Role of theranostics in thoracic oncology
Introduction
Theranostics was coined as a portmanteau of “therapeutic” and “diagnostic” (1). Theranostics can also be described as an agent of theranosis: an agent used in tailoring treatment to an individual (2). Theranostics, then, can be used to describe personalized medicine approaches as well as dual-functioning drugs. Theranostics are allowing physicians to use classically diagnostic tools as trackers of therapeutic efficacy as in the case of targeted positron emission tomography (PET) radiotracers. The current state of theranostic research is rich, diverse, and creative in scientists’ attempts to minimize the exposure of patients to drugs. Looking at research from the past 4 years, one could find applications of novel PET radiotracers, metal nanoparticles, quantum dot aggregates, decorated liposomes, and even catalytically-active DNA molecules (DNAzymes) (3-6). This mini-review will discuss theranostic agents in clinical practice.
Most theranostic clinical work is being done in oncology, especially since the American Cancer Society (ACS) estimated that this year in the United States: over 15 million people live with cancer, with 1.7 million new cases, and 0.7 million deaths resulting from cancer (7). Globally, the ACS estimated 17 million new cases and 9.5 million deaths will result from cancer in 2018. These tolls will only continue to climb in coming years with an increasing population (8). Therefore, we will only focus on theranostics in an oncologic context.
Theranostic agents could be created by either starting with a targeted therapeutic such as an antibody and attaching a radioactive isotope to use a traditionally diagnostic method such as PET, CT, or SPECT to determine the localization of that therapeutic. The technique of radiolabeling an existing therapeutic utilizes the existing qualities of that drug, whether it is specific or FDA-approved and combines it with the diagnostic power of radiology to individualize treatments. In individualizing treatments, the physician can assess the potential efficacy prior to prescribing a patient to a full regimen of a particular drug.
Several groups, including ours, have radioconjugated various radioisotopes to antibodies. We are currently working on radioconjugating checkpoint inhibitors to determine their potential as a predictor of response in the pre-treatment setting. Examples of other groups pursuing this research include the current phase II clinical trial (NCT03065764) of 89Zr-labeled pembrolizumab (Keytruda) for NSCLC (9).
The choice of radioisotope is one that requires a depth of understanding that entire chapters could be written on. However, to succinctly describe the parameters involved, one must consider whether they want an alpha (α), beta (β), positron (antielectron, e+) or gamma (γ) emitter. Additionally, one must also consider the half-life, the distribution of energy emitted by the radioisotope, the penetration depth of the alpha/beta particles, the availability and ease of acquisition/fabrication of the radioisotope, and also the ease of chemically working with the element (10). With these considerations in mind, the most commonly used radioisotopes in the thorax include 18F, 32P, 64Cu, 68Ga, 90Y, 99mTc, 123-125, 131I, and, 177Lu (10-13). A compilation of these radiotracers, their decay mode, and half-lives can be found in the table below (Table 1).
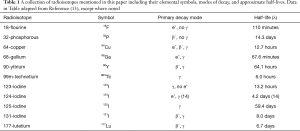
Full table
These radioisotopes can then be used and inserted or attached to a parent molecule that is known to localize to a particular organ or tissue type for diagnosis and/or treatment.
For example, 18F-FET (o-(2-[18F]fluoroethyl)-l-tyrosine) is a radiolabeled tyrosine mimetic that is incorporated into rapidly-diving cells due to increased amino acid uptake in cancer cells. 18F-FET targets tumors and other resource intensive organs such as the liver, assisting in diagnosis of non-hepatic tumors (15).
Another PET radiotracer is 18F-FLT (3'-[18F]fluoro-3'-deoxythymidine) which is a thymidine nucleoside analog and is thus incorporated into rapidly dividing cells requiring an increased amount of individual nucleic acids (15).
The thoracic cavity can be compartmentalized into the lungs, thymus, thyroid, and mediastinum which houses the heart, major blood vessels, and many lymph nodes. Since the thyroid is covered in another article by our group and information about the heart would best be found in a cardiac journal, they will not be discussed in this mini-review. Rather, we focus here on the lungs, thymus, and lymph nodes.
Lungs
Approximately 13% of all new cancer diagnoses in the United States are lung cancer, resulting in over 200,000 new cases of lung cancer per year (7). Globally, over 2 million new cases and 1.7 million deaths are estimated (8). Lung cancer also leads as the cause of death in front of colon, breast, and prostate cancer combined (7,8).
Small cell lung cancer (SCLC)
SCLCs are neuroendocrine tumors (NETs), and as such, can be targeted with somatostatin analogs (SSAs), which bind to somatostatin receptors (SSTRs), or norepinephrine analogs which localize to adrenergic tissue (12,16).
For diagnosis and treatment utilizing SSTRs, molecules like DOTA-TATE or DOTA-TOC are useful. A chelation of 68Ga allows for the effective diagnosis using PET or SPECT while the chelation of 177Lu or 90Y allows for the treatment of NETs (12). Additionally, any somatostatin analog can be labeling with 111In to aid in diagnosis.
The primary molecule mimicking norepinephrine in a theranostic context is mIBG (metaiodobenzyl-guanidine, or iobenguane). The radiolabeling of mIBG to either 123I or 124I is used for diagnosis, while the use of 131I can both image and treat (12). In terms of clinical updates, Azedra® is an 131I-mIBG drug that has been recently approved by the FDA for treatment of pheochromocytomas, targeting unresectable adrenal tumors, but can also target NETs due to mIBG being the parent molecule and could be investigated for off-label use (17).
Non-small cell lung cancer (NSCLC)
NSCLC accounts for 85% of all lung cancer diagnoses (7). NSCLCs are all cancers that are not small cell cancers and can be sub-divided, in descending order, into: adenocarcinomas (ADCs), squamous cell carcinomas (SCCs), and large cell carcinomas (a biopsy-based diagnosis of exclusion as of 2015, and therefore beyond the scope of this context) (7,18). For further reading about NSCLC therapy with TKI-PET and immune-PET, see the following reference (19).
ADCs
ADC overexpress epidermal growth factor receptors (EGFRs) like HER/ERBB 1–4, whose activation leads to the over-phosphorylation of tyrosine (20). Tyrosine kinase inhibitors (TKIs) which normally block EGFRs such as gefitinib and erlotinib are used to retard the progression of ADC due to their overexpression of EGFR (20). Likewise, antibody treatments exist which target EGFR such as cetuximab which carries the advantages of the antibody’s specificity and of triggering immunogenic cell death of the tumor cells (21). Because FET is a tyrosine mimetic, it follows that it can be used as a diagnostic tool to investigate the efficacy of TKI activity.
Another emerging target against tyrosine kinase is the Axl receptor, whose activation promotes tyrosine phosphorylation as well. Axl is a member of the TAM (Tyro3, Axl, Mer) family and by inhibiting the receptor, drug sensitivity can be restored to patients who have become desensitized to other TKIs (22). Currently TP-0903 and BGB324 are undergoing Phase I clinical trials in the United States.
An example of theranosis is the recent development of a radiopharmaceutical, Osimertinib, that selectively targets the most common NSCLC mutation, EGFR-T790M. This mutant protein renders traditional TKIs ineffective. Osimertinib was compared against EGFR-WT and EGFR-L858R in vivo using 18F-FEWS to identify standard TKI-resistance NSCLC tumors. Following this research a physician can then use this drug to detect TKI-resistance and made the decide whether to cease treatment using typical drugs like erlotinib and switch to FDA-approved EGFR mutant T790M-targeting treatments such as osimertinib (23,24). Cycling between these dugs would prevent for the complete selection of treatment-resistant NSCLC and increase the survival of patients who would otherwise need harsher treatment.
SCCs
SCCs are less frequently diagnosed than ADC and primarily differ in their morphology. However, the detection of differential mRNA signatures has been developed to help classify biopsies between ADC and SCC, especially since less voluminous specimens are being used for histological analyses (7,18,25). SCCs express greater TP63, KRT-type genes, and squamous cell carcinoma antigen (SSCA) than ADCs, so RNA-seq from biopsy would give a clear diagnosis, but noninvasive methods exist as well (25).
Because SCCs do not overexpress EGFRs like their ADC NSCLC counterparts, 18F-FLT in combination with 18F-FET can be used to exclude an ADC diagnosis (with 18F-FLT localizing to any site of rapid DNA synthesis and 18FET localizing to areas of EGFR overexpression) (15).
Conclusions of lungs
Lung cancer maintains as one of the largest cancer threats due to its high rates of diagnosis and death. However, modern radiotracing techniques now allow for physicians to more quickly diagnose, and therefore tailor, their treatment approach to individual patients. In short, if a patient has SCLC, the cells likely overexpress SSTRs and the use of a radiolabeled mIBG or DOTA-TATE/-TOC would be useful for both treatment and diagnosis. If a patient has NSCLC, then the ADC types likely overexpress EGFRs and TKIs would be useful in slowing the progression or be used as an adjuvant therapy. The SCC types can be diagnosed using less specific PET markers by demonstrating a lack of specificity to EGFR receptors while maintaining an uptake of other radiotracers in the lungs.
Thymus
Of the rare occurrence of disorders in the thymus, myasthenia gravis (MG) a disease impacting muscle function and is often caused by thymomas (26). For treatment of MG, a full resection of the thymus is recommended when a thymoma is discovered or highly suspected. Thymomas can suspected by a lack of auto-antibodies and an enlarged thymus in imaging (26). Otherwise, MG can be treated with acetylcholine esterase inhibitors, steroids like prednisolone, and immuno-suppressive drugs (26). Proven theranostic methods do not exist but could include radio-labeling the drugs used for treatment to track disease progression.
Lymph nodes
Lymphomas can be detected using standard imaging techniques including PET/CT or PET/MRI. 18F-FDG (a fluorinated glucose analog) can be used to detect nodal and non-nodal tumors with PET/MRI which is markedly more predictive in diagnosing Lymphoma than MRI-DWI (Diffusion Weighted Imaging) alone (27). Other work in diffuse large B-cell lymphoma (DLBCL) compared 18F-FDG to 18F-FLT and found 18F-FLT to provide a significantly higher positive predictive value (PPV) (28). This is important for physicians not only as a diagnostic tool, but also as a way to track disease progression, especially when utilizing chimeric antigen receptor (CAR) T cell therapies because the PET techniques can track the presence and activity of the lymphocytes (29).
CAR T cell therapy is an inherently attractive choice for hematological diseases such as lymphomas due to the CAR T cell already being able to travel through the lymph system. In CAR T cell therapy, a patient’s own T cells are genetically modified with an exogenous antigen receptor so that the specific surface markers of a target disease can be targeted by the patient’s own immune system (29).
In the realm of theranosis, anti-CD19 CAR T cells have been demonstrated to be effective in patients with chemotherapy-refractory lymphoma and sometimes lead to remissions lasting longer than 2 years (29).
Metastases
The metastasis of cancer cells to thoracic organs is often considered fatal (cite). To this end, the detection of metastatic tumors within the thorax would provide physicians with the ability to more effectively treat a patient by being able to identify and remove otherwise invisible tumors. Others have shown the efficacy of radiolabeling the prostate-specific membrane antigen (PSMA) (30,31). Indeed, in our own trial (NCT04216134) we have used 68Ga conjugated to PSMA and been able to effectively determine the presence of prostate cancer metastases in the thorax where the standard-of-care technique using 18FDG did not (Figure 1).
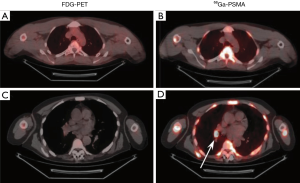
Conclusions
Theranostics can describe an agent of personalized medicine or an agent which can simultaneously treat while diagnosing a patient. In an era of advancing technologies and increased attention on the quality of life and treatment of an individual patient, it is important to find new methods that result in the least amount of suffering during treatment.
In the cage of the thorax, the lungs take up the majority of the space as well as the majority of the cancer cases. This places greater importance on being able to make swift, accurate, and direct diagnoses and treatment options for patients. As such, the FDA-approved mIBG, FLT, and FET have shown themselves capable in diagnosing and treating lung cancers. Within lymphomas, FDG and FLT will assist in accurate diagnosis and disease progression. In the thymus, the detection of thymomas suspect of, or causing disease are resectable and further treatable with conventional and immune-suppressive therapies. And finally, metastases can be detected within the thorax by targeting specific antigens or markers along with a radiotracer.
The information presented in this mini-review should act as a brief overview of the strengths of radiotracers and antibodies not only as diagnostic or therapeutic tools, but also as a foundation for the delivery of other radioactive elements to target sites for the treatment and tracking of disease. Ultimately, the goal of theranosis is to individualize the treatment a patient receives as to not suffer unnecessarily by the treatment meant to reduce that very same suffering.
Acknowledgments
Funding: This work was supported by the National Cancer Institute of the National Institutes of Health under grant: 5K12CA001727.
Footnote
Provenance and Peer Review: This article was commissioned by the Guest Editor (Ammar Chaudhry) for the series “Role of Precision Imaging in Thoracic Disease. The article was sent for external peer review organized by the Guest Editor and the editorial office.
Conflicts of Interest: All authors have completed the ICMJE uniform disclosure form (available at: http://dx.doi.org/10.21037/jtd-2019-pitd-13). The series “Role of Precision Imaging in Thoracic Disease” was commissioned by the editorial office without any funding or sponsorship. AC served as an unpaid Guest Editor of the series. The other authors have no other conflicts of interest to declare.
Ethical Statement: The authors are accountable for all aspects of the work in ensuring that questions related to the accuracy or integrity of any part of the work are appropriately investigated and resolved.
Open Access Statement: This is an Open Access article distributed in accordance with the Creative Commons Attribution-NonCommercial-NoDerivs 4.0 International License (CC BY-NC-ND 4.0), which permits the non-commercial replication and distribution of the article with the strict proviso that no changes or edits are made and the original work is properly cited (including links to both the formal publication through the relevant DOI and the license). See: https://creativecommons.org/licenses/by-nc-nd/4.0/.
References
- Gilban I. THERANOSTICS an emerging tool in drug discovery and commercialisation. Drug Discover World 2002;Fall 1:17-23.
- DeNardo GL, DeNardo SJ. Concepts, consequences, and implications of theranosis. Semin Nucl Med 2012;42:147-50. [Crossref] [PubMed]
- Sharma H, Mishra PK, Talegaonkar S, et al. Metal nanoparticles: a theranostic nanotool against cancer. Drug Discov Today 2015;20:1143-51. [Crossref] [PubMed]
- Feng G, Liu B. Aggregation-Induced Emission (AIE) Dots: Emerging Theranostic Nanolights. Acc Chem Res 2018;51:1404-14. [Crossref] [PubMed]
- Feng LZ, Gao M, Tao DL, et al. Cisplatin-Prodrug-Constructed Liposomes as a Versatile Theranostic Nanoplatform for Bimodal Imaging Guided Combination Cancer Therapy. Advanced Functional Materials 2016;26:2207-17. [Crossref]
- Zhou W, Ding J, Liu J. Theranostic DNAzymes. Theranostics 2017;7:1010-25. [Crossref] [PubMed]
- Siegel RL, Miller KD, Jemal A. Cancer statistics, 2019. CA Cancer J Clin 2019;69:7-34. [Crossref] [PubMed]
- Bray F, Ferlay J, Soerjomataram I, et al. Global cancer statistics 2018: GLOBOCAN estimates of incidence and mortality worldwide for 36 cancers in 185 countries. CA Cancer J Clin 2018;68:394-424. [Crossref] [PubMed]
- Center VUUM, Smit EF, Merck S, et al. 89Zr-labeled Pembrolizumab in Patients With Non-small-cell Lung Cancer. 2019.
- Das T, Pillai MR. Options to meet the future global demand of radionuclides for radionuclide therapy. Nucl Med Biol 2013;40:23-32. [Crossref] [PubMed]
- Maecke HR, Reubi JC. Somatostatin receptors as targets for nuclear medicine imaging and radionuclide treatment. J Nucl Med 2011;52:841-4. [Crossref] [PubMed]
- Yordanova A, Eppard E, Kurpig S, et al. Theranostics in nuclear medicine practice. Onco Targets Ther 2017;10:4821-8. [Crossref] [PubMed]
- Grupen C. Introduction to radiation protection: practical knowledge for handling radioactive sources. Graduate texts in physics. Berlin; New York: Springer, 2010.
- Cascini GL, Niccoli Asabella A, Notaristefano A, et al. 124 Iodine: a longer-life positron emitter isotope-new opportunities in molecular imaging. Biomed Res Int 2014;2014:672094. [Crossref] [PubMed]
- Lee TS, Ahn SH, Moon BS, et al. Comparison of 18F-FDG, 18F-FET and 18F-FLT for differentiation between tumor and inflammation in rats. Nucl Med Biol 2009;36:681-6. [Crossref] [PubMed]
- Mizutani G, Nakanishi Y, Watanabe N, et al. Expression of Somatostatin Receptor (SSTR) Subtypes (SSTR-1, 2A, 3, 4 and 5) in Neuroendocrine Tumors Using Real-time RT-PCR Method and Immunohistochemistry. Acta Histochem Cytochem 2012;45:167-76. [Crossref] [PubMed]
- FDA Approves AZEDRA Specified Use in Pheochromocytomas/Paragangliomas. Journal of Nuclear Medicine 2018;59:17.
- Travis WD, Brambilla E, Nicholson AG, et al. The 2015 World Health Organization Classification of Lung Tumors: Impact of Genetic, Clinical and Radiologic Advances Since the 2004 Classification. J Thorac Oncol 2015;10:1243-60. [Crossref] [PubMed]
- Bahce I, Yaqub M, Smit EF, et al. Personalizing NSCLC therapy by characterizing tumors using TKI-PET and immuno-PET. Lung Cancer 2017;107:1-13. [Crossref] [PubMed]
- Sridhar SS, Seymour L, Shepherd FA. Inhibitors of epidermal-growth-factor receptors: a review of clinical research with a focus on non-small-cell lung cancer. Lancet Oncol 2003;4:397-406. [Crossref] [PubMed]
- Mazzarella L, Guida A, Curigliano G. Cetuximab for treating non-small cell lung cancer. Expert Opin Biol Ther 2018;18:483-93. [Crossref] [PubMed]
- Gay CM, Balaji K, Byers LA. Giving AXL the axe: targeting AXL in human malignancy. Br J Cancer 2017;116:415-23. [Crossref] [PubMed]
- Goggi JL, Haslop A, Ramasamy B, et al. Identifying nonsmall-cell lung tumours bearing the T790M EGFR TKI resistance mutation using PET imaging. J Labelled Comp Radiopharm 2019;62:596-603. [Crossref] [PubMed]
- Passaro A, Guerini-Rocco E, Pochesci A, et al. Targeting EGFR T790M mutation in NSCLC: From biology to evaluation and treatment. Pharmacol Res 2017;117:406-15. [Crossref] [PubMed]
- Girard L, Rodriguez-Canales J, Behrens C, et al. An Expression Signature as an Aid to the Histologic Classification of Non-Small Cell Lung Cancer. Clin Cancer Res 2016;22:4880-9. [Crossref] [PubMed]
- Gilhus NE, Verschuuren JJ. Myasthenia gravis: subgroup classification and therapeutic strategies. Lancet Neurol 2015;14:1023-36. [Crossref] [PubMed]
- Heacock L, Weissbrot J, Raad R, et al. PET/MRI for the evaluation of patients with lymphoma: initial observations. AJR Am J Roentgenol 2015;204:842-8. [Crossref] [PubMed]
- Minamimoto R, Fayad L, Advani R, et al. Diffuse Large B-Cell Lymphoma: Prospective Multicenter Comparison of Early Interim FLT PET/CT versus FDG PET/CT with IHP, EORTC, Deauville, and PERCIST Criteria for Early Therapeutic Monitoring. Radiology 2016;280:220-9. [Crossref] [PubMed]
- Brudno JN, Kochenderfer JN. Chimeric antigen receptor T-cell therapies for lymphoma. Nat Rev Clin Oncol 2018;15:31-46. [Crossref] [PubMed]
- Hofman MS, Lawrentschuk N, Francis RJ, et al. Prostate-specific membrane antigen PET-CT in patients with high-risk prostate cancer before curative-intent surgery or radiotherapy (proPSMA): a prospective, randomised, multi-centre study. Lancet 2020;395:1208-16. [Crossref] [PubMed]
- Tanaka T, Yang M, Froemming AT, et al. Current Imaging Techniques for and Imaging Spectrum of Prostate Cancer Recurrence and Metastasis: A Pictorial Review. Radiographics 2020;40:709-26. [Crossref] [PubMed]