot dye marking performed under virtual bronchoscopy (9-14). A prospective multi-center trial showed that VAL-MAP has reproducible efficacy (13). However, another prospective multi-center study reported that about 12% of sublobar lung resections performed using VAL-MAP failed to acquire adequate resection margins larger than the tumor diameter or 2 cm (14). As VAL-MAP is a lung surface marking technique, the deep resection margin is the most significant factor affecting successful resection with sufficient resection margins.
The present study evaluated the efficacy and limitations of two lung surface marking techniques: CT-guided percutaneous marking and VAL-MAP. We analyzed the surgical outcomes of both procedures and evaluated the risk factors for resection failure.
Methods
Patients and main endpoint
The present single-center retrospective study included patients who underwent CT-guided percutaneous needle marking (CT-guided localization) or VAL-MAP from October 1998 to April 2018 at The University of Tokyo Hospital. The inclusion criteria were patients who underwent pulmonary wedge resection with curative intent for malignant lung tumors after a preoperative localization procedure. The exclusion criterion was missing data. Both procedures were indicated for patients with pulmonary nodules that were challenging to identify intraoperatively.
The primary endpoint was the rate of successful resection. Successful resection was defined as complete resection of target tumors with resection margins larger than or equal to the lesion diameter, or ≥2 cm if the tumor was larger than 2 cm (14). Unsuccessful resection was defined as either resection with insufficient margins or the performance of additional resection to obtain adequate margins to achieve curative wedge resection. The successful resection rate was calculated as the number of successfully resected nodules divided by the total number of nodules resected.
CT-guided percutaneous needle marking procedure
CT-guided localization was performed as previously described (5). In brief, a 21-gauge hookwire marker (Hakko Medical, Tokyo, Japan) was placed near the nodule under CT guidance after administration of local anesthesia by board-certificated radiologists on the day of surgery. Another chest CT scan was performed immediately after the procedure to confirm that the marker was appropriately placed.
VAL-MAP procedure
The VAL-MAP procedure was conducted on the day of surgery or 1–2 days before surgery as previously described (9,13). While the patient was under local anesthesia and mild sedation, clinicians performed the bronchoscopic procedure using a bronchoscope (BF-260F or BF-P260F; Olympus, Tokyo, Japan). Using virtual bronchoscopic images, a metal-tipped catheter (PW-6C-1; Olympus) preloaded with 1 mL of indigo carmine was inserted through the working channel of the bronchoscope into the target bronchus. Clinicians injected indigo carmine into the target bronchus after confirmation of the appropriate location of the catheter tip under X-ray fluoroscopy. This procedure was repeated multiple times for multiple markings. After the dye injections, a chest CT scan was performed to reconstruct three-dimensional images that showed the actual locations of the markings and the target nodules.
Data collection
All hospital records were retrospectively reviewed. The patients’ demographic data and main outcomes were assessed and confirmed by board-certified surgeons (K Nagayama, T Yoshioka, T Yotsumoto, and KJ Fukumoto). Two board-certificated surgeons (K Nagayama and S Kawashima) assessed the resection margins based on either the operative notes or pathological reports.
Statistical analysis
Continuous variables are reported as mean and standard deviation, while categorical variables are reported as frequency and percentage. The two-sample t-test and the chi-squared test were used to compare the frequencies of continuous and categorical variables, respectively.
Logistic regression analyses were conducted to assess the factors associated with successful resection, considering the preoperative marking technique, characteristics of target nodules, and intraoperative adhesion (15,16). The required resection depth was also assessed, as the successful resection rate reportedly decreases in tandem with increased required resection depth (14). The required resection depth was defined as the minimum depth of the resection line required to achieve successful resection and was calculated as: Required resection depth (mm) = depth (distance from the closest pleura) + {[lesion size × 2 (tumor <2 cm)] or [lesion size + 20 (tumor ≥2 cm)]} (14).
The receiver operating characteristics (ROCs) curve was plotted to determine the optimal cutoff point of the required resection depth for the prediction of successful resection. The ROC curve was obtained by calculating the sensitivity and specificity at every possible cutoff point. The optimal cutoff point was determined as the point that was closest to the upper left-hand corner (17).
All statistical analyses were performed with EZR (Saitama Medical Center, Jichi Medical University, Saitama, Japan, 2012) (18). The level of significance was set at P<0.05.
Ethical issues
This observational study was approved by the Ethics Committee of The University of Tokyo (approval number 2406-5). The requirement for written informed consent was waived because of the retrospective nature of this chart review.
Results
Patient and lesion characteristics
CT-guided localization was performed in 85 patients from October 1998 to July 2016, whereas VAL-MAP was performed in 130 patients from January 2014 to April 2018. Of these, 119 patients with 153 nodules met the eligibility criteria (Figure 1). The characteristics of the patients and resected nodules in the CT-guided localization group and VAL-MAP group were summarized in Table 1. The two groups significantly differed regarding patient age, lesion size, required resection depth, appearance of nodules, and pathological diagnosis (Table 1).
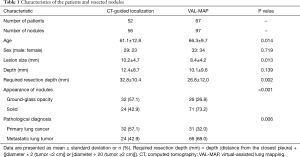
Full table
Factors affecting successful resection
Univariate analysis revealed that the VAL-MAP procedure was significantly associated with successful resection [odds ratio (OR): 2.72, 95% confidence interval (CI): 1.02–7.24; P=0.045], while the required resection depth (OR: 0.50, 95% CI: 0.33–0.77; P=0.002) and intraoperative adhesion (OR: 0.30, 95% CI: 0.11–0.83; P=0.020) were significantly related to unsuccessful resection (Table 2). Multivariate analysis revealed that the required resection depth was the only significant independent risk factor for unsuccessful resection (OR: 0.55, 95% CI: 0.35–0.87; P=0.011) (Table 2).
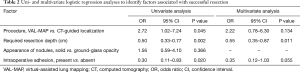
Full table
Optimal cutoff value for the required resection depth
As shown above, the required resection depth was a significant independent predictive factor for successful resection. The ROC curve identified the optimal cutoff value of the required resection depth for successful resection as 31 mm (Figure 2). This cutoff value had a sensitivity of 65.7% (95% CI: 57.0–73.7), specificity of 73.7% (95% CI: 48.8–90.9), positive predictive value of 94.6% (95% CI: 87.9–98.2), and negative predictive value of 23.3% (95% CI: 13.4–36.0).
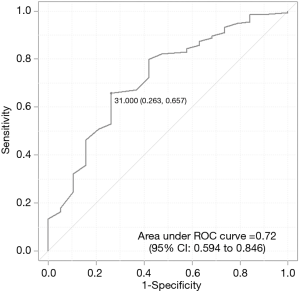
Successful resection rate in accordance with the localization procedure, intraoperative adhesion, and required resection depth
In the overall cohort, the successful resection rate was 87.6% (134 of 153 lesions). Although the successful resection rate did not significantly differ between the two groups, VAL-MAP tended to result in a higher successful resection rate than CT-guided localization (91.8% vs. 80.4%; P=0.071) (Figure 3A). The successful resection rate was significantly worse in the presence of adhesion than in the absence of adhesion (75.0% vs. 90.1%; P=0.034) (Figure 3B). The successful resection rate was significantly worse for nodules with a greater required resection depth (>31 mm) than those with a smaller required resection depth (≤31 mm) (76.7% vs. 94.6%; P=0.002) (Figure 3C).
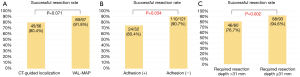
Post-hoc analysis of the effects of intraoperative adhesion and required resection depth on the successful resection rate for each localization procedure
The presence of intraoperative adhesion and greater required resection depth (>31 mm) were significant negative factors affecting successful resection (Table 2).
In the CT-guided localization group, the successful resection rate was significantly worse for nodules with versus without intraoperative adhesion (54.5% vs. 86.7%; P=0.048; Figure 4A). In the VAL-MAP group, the successful resection rate did not significantly differ for nodules with versus without adhesion (85.7% vs. 93.4%; P=0.491; Figure 4B).
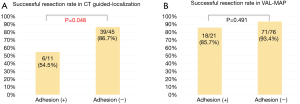
Although the differences were not significant, the successful resection rate for nodules with a greater required resection depth (>31 mm) tended to be worse than that for those with a smaller required resection depth in both the CT-guided localization group (70.0% vs. 92.3%; P=0.079; Figure S1A) and the VAL-MAP group (83.3% vs. 95.5%; P=0.106; Figure S1B).
Discussion
A greater required resection depth was a significant risk factor for resection failure, regardless of preoperative localization technique (Table 2, Figures 3C,S1). As both CT-guided localization and VAL-MAP only enable the placement of markings on the lung surface, uncertainty regarding the deep resection margin has been assumed to be a potential limitation, and the present findings confirmed that this was an actual problem. The present study revealed that a required resection depth of 31 mm may be the threshold value for predicting the success of curative pulmonary wedge resection assisted by lung surface preoperative localization. Our results suggest that care is needed when choosing the surgical approach for nodules that require a resection depth of more than 31 mm, and surgeons should consider selecting segmentectomy or lobectomy instead of wedge resection assisted by a preoperative localization technique.
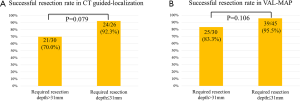
An interesting new approach to overcome the challenge of determining the deep resection margin is to place a fiducial marker in the deep lung parenchyma to indicate the optimal deep resection lines. Our pilot study combining VAL-MAP and bronchoscopic microcoil placement to indicate the deep resection line demonstrated a promising outcome (19), and we are currently evaluating this technique in a prospective multicenter study in Japan.
The present study revealed that intraoperative adhesion negatively affected curative wedge resection of small pulmonary nodules (Table 2, Figure 3B). The negative effects of intraoperative adhesion were especially notable when CT-guided localization was used (Figure 4A), while VAL-MAP was less influenced by intraoperative adhesion (Figure 4B). This may be because VAL-MAP provides multiple-spot marking and mapping for each nodule, while CT-guided localization only provides one marking for each nodule. Even if one VAL-MAP marking is not visible due to adhesion, the surgeon may be able to use other visible markings to successfully localize the target nodule (7). This advantage of VAL-MAP may have led to the relatively high successful resection rates regardless of the presence of adhesion (Figures 3A,4B).
The present study did not draw a definitive conclusion regarding the difference in the efficacy of CT-guided localization versus VAL-MAP. Although univariate analysis showed a significant association between VAL-MAP and successful resection (Table 2), surgeons tended to use CT-guided localization for nodules with a greater required resection depth (Table 1), and this confounding factor would have contributed to the relatively low successful resection rate (Table 2, Figure 3A). However, considering the relative resistance to intraoperative adhesion (Figure 4B) and the safety profile (15,20,21), we consider VAL-MAP the preferred method for preoperative lung marking for curative wedge resection.
The present study had some limitations. First, it was a small single-institutional retrospective study. Because of the retrospective nature, it would be challenging to compare the two marking techniques appropriately in the present study. Second, the constancy assumption may have been violated, as surgeons’ concerns about the resection margins and the quality of markings would have greatly changed over the decades included in the study period. In our institute, indication of lung surface markings and planned surgical procedure has been preoperatively discussed for each nodule among certificated surgeons. However, actual design of wedge resection considering surgical margin for each nodule has depended on each certificated surgeon. Surgeon’s bias could not be avoided.
In conclusion, intraoperative adhesion and greater required resection depth were limitations of preoperative lung surface localization for curative pulmonary wedge resection. Intraoperative adhesion negatively affected the resection status, especially in CT-guided localization. Considering the relative resistance to intraoperative adhesion, VAL-MAP might be a better preoperative lung surface marking technique than CT-guided localization. Further studies are needed to improve the preoperative localization technique for small lung nodules.
Acknowledgments
We thank Kelly Zammit, BVSc, from Edanz Editing (www.edanzediting.com/ac), for editing a draft of this manuscript.
Funding: This study was supported by the Japan Agency for Medical Research and Development (18ck0106244h0003).
Footnote
Conflicts of Interest: All authors have completed the ICMJE uniform disclosure form (available at http://dx.doi.org/10.21037/jtd.2020.04.43). JN reports grants from Astellas, grants from Taiho Pharma, outside the submitted work. MS serves as an unpaid editorial board member of Journal of Thoracic Disease from Oct 2018 to Sep 2020. The other authors have no conflicts of interest to declare.
Ethical Statement: The authors are accountable for all aspects of the work in ensuring that questions related to the accuracy or integrity of any part of the work are appropriately investigated and resolved. This observational study was approved by the Ethics Committee of The University of Tokyo (approval number 2406-5), and was conducted in compliance with the Declaration of Helsinki.
Open Access Statement: This is an Open Access article distributed in accordance with the Creative Commons Attribution-NonCommercial-NoDerivs 4.0 International License (CC BY-NC-ND 4.0), which permits the non-commercial replication and distribution of the article with the strict proviso that no changes or edits are made and the original work is properly cited (including links to both the formal publication through the relevant DOI and the license). See: https://creativecommons.org/licenses/by-nc-nd/4.0/.
References
- National Lung Screening Trial Research Team, Aberle DR, Adams AM, et al. Reduced lung-cancer mortality with low-dose computed tomographic screening. N Engl J Med 2011;365:395-409. [Crossref] [PubMed]
- Heuvelmans MA, Groen HJ, Oudkerk M. Early lung cancer detection by low-dose CT screening: therapeutic implications. Expert Rev Respir Med 2017;11:89-100. [Crossref] [PubMed]
- Hashimoto H, Yanagiya M, Suzuki Y, et al. A case of solitary pulmonary capillary hemangioma indicating true gross appearance. Pathol Int 2017;67:322-3. [Crossref] [PubMed]
- Nakashima S, Watanabe A, Obama T, et al. Need for preoperative computed tomography-guided localization in video-assisted thoracoscopic surgery pulmonary resections of metastatic pulmonary nodules. Ann Thorac Surg 2010;89:212-8. [Crossref] [PubMed]
- Yoshida Y, Inoh S, Murakawa T, et al. Preoperative localization of small peripheral pulmonary nodules by percutaneous marking under computed tomography guidance. Interact Cardiovasc Thorac Surg 2011;13:25-8. [Crossref] [PubMed]
- Sakiyama S, Kondo K, Matsuoka H, et al. Fatal air embolism during computed tomography-guided pulmonary marking with a hook-type marker. J Thorac Cardiovasc Surg 2003;126:1207-9. [Crossref] [PubMed]
- Horan TA, Pinheiro PM, Araújo LM, et al. Massive gas embolism during pulmonary nodule hook wire localization. Ann Thorac Surg 2002;73:1647-9. [Crossref] [PubMed]
- Yi JH, Choi PJ, Bang JH, et al. Systemic air embolism after computed tomography-guided hook wire localization: two case reports and literature review. J Thorac Dis 2018;10:E59-64. [Crossref] [PubMed]
- Sato M, Omasa M, Chen F, et al. Use of virtual assisted lung mapping (VAL-MAP), a bronchoscopic multispot dye-marking technique using virtual images, for precise navigation of thoracoscopic sublobar lung resection. J Thorac Cardiovasc Surg 2014;147:1813-9. [Crossref] [PubMed]
- Nakao K, Sato M, Nitadori JI, et al. Bilateral segmentectomies using virtual-assisted lung mapping (VAL-MAP) for metastatic lung tumors. Surg Case Rep 2017;3:104. [Crossref] [PubMed]
- Yanagiya M, Sato M, Kuwano H, et al. Management of lung nodules newly found by virtual-assisted lung mapping: a case report. Surg Case Rep 2017;3:49. [Crossref] [PubMed]
- Yanagiya M, Matsumoto J, Yamaguchi H, et al. Effect of patient position during virtual-assisted lung mapping. J Thorac Dis 2019;11:162-70. [Crossref] [PubMed]
- Sato M, Kuwata T, Yamanashi K, et al. Safety and reproducibility of virtual-assisted lung mapping: a multicentre study in Japan. Eur J Cardiothorac Surg 2017;51:861-8. [PubMed]
- Sato M, Kobayashi M, Kojima F, et al. Effect of virtual-assisted lung mapping in acquisition of surgical margins in sublobar lung resection. J Thorac Cardiovasc Surg 2018;156:1691-701.e5. [Crossref] [PubMed]
- Anayama T, Hirohashi K, Miyazaki R, et al. Near-infrared dye marking for thoracoscopic resection of small-sized pulmonary nodules: comparison of percutaneous and bronchoscopic injection techniques. J Cardiothorac Surg 2018;13:5. [Crossref] [PubMed]
- Suzuki K, Nagai K, Yoshida J, et al. Video-assisted thoracoscopic surgery for small indeterminate pulmonary nodules: indications for preoperative marking. Chest 1999;115:563-8. [Crossref] [PubMed]
- Akobeng AK. Understanding diagnostic tests 3: receiver operating characteristic curves. Acta Paediatr 2007;96:644-7. [Crossref] [PubMed]
- Kanda Y. Investigation of the freely available easy-to-use software 'EZR' for medical statistics. Bone Marrow Transplant 2013;48:452-8. [Crossref] [PubMed]
- Sato M, Nagayama K, Kobayashi M, et al. Virtual-assisted lung mapping 2.0: preoperative bronchoscopic three-dimensional lung mapping. Ann Thorac Surg 2019;108:269-73. [Crossref] [PubMed]
- Kuo SW, Tseng YF, Dai KY, et al. Electromagnetic navigation bronchoscopy localization versus percutaneous CT-guided localization for lung resection via video-assisted thoracoscopic surgery: a propensity-matched study. J Clin Med 2019. [Crossref] [PubMed]
- Park CH, Han K, Hur J, et al. Comparative effectiveness and safety of preoperative lung localization for pulmonary nodules: a systematic review and meta-analysis. Chest 2017;151:316-28. [Crossref] [PubMed]