Dosimetric analysis of lymphopenia during chemoradiotherapy for esophageal cancer
Introduction
Esophageal carcinoma accounts for nearly 17,000 annual cancer cases in the United States (450 K worldwide) and portends a very poor prognosis with a 5-year overall survival (OS) rate less than 20% (1). Standard of care treatment for esophageal cancer (EC) treatment in order to optimize chances of a pathological complete response consist of neoadjuvant chemoradiation (CRT) followed by surgical resection (2) or, when unresectable, definitive CRT (3). Treatment related lymphopenia has been associated with a poor prognosis in several solid tumor disease sites including lung, head and neck, pancreatic, breast, and EC (4-8), and has been associated with decreased rates of overall survival (OS), disease free survival (DFS), and distant metastasis free survival (DMFS). It is hypothesized that the relationship between lymphopenia and prognosis may be in large part driven by an associated decrement in cytotoxic T cell activity against neoplastic cells (9).
Lymphocytotoxicity occurs during both chemotherapy and radiation due to relative sensitivity of lymphocytes to DNA damaging agents (10). Radiation exposure alone, independent of chemotherapy, is able to cause lymphopenia (11) and both peripheral circulating lymphocytes, and resident bone marrow lymphocytes are sensitive to radiation therapy (12). Hematologic toxicity (HT) as a result of pelvic bone marrow irradiation has been well described in rectal (13,14) and anal cancer (15). Prospective sparing of the bone marrow during the treatment planning process (16) and use of intensity modulated radiation therapy (IMRT) in anal cancer (17,18) has helped to mitigate this effect.
The cervical and thoracic vertebrae house 20% of functional marrow (19). Several groups have explored the relationship between HT and BM irradiation in patients with non-small cell lung cancer. These studies have demonstrated a dose-dependent association between irradiation of the thoracic vertebral bodies and hematological toxicity (20,21). Additionally, some data suggests that radiation dose to the spleen can also induce lymphopenia (22). This association may be particularly salient in light of recent data establishing adjuvant immunotherapy as standard of care patients treated with CRT for locally advanced NSCLC (23).
Some authors have related hematologic toxicity in EC to bone marrow dose (24), while others have attributed dose to peripheral marrow dose by extrapolating that protons have less dose to circulating cells (7). Given the association between lymphopenia and poor prognosis in EC, we sought to identify potentially modifiable treatment-related factors that contribute to lymphopenia by both evaluating peripheral lymphocytes, dose to bone marrow, and to circulating lymphocytes. Based on the anatomical proximity of the esophagus to the thoracic vertebrae and spleen, along with the extensive radiotherapy treatment fields often employed, we explored a potential association between vertebral body irradiation and splenic volume irradiation during treatment for EC and bone marrow suppression. The primary aim of this study was to examine whether there is an association between vertebral, peripheral, or splenic DVH parameters and grade 4 lymphopenia (G4HL) or lymphocyte nadir. Secondary endpoints included an examination of the impact of radiotherapy technique (IMRT vs. 3DCRT) on BM toxicity in this population as well as whether there is an association between missed chemotherapy and lymphopenia.
Methods
Patients
With investigational review board (IRB) approval, IRB #171972 we retrospectively identified 54 patients with EC treated at our institution from January 2007 through April 2017 for initial review. To be included for further analysis, patients were required to have had at least 4 weeks of available complete blood counts including differential count data. We included patients who were treated with concurrent chemoradiation in either the neoadjuvant (preoperative) setting or definitive setting. Patients receiving standard fractionation with curative intent to the primary site along with concurrent chemotherapy were included while patients treated with palliative intent therapy were excluded. Patients were included if they received induction chemotherapy as long as they had normal blood counts at the start of CRT and had not experienced > grade 2 HT during the induction chemotherapy (n=7). Application of our inclusion and exclusion criteria resulted in a total of 54 patients eligible for analysis.
Blood counts
The absolute lymphocyte counts (ALC) measured as cells ×103/µL were drawn prior to starting CRT and weekly prior to administration of chemotherapy. We used common terminology of adverse events (CTCAE 4.0) which defines grade 4 lymphopenia as a lymphocyte count less than 200 cells/µL.
Radiation dose volumes
We retrospectively contoured the thoracic vertebral bodies of eligible patients. For proximally located tumors (n=7), two vertebral levels above the superior aspect of the planning treatment volume (PTV) were included in the vertebral volume in addition to thoracic vertebral volumes. Based on our population and contouring approach all vertebral volumes contoured were limited to C5 or below. Dose volume histograms (DVH) were evaluated to record the absolute vertebral volume (VVert), in ccs, receiving between 10–40 Gy as well as the mean vertebral dose. We evaluated the splenic volume dosimetric (SV) parameters (in ccs) from volumes between 5–30 Gy along with the mean dose. We also sought to evaluate the effect of radiotherapy dose to the systemic circulating lymphocyte pool by collecting mean body dose (MBD). During collection of MBD we noted the total length of CT simulation scans (and hence relative volume of body captured) was not uniform across all patients due to the retrospective nature of our analysis. In an effort to account for dose to peripheral lymphocytes in a more standardized manner, we performed dosimetric analysis on a volume designed to account for the radiotherapy dose received to the majority of the thoracic blood pool. We termed this volume “standardized thoracic volume (sTV)” and it includes a boolean sum of the contours of the heart to the superior portion of the aortic arch, the entire aortic arch volume, and the entire lungs, while excluding thoracic vertebrae. Mean dose to sTV was calculated and designated by the term mean dose (sTV is displayed in Figure 1). We hypothesized that this volume would capture a majority of cardiac output during daily RT delivery and had the advantage of excluding thoracic vertebral volumes. Radiation was delivered using Intensity modulated therapy (IMRT) or 3-dimensional conformal therapy (3-DCRT). Standard dose constraints to the OARs in the thorax such as those used in RTOG 0436 were followed on all plans (25).
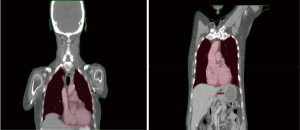
Chemotherapy
Chemotherapy was delivered weekly, concurrently with radiation. Patients primarily received carboplatin (AUC =2) and paclitaxel (50 mg/m2) (n=43), or oxaliplatin (130 mg/m2) with 5-FU (CI 1,000 mg/m2) administered on a weekly basis.
Statistical methods
The primary outcome of this study was to determine whether increasing radiation dose to VVert, SV, MBD, or STV is associated with ALC nadir and G4HL during CRT. Secondary outcomes evaluated the relationship between lymphopenia and missing chemotherapy and assessed the impact of 3DCRT vs. IMRT on lymphopenia as well whether there was an association with lymphocyte count at 3 months. Baseline characteristics were recorded for each variable. The Shapiro-Wilk test was performed to determine if the data was normalized. If it was not it was described as the median with the interquartile range (third quartile minus first quartile). Categorical variables are described as the absolute number of the specified value along with its percentage within the cohort.
Univariable logistic regression models were created to test the correlation between clinical and dosimetric variables with G4HL. Clinical and dosimetric parameters were tested against ALC nadir by creating univariable linear regression models. Due to the challenges of collinearity in an analysis involving multiple dosimetric variables we conducted our analysis in two separate ways. At first, multivariable logistic regression models were generated to include BMI, DM, and one dosimetric parameter predictive of G4HL. We then generated separate multivariable logistic models including both parameters from Vvert and SV (and BMI) if P was less than 0.3 on univariable analysis in order to clearly assess the true contribution of each compartment to lymphopenic reserve.
Interaction testing were performed amongst all dosimetric predictors to assess their effect on the primary endpoint.
Likewise, we generated two separate methods to best analyze collinearity with predictors of ALC nadir identified on multivariable linear regression. Multivariable linear regression model building was comprised of taking univariable P values less than 0.25. Upon fitting these variables, any value which was deemed non-contributory to the model was removed and the subsequent new model was compared to the previous model via the partial likelihood test. If the coefficients (Beta) changed by more than 20% in the parsimonious model, they were added back in to create the final model. In order to avoid multicollinearity only one dosimetric variable was included per model. This was reiterated when appropriate with one predictor from Vvert and SV.
Regression models are expressed as odds ratios (OR) with 95% confidence intervals (CI). OR was obtained as the exponent of the beta coefficient. Probability graphs were created based on the logistic regression model to demonstrate the relationship between volume and probability and G4HL.
Analysis of ROCs using the closest top left method were utilized to determine optimal sensitivity and specificity thresholds for predicting G4HL for a given positive dosimetric variable.
All P values were 2-sided with a level ≤0.05 considered as significant. All statistics and graphs were calculated and created using R software 3.5.0 (Vienna, Austria, http://www.R-project.org).
Results
Patient characteristics
Patient characteristics along with dosimetric descriptive statistics are summarized in Table 1. The majority of patients in this cohort were male (85.3%) with stage III (74%) esophageal carcinoma. Most patient had distally located tumors and had adenocarcinoma histology (77.7%). The majority of patients were treated to a dose of 5,040 cGy with carboplatin/paclitaxel (68.5%) along with 3-Dimensional Conformal Radiation Therapy (57.4%). There were 28 patients (51.8%) of patients that required to have at least one cycle of chemotherapy held. Dosimetric parameters are described in Table 2.
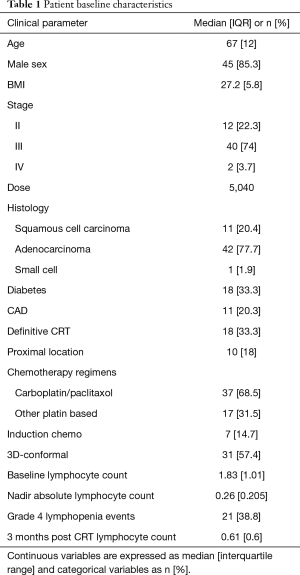
Full table
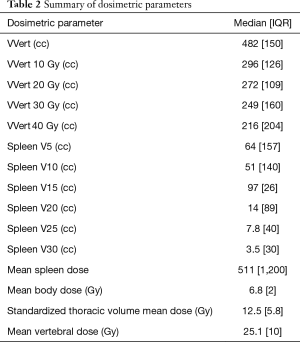
Full table
Predictors for grade 4 lymphopenia
Overall, there were 21 (38.8%) patients who experienced grade 4 lymphopenia during CRT. Table 3 provides a summary of all clinical and dosimetric parameters tested on univariable analysis against G4HL during chemoradiation therapy. Increasing absolute volumes of VVert (per 1 cc) receiving 20 Gy (VV20 cc) and 30 Gy (V30 cc) increased the risk of G4HL on univariable analysis. Spleen DVH values did not correlate with grade 4 lymphopenia nor did mean vertebral dose, mean body dose, and dose to the standardized thoracic volume. Furthermore, planned treatment volume (PTV), location, and utilization of IMRT did not correlate on univariate analysis.
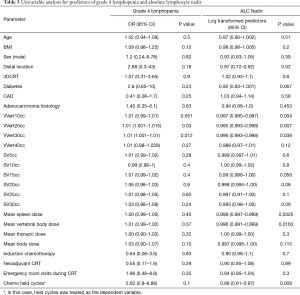
Full table
From these data a multivariate regression model was created in Table 4 accounting for DM, BMI, and the respective individual DVH parameters. In this model, per every 10 cc in the parameters VV10, VV20, and VV30 remained significant predictors of G4HL, with an OR of 1.1 (1.04–1.12; P=0.04) for VV10, 1.1 (1.01–1.14; P=0.03) for VV20, and 1.1 (1.01–1.17; P=0.012) for VV30. In other words, for each 10 cc increase in vertebral BM volume receiving ≥10 Gy, ≥20 Gy, or ≥30 Gy, the risk of G4HL increases by 10%. MBD also correlated in this model with 1.04 (1.00–1.11; P=0.032). A diagnosis of DM or BMI remained not significant when adjusting for DVH parameters.
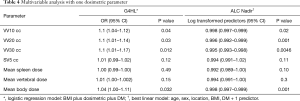
Full table
When the multivariate models were adjusted to account for the doses to the spleen (Table S1), there was no significant changes with what was seen when the models only included one DVH parameter. Essentially, the effects size for VV10, VV20, and VV30 remained significantly the same, OR 1.1, with no single parameter altering the effect size. This further demonstrated the relative effect of marrow dose.
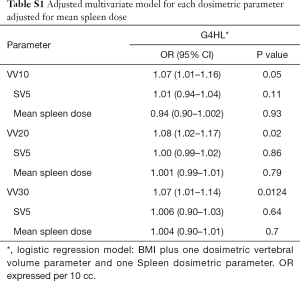
Full table
Graphical representations, generated from the logistic regression equations of the probability of a G4HL are depicted in Figure 2 for VV20 and VV30.
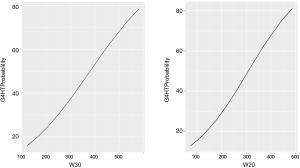
By utilizing the closest top left method for ROC analysis, we found optimal parameters for predicting G4HL were VV10 >289 cc, VV20 >270 cc, VV30 >197 cc. The OR of a G4HL for patients receiving above the specified ROC parameters were 9.4 (P=0.04) and 9.0 (P=0.006) for VV20 and VV30 respectively. Of note, STV was not a significant predictor of G4HL on either univariable analysis or on multivariable regression analysis.
Furthermore, while logistic correlation did not correlation G4HL with missing chemotherapy, patients with G4HL, on chi-squared test were more likely to miss at least a cycle of chemotherapy (P<0.001).
Predictors of lymphocyte Nadir
Initial baseline median lymphocyte count was 1.83 cells ×103/µL, while median lymphocyte count at nadir declined to 0.26 cells ×103/µL (P<0.001). Univariable regression were performed on both lymphocyte nadir and the lymphocyte ratios with Table 3 demonstrating the relationship with the nadir. We found that increasing dose per 10 cc that VV 10 (Beta −0.002, P=0.002), VV20 (Beta −0.004, P=0.003), and VV30 (Beta −0.004, P=0.007) were significantly correlated with absolute lymphocyte nadir. While SV receiving ≥5 Gy was associated with lymphocyte nadir, it is notable that all other spleen DVH parameters examined did not correlate with this endpoint. These results did not significantly change when tested against lymphocyte ratio, with the exception of spleen ≥5 cc no longer retaining significance. On multivariable linear regression, when accounting for age, diabetes, BMI, sex, and location we found that per 10 cc increase VV20 (Beta −0.004, P=0.001), VV30 (Beta −0.005, P=0.0046), and MBD (Beta −0.002, P=0.001) all correlated with absolute lymphocyte nadir. We tested spleen DVH parameters on multivariable regression analysis and did not find a significant correlation.
We tested DVH parameters associated with the sTV, mean vertebral dose and mean body dose. Mean body dose was significantly correlated with lymphocyte nadir on multivariate analysis (Beta −0.002, P=0.008).
In order to adjust for the dose received by the spleen vs. the marrow, we ran another multivariable regression model combining spleen DVH parameters with thoracic bone marrow parameters with the above listed clinical variables and found primarily that the doses received by the bone marrow were most strongly correlated with lymphopenia (Table S1). The odds ratios with respect to bone marrow did not alter considerably in these models.
We furthermore attempted to test whether is an association between dose to the vertebral marrow parameters and lymphocyte nadir at 3 months. While we were unable to find an association on linear regression, we did find that there was a significant difference between the median lymphocyte counts at baseline vs. the median at 3 months (Wilcoxon sign rank test P<0.001). This suggests that there may be a protracted effect of lymphocyte depletion.
Of note, decreasing lymphocyte nadir (measured as an independent variable) was associated with missing at least 1 cycle of chemotherapy (OR 0.88, P=0.009) which suggests for each one unit increase in lymphocyte the odds of not missing a cycle of chemotherapy increases by 12%.
Discussion
Lymphopenia is a known negative prognostic factor in terms of OS and DFS in several malignancies, including esophageal carcinoma (7,26). This study was designed to evaluate the relationship between radiation dose to the vertebral bone marrow, peripheral lymphocytes, and spleen in patients undergoing curative intent chemoradiotherapy for EC. Our data demonstrated that radiation dose to thoracic vertebral volumes during treatment with CCRT is correlated to both grade 4 lymphopenia and lymphocyte nadir in a dose dependent manner. More specifically we found that VV10, VV20, VV30, and MBD were strongly associated with our primary endpoint of G4HL and ALC. Using ROC analysis, we identified practical thresholds for vertebral marrow irradiation, above which the risk of lymphopenia increases significantly. These data, once confirmed with prospective analysis, may establish vertebral bone marrow as an OAR during CRT for EC.
Several secondary findings of this study are worth emphasizing. Our data demonstrate that patients with treatment associated lymphopenia are significantly more likely to miss one or more doses of chemotherapy which has been shown to have negative prognostic implications in NSCLC (26) and other malignancies (27). Importantly, this implies that radiotherapy field design and vertebral BM sparing techniques may improve tolerance and completion of systemic therapy in EC patients.
Finally, while we have suggested practical threshold values for vertebral body dosing, the data represented in Figure 2 displays a sigmoidal relationship between lymphopenia risk of irradiated vertebral volume consistent with the parallel organ structure of bone marrow.
The association between radiation dose to BM and peripheral cytopenias has been well described in patients treated with radiotherapy to the vertebral bones. The vertebral bodies host nearly 20% of the total active marrow and a review of two hundred patients with lung cancer has revealed that the dose to the vertebral bones has a direct causal association with cytopenias (21). In our series, with a separate thoracic malignancy, we have found a similar relationship between the importance of marrow and dose. The marrow suppressive effects of craniospinal irradiation are well known and leukopenia is lessened when vertebral BM sparing techniques are employed (28).
In a prospective trial, Carmona et al. identified significant MRI changes to vertebral BM proton fat density (a measure of active hematopoietic marrow) pre- and post-CRT, which could have protracted effects (16). Interestingly while we could not correlate the doses to the vertebral marrow and lymphopenia at 3 months the median counts were significantly lower than at baseline potentially suggesting a longer-term effect of radiation dose to the marrow.
Further radiographic evidence correlating specific sites of BM irradiation and hematological toxicity comes from Rose et al. who demonstrated WBC nadirs correlated with radiation dose to FDG-avid, active marrow, but not to FDG avid, inactive marrow (29). Finally, irradiation of vertebral bone marrow, in comparison to hematopoietic reservoirs, may have unique effects on the systemic lymphocyte pool as in murine models it has been shown that the vertebrae house a greater proportion of CD8+ lymphocytes compared to other bones (30). While this requires confirmation in prospective studies, this could have important clinical implications as immunotherapies become integrated into combined modality therapies involving thoracic radiation.
While our data demonstrate a correlation between vertebral bone (marrow) irradiation with lymphopenia, some of the decline in measured lymphocyte counts during CRT could be related to direct radiation effects on the circulating lymphocyte pool and other lymphoid tissue. The relative radiosensitivity of lymphocytes is well established (10) and with this understanding, it has been suggested that mean body dose may be a surrogate measure of radiotherapy to the circulating lymphocyte pool (7). Davuluri et al. attribute their finding that proton beam therapy less associated with G4HL due to less peripheral dose and field size (7). While we did find a correlation between MBD and lymphopenia, using this metric as a correlate to peripheral lymphocyte exposure is limited for several reasons. First, mean body dose is calculated on a volume incorporating a significant proportion of bone marrow. Second, valid patient to patient dosimetric evaluation of MBD implies that each patient would be undergo identical length simulation scan. Finally, it may be impractical to utilize MBD as a criterion for plan optimization except perhaps in the limited scenarios where comparisons between particle therapy (e.g., protons) plans and photon therapy plans.
In an attempt to measure the effects of radiation dose to the circulating lymphocyte pool in a standardized manner, we analyzed mean thoracic radiation dose to a novel volume (sTV) designed to encompass the irradiated thoracic blood pool (excluding marrow) which we presume captures the majority of cardiac output during daily RT delivery. While our study may be underpowered to detect this end point, it is notable that radiation dose to this volume did not correlate with either G4HL or lymphocyte nadir which is contrary to what we had initially hypothesized. Interestingly, as previously discussed, we found mean body dose to be associated with lymphocyte nadir, similar to previously reported studies (7). This could be suggestive of a cumulative dose to circulating cells as well as progenitor cells in the BM.
Furthermore, while we did find an association between SV5CC and absolute lymphopenia count, this relationship did not hold on multivariable analysis nor was it significant when included in a model with the thoracic vertebral bone parameters. Similar to what Liu et al. had found in their analysis on hepatocellular carcinoma, we did find lower splenic doses receiving radiation to have some association (22) albeit on univariable analysis. While dose to the spleen likely plays a role, we do not believe it is the primary contributor. Advances in planning are likely to spare to the spleen more so than the vertebral bones based on anatomical proximity. Furthermore, the spleen has less marrow reserve compared to the vertebral bones. Our data combined with the previously discussed translational and clinical work serve to measure the importance.
Contrary to our initial expectations, our analysis did not reveal any significant differences in lymphopenia risk when comparing patients treated with IMRT vs. 3DCRT. While this may have been due to power limitations due to our population size, it may also be related to the dosimetric tradeoffs inherent in IMRT planning. IMRT planning algorithms optimize beam paths in relation to OARs and unless prioritized during treatment planning, dose pushed into vertebral bodies can be substantial in such plans, especially given their proximity to the esophagus. Furthermore, IMRT plans may more broadly disperse low dose to both sensitive peripheral lymphocytes and marrow progenitors. It is notable that during the course of our analysis, we observed three cases treated with 3DCRT planning with beam arrangements leading to exceptionally low dose to the bone marrow and very minimal lymphocytotoxicity (Figure 3); in these individual cases there was no G4HL.
Taken together, data from this analysis supports the conclusion that irradiation to vertebral bone marrow is a potentially modifiable risk factor for lymphopenia with important clinical implications. We acknowledge that damage to circulating lymphocytes as well as indirect BM inhibition due to inflammatory mediators in blood stream can also serve as additive factors (31). These confounders though do not in themselves suggest that VVert dose should not be prioritized to some degree during treatment planning, as doing so would likely reduce lymphopenia.
In addition to standard biases inherent to retrospective research, there are other limitations to the scope of this study. Although our selection criteria were well defined, it led to the inclusion of a broad spectrum of patients and there were some imbalanced base line characteristics in our population. Furthermore, we did not exclude patients who underwent induction chemotherapy as long as they had normal baseline values prior to administration of chemoradiotherapy. While induction chemotherapy did not correlate with lymphopenia on univariable analysis, induction therapy could theoretically deplete marrow reserve in manner not detectable in a study our sample size. Ideally all regimens and timing of chemotherapy should be the same which could be ensured in prospective study.
Acknowledgments
Funding: None.
Footnote
Conflicts of Interest: All authors have completed the ICMJE uniform disclosure form (available at http://dx.doi.org/10.21037/jtd.2020.03.93). The authors have no conflicts of interest to declare.
Ethical Statement: The authors are accountable for all aspects of the work in ensuring that questions related to the accuracy or integrity of any part of the work are appropriately investigated and resolved. With investigational review board (IRB) approval, IRB #171972.
Open Access Statement: This is an Open Access article distributed in accordance with the Creative Commons Attribution-NonCommercial-NoDerivs 4.0 International License (CC BY-NC-ND 4.0), which permits the non-commercial replication and distribution of the article with the strict proviso that no changes or edits are made and the original work is properly cited (including links to both the formal publication through the relevant DOI and the license). See: https://creativecommons.org/licenses/by-nc-nd/4.0/.
References
- Siegel RL, Miller KD, Jemal A. Cancer statistics, 2018. CA Cancer J Clin 2018;68:7-30. [Crossref] [PubMed]
- van Hagen P, Hulshof MC, van Lanschot JJ, et al. Preoperative chemoradiotherapy for esophageal or junctional cancer. N Engl J Med 2012;366:2074-84. [Crossref] [PubMed]
- Herskovic A, Martz K, al-Sarraf M, et al. Combined chemotherapy and radiotherapy compared with radiotherapy alone in patients with cancer of the esophagus. N Engl J Med 1992;326:1593-8. [Crossref] [PubMed]
- Campian JL, Ye X, Brock M, et al. Treatment-related lymphopenia in patients with stage III non-small-cell lung cancer. Cancer Invest 2013;31:183-8. [Crossref] [PubMed]
- Balmanoukian A, Ye X, Herman J, et al. The association between treatment-related lymphopenia and survival in newly diagnosed patients with resected adenocarcinoma of the pancreas. Cancer Invest 2012;30:571-6. [Crossref] [PubMed]
- Cho O, Oh YT, Chun M, et al. Radiation-related lymphopenia as a new prognostic factor in limited-stage small cell lung cancer. Tumour Biol 2016;37:971-8. [Crossref] [PubMed]
- Davuluri R, Jiang W, Fang P, et al. Lymphocyte Nadir and Esophageal Cancer Survival Outcomes After Chemoradiation Therapy. Int J Radiat Oncol Biol Phys 2017;99:128-35. [Crossref] [PubMed]
- Afghahi A, Purington N, Han SS, et al. Higher Absolute Lymphocyte Counts Predict Lower Mortality from Early-Stage Triple-Negative Breast Cancer. Clin Cancer Res 2018;24:2851-8. [Crossref] [PubMed]
- Hung K, Hayashi R, Lafond-Walker A, et al. The central role of CD4(+) T cells in the antitumor immune response. J Exp Med 1998;188:2357-68. [Crossref] [PubMed]
- Venkatesulu BP, Mallick S, Lin SH, et al. A systematic review of the influence of radiation-induced lymphopenia on survival outcomes in solid tumors. Crit Rev Oncol Hematol 2018;123:42-51. [Crossref] [PubMed]
- Santin AD, Hermonat PL, Ravaggi A, et al. Effects of concurrent cisplatinum administration during radiotherapy vs. radiotherapy alone on the immune function of patients with cancer of the uterine cervix. Int J Radiat Oncol Biol Phys 2000;48:997-1006. [Crossref] [PubMed]
- Mauch P, Constine L, Greenberger J, et al. Hematopoietic stem cell compartment: acute and late effects of radiation therapy and chemotherapy. Int J Radiat Oncol Biol Phys 1995;31:1319-39. [Crossref] [PubMed]
- Newman NB, Sidhu MK, Baby R, et al. Long-Term Bone Marrow Suppression During Postoperative Chemotherapy in Rectal Cancer Patients After Preoperative Chemoradiation Therapy. Int J Radiat Oncol Biol Phys 2016;94:1052-60. [Crossref] [PubMed]
- Yang TJ, Oh JH, Apte A, et al. Clinical and dosimetric predictors of acute hematologic toxicity in rectal cancer patients undergoing chemoradiotherapy. Radiother Oncol 2014;113:29-34. [Crossref] [PubMed]
- Mell LK, Schomas DA, Salama JK, et al. Association between bone marrow dosimetric parameters and acute hematologic toxicity in anal cancer patients treated with concurrent chemotherapy and intensity-modulated radiotherapy. Int J Radiat Oncol Biol Phys 2008;70:1431-7. [Crossref] [PubMed]
- Carmona R, Pritz J, Bydder M, et al. Fat composition changes in bone marrow during chemotherapy and radiation therapy. Int J Radiat Oncol Biol Phys 2014;90:155-63. [Crossref] [PubMed]
- Kachnic LA, Winter K, Myerson RJ, et al. RTOG 0529: a phase 2 evaluation of dose-painted intensity modulated radiation therapy in combination with 5-fluorouracil and mitomycin-C for the reduction of acute morbidity in carcinoma of the anal canal. Int J Radiat Oncol Biol Phys 2013;86:27-33. [Crossref] [PubMed]
- Bazan JG, Luxton G, Kozak MM, et al. Impact of chemotherapy on normal tissue complication probability models of acute hematologic toxicity in patients receiving pelvic intensity modulated radiation therapy. Int J Radiat Oncol Biol Phys 2013;87:983-91. [Crossref] [PubMed]
- Ellis RE. The distribution of active bone marrow in the adult. Phys Med Biol 1961;5:255-8. [Crossref] [PubMed]
- Deek MP, Benenati B, Kim S, et al. Thoracic Vertebral Body Irradiation Contributes to Acute Hematologic Toxicity During Chemoradiation Therapy for Non-Small Cell Lung Cancer. Int J Radiat Oncol Biol Phys 2016;94:147-54. [Crossref] [PubMed]
- Barney CL, Scoville N, Allan E, et al. Radiation Dose to the Thoracic Vertebral Bodies Is Associated With Acute Hematologic Toxicities in Patients Receiving Concurrent Chemoradiation for Lung Cancer: Results of a Single-Center Retrospective Analysis. Int J Radiat Oncol Biol Phys 2018;100:748-55. [Crossref] [PubMed]
- Liu J, Zhao Q, Deng W, et al. Radiation-related lymphopenia is associated with spleen irradiation dose during radiotherapy in patients with hepatocellular carcinoma. Radiat Oncol 2017;12:90. [Crossref] [PubMed]
- Antonia SJ, Ozguroglu M. Durvalumab in Stage III Non-Small-Cell Lung Cancer. N Engl J Med 2018;378:869-70. [PubMed]
- Zhang A, Deek MP, Kim S, et al. Vertebral body irradiation during chemoradiation therapy for esophageal cancer contributes to acute bone marrow toxicity. J Gastrointest Oncol 2019;10:513-22. [Crossref] [PubMed]
- Suntharalingam M, Winter K, Ilson D, et al. Effect of the Addition of Cetuximab to Paclitaxel, Cisplatin, and Radiation Therapy for Patients With Esophageal Cancer: The NRG Oncology RTOG 0436 Phase 3 Randomized Clinical Trial. JAMA Oncol 2017;3:1520-8. [Crossref] [PubMed]
- Deek MP, Kim S, Beck R, et al. Variations in Initiation Dates of Chemotherapy and Radiation Therapy for Definitive Management of Inoperable Non-Small Cell Lung Cancer Are Associated With Decreases in Overall Survival. Clin Lung Cancer 2018;19:e381-90. [Crossref] [PubMed]
- Matsumoto I, Tanaka M, Shirakawa S, et al. Postoperative Serum Albumin Level is a Marker of Incomplete Adjuvant Chemotherapy in Patients with Pancreatic Ductal Adenocarcinoma. Ann Surg Oncol 2015;22:2408-15. [Crossref] [PubMed]
- Brown AP, Barney CL, Grosshans DR, et al. Proton beam craniospinal irradiation reduces acute toxicity for adults with medulloblastoma. Int J Radiat Oncol Biol Phys 2013;86:277-84. [Crossref] [PubMed]
- Rose BS, Aydogan B, Liang Y, et al. Normal tissue complication probability modeling of acute hematologic toxicity in cervical cancer patients treated with chemoradiotherapy. Int J Radiat Oncol Biol Phys 2011;79:800-7. [Crossref] [PubMed]
- Geerman S, Hickson S, Brasser G, et al. Quantitative and Qualitative Analysis of Bone Marrow CD8(+) T Cells from Different Bones Uncovers a Major Contribution of the Bone Marrow in the Vertebrae. Front Immunol 2016;6:660. [PubMed]
- Prise KM, Schettino G, Folkard M, et al. New insights on cell death from radiation exposure. Lancet Oncol 2005;6:520-8. [Crossref] [PubMed]