The role of ATP in cough hypersensitivity syndrome: new targets for treatment
Introduction
Chronic cough refers to a group of diseases in which cough symptoms last for more than 8 weeks. Common causes of chronic cough include upper airway cough syndrome, cough variant asthma, eosinophilic bronchitis, atopic cough, and gastroesophageal cough (1), and it can be effectively controlled in most patients by treatment of symptoms; however, there remain a small number of patients with unidentifiable etiology, or where treatment has poor efficacy once the etiology is identified, in whom the condition is referred to as unexplained chronic cough or refractory chronic cough (2-4). Patients with refractory chronic cough or unexplained chronic cough commonly have the clinical/pathophysiological characteristic of increased cough reflex sensitivity (4). In clinical practice, Morice et al. (5) described this group of patients as having cough hypersensitivity syndrome (CHS), defined as a disease with chronic cough as the only, or only prominent, symptom.
Recent studies have found that extracellular ATP plays an important role in diseases involving airway inflammation (6-10) and that it is closely associated with cough (11,12). The antagonist of ATP P2X3 receptor, AF-219/MK-7264 (gefapixant) (13), is useful for treatment of refractory chronic cough or unexplained chronic cough (14-16); however, there is no consensus on how ATP affects cough reflex sensitivity. In this study, we review the function of ATP in CHS etiology.
Pathogenesis of CHS—the association of TRPV1 with refractory chronic cough
CHS is heterogeneous, with the main underlying mechanisms involving excitatory changes in relevant pathways, including peripheral cough receptors, vagal afferent nerves, the higher cough center, efferent nerves, and effectors, of which activation of the transient receptor potential (TRP) pathway, airway inflammation, and cough center facilitation, may be involved in the development of increased cough reflex sensitivity (17). TRP ion channels distributed in the airways are mainly include TRP vanilloid l (TRPV1), TRP vanilloid 4 (TRPV4), TRP ankyrin 1 (TRPA1), and TRP cation channel subfamily M member 8 (TRPM8) (18). An altered phenotype of TRPV1 expression in airways and central sensory afferent nerves may underlie the development of cough hypersensitivity (19). TRPV1 is a ligand-gated non-selective cation channel protein, expressed in primary sensory neurons, pulmonary smooth muscle cells, bronchial and tracheal epithelial cells, and pulmonary dendritic cells (20), and can be activated by non-selective stimuli, such as capsaicin, acid (pH ≤5.9), and heat (>43 °C). Such activation opens calcium channels, causing calcium influx and producing an action potential, which prompts vagal afferent C fibers to release neuropeptides, such as substance P (SP) and calcitonin gene-related peptide (CGRP), leading to local neurogenic inflammation or transmitting nerve impulses to the cough center, and increasing cough reflex sensitivity (18,21). TRPV1 was shown to mediate cough reflex in guinea pigs as early as 1995 (22). In guinea pig models with chronic cough, established by repeated aerosol inhalation of citric acid, increased capsaicin cough responses were also found to parallel tracheobronchial TRPV1 protein expression levels, suggesting that cough hypersensitivity is closely related to TRPV1 activation (23). Furthermore, TRPV1 expression is increased on sensory nerve fibers in the bronchial walls of patients with chronic cough (24). Thus, TRPV1 may be important in the development of CHS. Heightened cough responses to capsaicin inhalation have been described in patients with viral upper respiratory tract infections (25), asthma (26-28), chronic obstructive pulmonary disease (28,29), idiopathic fibrosis (30) and chronic cough (31,32). In addition, some clinical studies have found that leukotriene receptor antagonist (33), tiotropium (34) and bronchial thermoplasty (35) may ameliorate chronic asthmatic cough by altering the expression of TRPV1 to regulate capsaicin sensitivity; however, the clinical utility of TRPV1 antagonists is limited by their adverse effects, which include hyperthermia and impaired thermal sensation, as well as by their lack of stable pharmacokinetics and pharmacodynamics (36,37). In a double-blind randomized controlled trial conducted by Khalid et al., which was the first study to assess a TRPV1 receptor antagonist (SB-705498) as an antitussive agent in patients, SB-705498) failed to improve spontaneous cough frequency in patients with refractory chronic cough, but produced the reduction in capsaicin-evoked cough (38). Subsequently, Belvisi et al. validated that the potent TRPV1 inhibitor XEN-D051 showed superior efficacy and potency in preclinical and clinical capsaicin challenge studies, but was no associated with any improvements in objective cough frequency, further providing the evidence that TRPV1 is not a therapeutic effective target for refractory cough (39).
Role and potential mechanism of ATP in the etiology of CHS
ATP and P2 receptors
Under physiological conditions, cellular ATP functions in energy storage and energy supply, and the few ATP molecules released into the extracellular space are degraded to adenosine by extracellular nucleotidases; however, in inflamed airways, ATP is released into the extracellular space in large amounts, due to cell damage, stress, or hypoxia and, contributes to the development of disease and inflammation as a signaling molecule (9). Extracellular ATP can originate from nerve endings, red blood cells, platelets, skeletal muscle cells, ischemic and apoptotic cells, vascular smooth muscle cells, and endothelial or epithelial cells (8,40), and acts in an autocrine or paracrine manner on P2 purine receptors on the surface of target cells, thereby regulating cellular immune function.
In addition to cytolytic and extracellular secretion mechanisms, ATP release can even be induced by normal respiratory movements (41), when respiratory epithelium is subjected to stress; for example, by mechanical stimuli. Purinergic receptors include two major categories: P1 and P2. The ligand for the P1 receptor is adenosine, which has four subtypes: A1, A2A, A2B, and A3. ATP-bound P2 receptors include two major families, P2XR and P2YR, the former being ligand-ATP-gated non-selective cation channels, with seven known receptor subtypes, P2X1–7R, which allow the passage of cations, such as Ca2 +, Na2 +, and K+; when activated. Of these subtypes, P2X2R is widely distributed in the peripheral and central nervous systems, and P2X3R is primarily found in sensory nerve cells, including the trigeminal nerve, dorsal root ganglia, and nodose ganglia, and is closely associated with cough (42). P2X4R and P2X4/P2X6R heteromers are moderately expressed in the lung, while P2YR is a metabotropic G-protein coupled receptor expressed in almost all epithelial cells, and is responsible for fluid control and electrolyte transport, including P2Y1R, P2Y2R, P2Y4R, P2Y6R, and P2Y11-14R8 receptor subtypes (43). Among them, P2Y1R, P2Y2R, and P2Y4R are more strongly expressed in the airways and lungs (43,44), while P2Y1R is mainly related to transient platelet aggregation and deformation, and P2Y2R and P2Y4R are involved in regulation of the physiological functions of the respiratory system. (More specific distributions and functions of purinergic receptors are shown in Table S1).

Full table
ATP may be involved in the etiology of CHS
Airway inflammation and remodeling are common pathological changes in chronic cough caused by various intrapulmonary and extrapulmonary diseases; however, airway injury caused by persistent cough also leads to airway inflammation (45,46), which presents as pathological changes, such as mucosal vasodilatation, plasma exudation, tissue edema, epithelial injury, and inflammatory cell infiltration (46). Numerous studies have indicated the presence of extracellular ATP during the development of airway inflammation. Esther et al. found that ATP levels in bronchoalveolar lavage are inversely correlated with lung function in children with pulmonary cystic fibrosis, while extracellular purines, including ATP, can be used as biomarkers of neutrophilic airway inflammation (47). Basoglu et al. found that aerosol inhalation of ATP can produce cough in 70% of healthy subjects and 90% of those with mild intermittent asthma; however, ATP had no bronchoconstriction effect on healthy subjects. Extracellular ATP is involved in neuropathic bronchoconstriction, inflammation, and cough caused by P2 receptor sensitization in airway sensory nerves, which may contribute to the pathogenesis of asthma and obstructive airway diseases (10). Fowles et al. conducted a randomized controlled trial that recruited 20 healthy volunteers and 20 patients with chronic cough, with the same sex ratio in both groups. Each group was subjected to cough provocation tests with ATP and AMP; the primary endpoints were the differences between ATP and AMP, as well as the substance concentration provoking cough after 2 and 5 cough exposures (C2 and C5), between healthy volunteers and patients with chronic cough. They found that aerosol inhalation of ATP could produce cough in both healthy subjects and patients with chronic cough, and that C2 and C5 values were significantly lower in patients with chronic cough than those in healthy volunteers, while the response to ATP was more pronounced (12). Under pathological conditions, binding of extracellular ATP to P2 receptors can produce different physiological effects. ATP activates P2X2/3R, located on C and Aδ fibers, to stimulate vagal afferent nerve endings in the lungs, leading to the release of local neurogenic inflammatory mediators, bronchoconstriction, and cough (48,49). By activating P2Y6R, ATP causes the release of inflammatory factors, including IL-6 and IL-8, which amplifies inflammatory effects on the airway (50,51). IL-6 and IL-8 also contribute to the release of thromboxane from the airway epithelium via P2Y4 and P2Y6, causing allergic bronchospasm (52). Exonuclease E-NPP1 expression is decreased in blood leukocytes from patients with severe acute bronchial asthma episodes, and P2X7 and P2Y4 receptors mediate increased production of endogenous ATP, exacerbating asthma (53). Pelleg et al. found that ATP enhances IgE-mediated histamine release from mast cells (54). In addition, histamine also induces ATP release from subcutaneous and smooth muscle fibroblasts (55,56). Kamei et al. suggested that ATP did not cause cough directly, but may enhance cough reflex sensitivity in guinea pigs by becoming rapidly adapted to cough receptors, rather than the C-afferent fiber pathway, with P2X receptors an important link in this process (11,56).
P2 receptor antagonists can be used to treat unexplained refractory chronic cough
Selective P2X3R antagonists can be used to treat unexplained refractory chronic cough. P2X3R is an important vector for cough hypersensitivity that is mainly expressed on C-vagal afferent nerve fibers (57). A recent study using the whole-cell patch clamp technique in 1321N1 cells expressing human P2X3R and P2X2/3R receptors found that the reversible selective P2X3R and P2X2/3R antagonist, MK-7264 (gefapixant), exerted its antagonistic effects through negative allosteric modulation. In addition, in vivo experiments demonstrated that MK-7264 had high oral bioavailability in a rat model of neuropathic sensitization, could treat neuralgia, and had comparable efficacy to gabapentin, supporting its clinical application for diseases involving chronic hypersensitivity (13). In a randomized placebo-controlled clinical trial, Morice et al. demonstrated that oral administration of 100 mg of the P2X3 receptor antagonist, AF-219/MK-7264, in patients with chronic cough significantly reduced cough reflex sensitivity induced by inhaled ATP challenge 5 hours later; however, it had no inhibitory effect on cough hyperresponsiveness induced by capsaicin and citric acid challenge, indicating that AF-219/MK-7264 only inhibits P2X3 receptors on airway cough receptors, and is not associated with the inhibition of receptors such as TRPV1 (16). Further, a single-center randomized, double-blind, placebo-controlled phase II clinical study, conducted by Rayid Abdulqawi et al. (14), showed that oral administration of the P2X3 receptor antagonist, AF-219/MK-7264 (600 mg twice daily for 2 weeks), could significantly reduce cough frequency and severity, and improve the quality of life in patients with refractory chronic cough. Despite good antitussive effects, where cough frequency can be reduced by 75% in patients administered AF-219 compared with those receiving placebo, AF-219/MK-7264 was associated with frequent dysgeusia as a side-effect; all patients with refractory chronic cough taking AF-219 experienced this symptom, and six discontinued the drugs due to intolerance, which usually appeared within a few hours after administration, fully recovering by 24 hours after discontinuation. Subsequent dose-finding studies showed that reducing the dose of medication significantly diminished the incidence and extent of dysgeusia, while maintaining high efficacy, indicating that AF-219/MK-7264 has great promise for final inclusion in clinical treatment. To explore efficacy and tolerability of gefapixant (AF-219/MK-7264), the P2X3 receptor antagonist, for the treatment of chronic cough, Smith et al. designed two randomized, double-blind, placebo-controlled, crossover, dose-escalation studies (58). Both studies were composed of two 16-day treatment periods with either 3–7 days (Study 1) or 14–21 days (Study 2) washout periods. Study 1 was the high-dose cohort, in which gefapixant was received at four BID dose levels (50, 100, 150 and 200 mg); study 2 investigated a lower range of four BID dose levels (7.5, 15, 30 and 50 mg); both doses escalated every 4 days. This work suggested that compared with placebo, gefapixant dose ≥30 mg ameliorated cough utmostly and taste disturbances were closely related to the doses, most obvious at dose ≥150 mg. Gefapixant could maintain the maximum efficacy at a lower dose of 50 mg than previous research twice daily, well tolerated. Recent work about gefapixant in the treatment of refractory or unexplained chronic cough, a randomized, double-blind, controlled, parallel-group, phase 2b trial, was reported in Lancet Respir Med by Smith et al. (59), first to show the anti-tussive efficacy of P2X3 antagonist maintained over 12 weeks. Dosing regimen used in this study was set at 7.5 to 50 mg twice daily as a dose response plateau in the former dose-escalation research evaluating doses of gefapixant from 7.5 to 200 mg (58). 253 patients enrolled were randomly assigned to receive placebo or one of three doses (7.5, 20, or 50 mg) of oral gefapixant twice daily for 84 days and were found that inhibiting P2X3 receptor with gefapixant at a dose of 50 mg twice daily had good safety and tolerability, significantly reducing cough frequency in patients with refractory and unexplained chronic cough after 12-week treatment. This large-scale, multicenter study validated and complemented previous preliminary proof-of-concept studies on the anti-tussive efficacy of P2X3 antagonist and investigations on the balance between efficacy and tolerability (Table S2). At present, an ongoing global multicenter phase III clinical trial of low-dose gefapixant for treatment of refractory chronic cough has enrolled 2,000 subjects, and is expected to obtain positive efficacy results, representing an optimistic development for patients with refractory chronic cough (15). Moreover, studies by Smith et al. also found that gefapixant did not eliminate excessive cough completely (59) and failed to show a good response to patients with lower daily cough frequencies (14), which can be explained by the heterogeneity among patients with chronic refractory cough (60,61). There may exist other processes related to the cough hypersensitivity in refractory chronic cough patients rather than ATP-P2X pathways. Coughing of those subjects with poor efficacy may be mainly produced by hypersensitivity and continuous exposure to inhaled stimuli, rather than by endogenous ATP-dependent mechanisms (62).
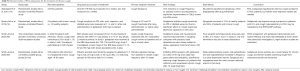
Full table
Novel P2X3R antagonists are also being explored. BLU-5937 is a non-competitive antagonist with high selectivity for P2X3R homotrimers. In guinea pig cough models, BLU-5937 significantly reduced the increase in cough episodes induced by histamine or ATP in a dose-dependent manner. Further, in rat models of behavioral taste response, BLU-5937 had no significant effect on taste, compared with control animals (63). BLU-5937 has excellent pharmaceutical properties, including high oral bioavailability, stable metabolism, no blood-brain barrier permeability, and good tolerability, and is currently in clinical phase I development. Besides, S-600918, a new antagonist, highly selective for P2X3 homomer compared with P2X2/3 heteromer, is expected to reduce cough frequency with minimal taste-related side effects in patients with refractory chronic cough (64). Molecules exhibiting greater selectivity for P2X3 compared with P2X2/3 are also under investigation, such as BAY 1817080 and BAY1902607 (42). The results of the above studies indicate that ATP may be involved in the formation of CHS, and show that P2X3R antagonists have a clear therapeutic effects on refractory chronic cough; however, in view of the high incidence of dysgeusia, further dose response studies are needed to achieve cough improvement at well-tolerated doses. Further, BLU-5937, as a non-competitive antagonist with high potency, selectivity for the P2X3R homotrimer, and excellent pharmaceutical properties for the treatment of chronic cough, strongly warrants additional investigation in clinical practice. Gefapixant, the P2X3 antagonist, is expected to be the first therapy approved for the treatment of refractory chronic cough. In addition, refractory chronic cough can be treated by therapies targeting other channels, such as neuromodulators and speech therapy, indicating that the pathogenesis of excess cough are unlikely occur independently, and coughing in different patients may be dominated by different mechanisms (42). Consequently, for the management of different patients with refractory chronic cough, their clinical characteristics must be analyzed to achieve accurate phenotyping in line with various preclinical and clinical researches to predict their potential underlying mechanisms and choose the optimal therapy.
Possible mechanism of the involvement of ATP in the etiology of CHS
ATP and TRPV1
TRPV1 is mainly expressed in primary afferent sensory neurons, smooth muscle cells of the lung, bronchial and small airway epithelial cells, and dendritic cells of the lung, and is involved in the activation of a variety of neuroinflammatory pathways (20,65), usually mediated by capsaicin. In addition, almost all major neurogenic inflammatory signaling pathways converge on TRPV1, to increase the excitability of C fibers during airway inflammation (21), and produce neurohypersensitivity, causing patients to respond to minimal stimulation, thus forming a vicious cycle and leading to therapeutic difficulties. Clinically, airway wall injury, due to mechanical shear stress generated during prolonged and repeated coughing in patients with refractory chronic cough, may increase ATP release into the airways (66,67), recruit inflammatory cells, and initiate or aggravate airway inflammation and remodeling (68). Extracellular ATP itself can directly depolarize vagal nerve fibers in animals and humans (57), with similar effects to capsaicin, producing transient but unsustained receptor potential pathways that sensitize vagal sensory fiber terminals in the lung, resulting in increased bronchoconstriction and cough reflex sensitivity, an effect that may be associated with activation of TRPV1 (8). In addition, Ma et al. found that ATP is involved in HCl-induced activation of TRPV1 receptors in the esophageal mucosa (69) and that TRPV1 can also mediate acid-stimulated ATP release from porcine bladder mucosa (70). ATP can interact with TRPV1, as demonstrated by studies in TRPV1-deficient mice, as it induces thermal hyperalgesia in wild-type, but not TRPV1-deficient, mice (71). TRPV1 expressed by C afferent vagal nerve fiber terminals is closely related to cough reflex sensitivity. During airway inflammation, a variety of inflammatory mediators can activate TRPV1, so that C afferent vagal nerve fiber neurolemma is rapidly depolarized, leading to excitement and enhancing cough reflex sensitivity. However, combined with the finding of Morice et al. that oral administration of the P2X3 receptor antagonist, AF-219/MK-7264, did not affect cough reflex sensitivity to either capsaicin or citric acid in patients with chronic cough (16), whether ATP-mediated changes in cough reflex sensitivity are related to TRPV1 is uncertain and further studies are warranted.
TRPV4 and ATP
TRPV4 is a non-selective calcium channel, widely expressed in the respiratory tract, including epithelium (human), macrophages (human and rat), and airway smooth muscles (human and guinea pig). It is also expressed in the trigeminal and dorsal root ganglia. A variety of physical and chemical stimuli, including mechanical stimulation, mild thermal stimulation above 24 °C, and arachidonic acid metabolites (72), can activate TRPV4. Further, TRPV4 can act as an osmotic sensor, to participate in cough development. Buday et al. also confirmed that TRPV4 mediates the cough response induced by citric acid and hypotonic solution (72-75). Activation of TRPV4 causes depolarization of vagal fibers and activation of Aδ, rather than C, fibers and the transmission of impulses to the nodose ganglia causes an increase in intracytoplasmic calcium concentration, which exacerbates cough, or causes airway smooth muscle contraction, in humans and animals; the jugular ganglion does not contribute to this process, and TRPV4 antagonists can reduce cough (75,76). Studies on human primary alveolar macrophages and bronchial epithelial cells exposed to cigarettes have shown that ATP release can be reduced using specific antagonists of TRPV1 or TRPV4, and that TRPV1 or TRPV4 agonists can directly induce ATP release (74). TRPV4 also mediates ATP release from human lung fibroblasts and subsequently acts on the purinergic receptor, P2YR, in an autocrine or paracrine manner (77).
Unlike the capsaicin-mediated cough reflex, extracellular ATP-mediated enhancement of the cough reflex is not attenuated by desensitization of C-fibers, suggesting that the mechanism by which ATP enhances the cough reflex may also involve Aδ-fibers (8). In a study by Morice et al., the P2X3R antagonist, gefapixant, was found to increase the cough threshold for inhalation of distilled water, without having a significant effect on cough threshold on inhalation of capsaicin or citric acid, which also suggests that ATP-related changes in cough reflex sensitivity may not be related to C fibers (16). Further, Gu et al. injected the potent TRPV4 agonist, GSK1016790A, into the right atrium of anesthetic rats with spontaneous breathing, causing rapid shallow breathing observed in anesthetized rats, an effect that could be abolished by the selective TRPV4 antagonist, GSK2193874, or vagotomy. Moreover, patch clamp experiments showed that GSK1016790A does not directly activate bronchopulmonary sensory neurons, possibly acting indirectly on TRPV4-expressing cells in the lung and airways to regulate respiration (72). In addition, Bonvini et al. found that TRPV4 agonists and hypotonic solutions can induce depolarization of isolated vagal and Aδ fibers (non-C fibers) in mouse, guinea pig, and human tissues, using calcium imaging techniques, in vivo electrophysiology, and in vivo animal models of cough. Further, this effect was blocked by TRPV4 or P2X3R antagonists, which have similar effects, while the TRPV4 agonist, GSK1016790A, caused slower activation of Aδ fibers than capsaicin and citric acid, indicating that TRPV4 activation has an indirect effect on Aδ fiber activation, rather than a direct effect (75). Hence, it is speculated that TRPV4 may mediate ATP-activation-induced depolarization of vagal fibers and activation of Aδ fibers.
Overall, we propose that endogenous ATP release and P2XR activation are prerequisites for the TRPV4-mediated cough reflex, and that TRPV4-ATP-P2X signaling may be the main driver of hypotonicity-induced airway afferent nerve fiber activation. Nevertheless, Buday et al. also found that TRPV4 antagonists partially inhibited citric acid-induced cough in guinea pigs, while having no effect on cough induced by distilled water and hypertonic fluid. TRPV4-mediated activation of airway afferent nerve fibers does not fully explain osmolarity-related cough (73). The mechanism underlying TRPV4-induced ATP release remains unclear and requires further study. However, a clinical research by Ludbrook et al. suggested that the selective TRPV4 channel blocker GSK2798745 had no effect on reducing cough frequency (78). Therefore, whether TRPV4 antagonists can be used as an alternative therapy to P2X3R antagonists for patients with refractory chronic cough remains to be validated.
Summary of mechanism of action
In summary, ATP is released into the extracellular space during airway inflammation, where it binds to corresponding P2 receptors to mediate the cough reflex and participate in the formation of cough hypersensitivity. The underlying mechanism may be related to TRPV4-mediated activation of Aδ afferent vagal fibers. Periodic force, generated during activation of TRPV4 (for example by hypotonic solution, low PH, citric acid, or inflammatory metabolites) or cough, damages airway epithelium and causes it to release a large amount of ATP, thereby stimulating P2X receptors on activated cough receptors, producing signal transduction, and further activating Aδ afferent vagal fibers. Nerve impulse transmission to nodose ganglia causes increased intracytoplasmic calcium concentration. The conduction of peripheral stimuli to the cough center will result in increased cough reflex sensitivity, further inducing TRPV1 expression in airway sensory nerve fiber terminals. In addition, ATP may also promote inflammatory cells to release inflammatory factors or act directly on P2 receptors on a few nodose ganglion C fibers, producing neurogenic inflammation and activating TRPV1 in vagal nerve endings, thereby generating a vicious cycle of increased cough reflex sensitivity, rendering the cough difficult to cure.
Future prospects
The treatment of refractory chronic cough remains a common medical problem facing clinicians. Although a number of studies have verified the effectiveness of P2X3R for the treatment of refractory chronic cough, the high incidence of the adverse reaction of dysgeusia limits the sample size of clinical trials. Further, the optimal dose, treatment cycle, underlying mechanism of action, and safety of the long-term application of P2X3R for the treatment of refractory chronic cough are unclear. A global multicenter study is ongoing. New drugs, highly specific for P2X3R targets, need to be studied and developed. Simultaneously, basic research to further explore the specific mechanism underlying the involvement of ATP in the etiology of cough hypersensitivity is warranted, to provide theoretical support for the clinical research.
Acknowledgments
Funding: This study was supported by the National Natural Science Foundation of China (No. 81770097 and 81670092), the Project of Science and Technology Commission of Shanghai Municipality (No. 17411970800), the Fund of Shanghai Youth Talent Support Program, and the Fund of Shanghai Municipal Health Commission for Excellent Young Scholars (No. 2018YQ01).
Footnote
Provenance and Peer Review: This article was commissioned by the Guest Editors (Woo-Jung Song) for the series “Cough Section” published in Journal of Thoracic Disease. The article was sent for external peer review organized by the Guest Editor and the editorial office.
Conflicts of Interest: All authors have completed the ICMJE uniform disclosure form (available at http://dx.doi.org/10.21037/jtd-20-cough-001). The series “Cough Section” was commissioned by the editorial office without any funding or sponsorship. The authors have no other conflicts of interest to declare.
Ethical Statement: The authors are accountable for all aspects of the work in ensuring that questions related to the accuracy or integrity of any part of the work are appropriately investigated and resolved.
Open Access Statement: This is an Open Access article distributed in accordance with the Creative Commons Attribution-NonCommercial-NoDerivs 4.0 International License (CC BY-NC-ND 4.0), which permits the non-commercial replication and distribution of the article with the strict proviso that no changes or edits are made and the original work is properly cited (including links to both the formal publication through the relevant DOI and the license). See: https://creativecommons.org/licenses/by-nc-nd/4.0/.
References
- Pratter MR. Overview of common causes of chronic cough: ACCP evidence-based clinical practice guidelines. Chest 2006;129:59S-62S. [Crossref] [PubMed]
- Morice AH, Millqvist E, Bieksiene K, et al. ERS guidelines on the diagnosis and treatment of chronic cough in adults and children. Eur Respir J 2020;55:1901136. [PubMed]
- Yu L, Chen Q, Qiu Z. Diagnosis and treatment of refractory cough. Zhonghua Jie He He Hu Xi Za Zhi 2016;39:383-6. [PubMed]
- Subspecialty Group of Respiratory Diseases Society of Asthma CMA. Guidelines for the diagnosis and treatment of cough(2015). Zhonghua Jie He He Hu Xi Za Zhi 2016;39:323-54.
- Morice AH. The cough hypersensitivity syndrome: a novel paradigm for understanding cough. Lung 2010;188:S87-90. [Crossref] [PubMed]
- Kouzaki H, Iijima K, Kobayashi T, et al. The danger signal, extracellular ATP, is a sensor for an airborne allergen and triggers IL-33 release and innate Th2-type responses. J Immunol 2011;186:4375-87. [Crossref] [PubMed]
- Chen H, Xia Q, Feng X, et al. Effect of P2X4R on airway inflammation and airway remodeling in allergic airway challenge in mice. Mol Med Rep 2016;13:697-704. [Crossref] [PubMed]
- Pelleg A, Schulman ES, Barnes PJ. Extracellular Adenosine 5'-Triphosphate in Obstructive Airway Diseases. Chest 2016;150:908-15. [Crossref] [PubMed]
- Ford AP, Undem BJ. The therapeutic promise of ATP antagonism at P2X3 receptors in respiratory and urological disorders. Front Cell Neurosci 2013;7:267. [Crossref] [PubMed]
- Basoglu OK, Pelleg A, Essilfie-Quaye S, et al. Effects of aerosolized adenosine 5'-triphosphate vs adenosine 5'-monophosphate on dyspnea and airway caliber in healthy nonsmokers and patients with asthma. Chest 2005;128:1905-09. [Crossref] [PubMed]
- Kamei J, Takahashi Y, Yoshikawa Y, et al. Involvement of P2X receptor subtypes in ATP-induced enhancement of the cough reflex sensitivity. Eur J Pharmacol 2005;528:158-61. [Crossref] [PubMed]
- Fowles HE, Rowland T, Wright C, et al. Tussive challenge with ATP and AMP: does it reveal cough hypersensitivity? Eur Respir J 2017;49:1601452. [Crossref] [PubMed]
- Richards D, Gever JR, Ford AP, et al. Action of MK-7264 (gefapixant) at human P2X3 and P2X2/3 receptors and in vivo efficacy in models of sensitisation. Br J Pharmacol 2019;176:2279-91. [PubMed]
- Abdulqawi R, Dockry R, Holt K, et al. P2X3 receptor antagonist (AF-219) in refractory chronic cough: a randomised, double-blind, placebo-controlled phase 2 study. Lancet 2015;385:1198-205. [Crossref] [PubMed]
- Muccino D, Green S. Update on the clinical development of gefapixant, a P2X3 receptor antagonist for the treatment of refractory chronic cough. Pulm Pharmacol Ther 2019;56:75-8. [Crossref] [PubMed]
- Morice AH, Kitt MM, Ford AP, et al. The effect of gefapixant, a P2X3 antagonist, on cough reflex sensitivity: a randomised placebo-controlled study. Eur Respir J 2019;54. [Crossref] [PubMed]
- Lai K, Fang Z, Yao H. Cough hypersensitivity syndrome: a new concept for chronic idiopathic cough. Jie Fang Jun Yi Xue Za Zhi 2014;39:343-9.
- Xia Y, Xia L, Lou L, et al. Transient Receptor Potential Channels and Chronic Airway Inflammatory Diseases: A Comprehensive Review. Lung 2018;196:505-16. [Crossref] [PubMed]
- Bessac BF, Jordt SE. Breathtaking TRP channels: TRPA1 and TRPV1 in airway chemosensation and reflex control. Physiology (Bethesda) 2008;23:360-70. [Crossref] [PubMed]
- Colsoul B, Nilius B, Vennekens R. On the putative role of transient receptor potential cation channels in asthma. Clin Exp Allergy 2009;39:1456-66. [Crossref] [PubMed]
- Lee LY, Hsu CC, Lin YJ, et al. Interaction between TRPA1 and TRPV1: Synergy on pulmonary sensory nerves. Pulm Pharmacol Ther 2015;35:87-93. [Crossref] [PubMed]
- Lalloo UG, Fox AJ, Belvisi MG, et al. Capsazepine inhibits cough induced by capsaicin and citric acid but not by hypertonic saline in guinea pigs. J Appl Physiol 1995;79:1082-7. [Crossref] [PubMed]
- Xu X, Chen Q, Qiu Z, et al. Association of cough hypersensitivity with tracheal TRPV1 activation and neurogenic inflammation in a novel guinea pig model of citric acid-induced chronic cough. J Int Med Res 2018;46:2913-24. [Crossref] [PubMed]
- Groneberg DA, Niimi A, Dinh QT, et al. Increased expression of transient receptor potential vanilloid-1 in airway nerves of chronic cough. Am J Respir Crit Care Med 2004;170:1276-80. [Crossref] [PubMed]
- O'Connell F, Thomas VE, Studham JM, et al. Capsaicin cough sensitivity increases during upper respiratory infection. Respir Med 1996;90:279-86. [Crossref] [PubMed]
- Fujimura M, Kamio Y, Kasahara K, et al. Prostanoids and cough response to capsaicin in asthma and chronic bronchitis. Eur Respir J 1995;8:1499-505. [PubMed]
- Satia I, Tsamandouras N, Holt K, et al. Capsaicin-evoked cough responses in asthmatic patients: Evidence for airway neuronal dysfunction. J Allergy Clin Immunol 2017;139:771-9 e10.
- Doherty MJ, Mister R, Pearson MG, et al. Capsaicin responsiveness and cough in asthma and chronic obstructive pulmonary disease. Thorax 2000;55:643-9. [Crossref] [PubMed]
- Blanc FX, Macedo P, Hew M, et al. Capsaicin cough sensitivity in smokers with and without airflow obstruction. Respir Med 2009;103:786-90. [Crossref] [PubMed]
- Hope-Gill BD, Hilldrup S, Davies C, et al. A study of the cough reflex in idiopathic pulmonary fibrosis. Am J Respir Crit Care Med 2003;168:995-1002. [Crossref] [PubMed]
- Belvisi MG, Birrell MA, Khalid S, et al. Neurophenotypes in Airway Diseases. Insights from Translational Cough Studies. Am J Respir Crit Care Med 2016;193:1364-72. [Crossref] [PubMed]
- Prudon B, Birring SS, Vara DD, et al. Cough and glottic-stop reflex sensitivity in health and disease. Chest 2005;127:550-7. [Crossref] [PubMed]
- Dicpinigaitis PV, Dobkin JB, Reichel J. Antitussive effect of the leukotriene receptor antagonist zafirlukast in subjects with cough-variant asthma. J Asthma 2002;39:291-7. [Crossref] [PubMed]
- Fukumitsu K, Kanemitsu Y, Asano T, et al. Tiotropium Attenuates Refractory Cough and Capsaicin Cough Reflex Sensitivity in Patients with Asthma. J Allergy Clin Immunol Pract 2018;6:1613-20.e2. [Crossref] [PubMed]
- Pretolani M, Bergqvist A, Thabut G, et al. Effectiveness of bronchial thermoplasty in patients with severe refractory asthma: Clinical and histopathologic correlations. J Allergy Clin Immunol 2017;139:1176-85. [Crossref] [PubMed]
- Gunthorpe MJ, Chizh BA. Clinical development of TRPV1 antagonists: targeting a pivotal point in the pain pathway. Drug Discov Today 2009;14:56-67. [Crossref] [PubMed]
- Delescluse I, Mace H, Adcock JJ. Inhibition of airway hyper-responsiveness by TRPV1 antagonists (SB-705498 and PF-04065463) in the unanaesthetized, ovalbumin-sensitized guinea pig. Br J Pharmacol 2012;166:1822-32. [Crossref] [PubMed]
- Khalid S, Murdoch R, Newlands A, et al. Transient receptor potential vanilloid 1 (TRPV1) antagonism in patients with refractory chronic cough: A double-blind randomized controlled trial. J Allergy Clin Immunol 2014;134:56-62. [Crossref] [PubMed]
- Belvisi MG, Birrell MA, Wortley MA, et al. XEN-D0501, a Novel Transient Receptor Potential Vanilloid 1 Antagonist, Does Not Reduce Cough in Patients with Refractory Cough. Am J Respir Crit Care Med 2017;196:1255-63. [Crossref] [PubMed]
- Burnstock G, Brouns I, Adriaensen D, et al. Purinergic signaling in the airways. Pharmacol Rev 2012;64:834-68. [Crossref] [PubMed]
- Guo HM, Luo YL, Zhou WL. The role of extralcellular ATP in the airway. Sheng Li Ke Xue Jin Zhan 2010;41:189-92. [PubMed]
- Smith JA, Badri H. Cough: New Pharmacology. J Allergy Clin Immunol Pract 2019;7:1731-8. [Crossref] [PubMed]
- Burnstock G. Purine and pyrimidine receptors. Cell Mol Life Sci 2007;64:1471-83. [Crossref] [PubMed]
- Li H, Ji H, Hu Q. Advances in research on the function of purinergic receptor P2Y in immune inflammation. J Pharm Res 2019;38:218-24.
- Irwin RS, Ownbey R, Cagle PT, et al. Interpreting the histopathology of chronic cough: a prospective, controlled, comparative study. Chest 2006;130:362-70. [Crossref] [PubMed]
- Shi C, Qiu Z. Effect of airway inflammation on chronic cough. Int J Respir 2007;27:773-6.
- Esther CR Jr, Alexis NE, Clas ML, et al. Extracellular purines are biomarkers of neutrophilic airway inflammation. Eur Respir J 2008;31:949-56. [Crossref] [PubMed]
- Weigand LA, Ford AP, Undem BJ. A role for ATP in bronchoconstriction-induced activation of guinea pig vagal intrapulmonary C-fibres. J Physiol 2012;590:4109-20. [Crossref] [PubMed]
- Pelleg A, Hurt C. Mechanism of action of ATP on canine pulmonary vagal C fibre nerve terminals. J Physiol 1996;490:265-75. [Crossref] [PubMed]
- Hao Y, Liang JF, Chow AW, et al. P2Y6 receptor-mediated proinflammatory signaling in human bronchial epithelia. PLoS One 2014;9:e106235. [Crossref] [PubMed]
- Chávez J, Vargas MH, Martinez-Zuniga J, et al. Allergic sensitization increases the amount of extracellular ATP hydrolyzed by guinea pig leukocytes. Purinergic Signal 2019;15:69-76. [Crossref] [PubMed]
- Chávez J, Vargas MH, Rebollar-Ayala DC, et al. Inhibition of extracellular nucleotides hydrolysis intensifies the allergic bronchospasm. A novel protective role of ectonucleotidases. Allergy 2013;68:462-71. [Crossref] [PubMed]
- Montaño LM, Vargas MH, Diaz-Hernandez V, et al. Decreased expression of ectonucleotidase E-NPP1 in leukocytes from subjects with severe asthma exacerbation. Allergy 2016;71:124-8. [Crossref] [PubMed]
- Schulman ES, Glaum MC, Post T, et al. ATP modulates anti-IgE-induced release of histamine from human lung mast cells. Am J Respir Cell Mol Biol 1999;20:530-7. [Crossref] [PubMed]
- Pinheiro AR, Paramos-de-Carvalho D, Certal M, et al. Histamine induces ATP release from human subcutaneous fibroblasts, via pannexin-1 hemichannels, leading to Ca2+ mobilization and cell proliferation. J Biol Chem 2013;288:27571-83. [Crossref] [PubMed]
- Kamei J, Takahashi Y. Involvement of ionotropic purinergic receptors in the histamine-induced enhancement of the cough reflex sensitivity in guinea pigs. Eur J Pharmacol 2006;547:160-4. [Crossref] [PubMed]
- Song W-J, Morice AH. Cough Hypersensitivity Syndrome: A Few More Steps Forward. Allergy Asthma Immunol Res 2017;9:394-402. [Crossref] [PubMed]
- Smith JA, Kitt MM, Butera P, et al. Gefapixant in two randomised dose-escalation studies in chronic cough. Eur Respir J 2020;55. [Crossref] [PubMed]
- Smith JA, Kitt MM, Morice AH, et al. Gefapixant, a P2X3 receptor antagonist, for the treatment of refractory or unexplained chronic cough: a randomised, double-blind, controlled, parallel-group, phase 2b trial. Lancet Respir Med 2020. [Epub ahead of print]. [Crossref] [PubMed]
- Mazzone SB, Chung KF, McGarvey L. The heterogeneity of chronic cough: a case for endotypes of cough hypersensitivity. The Lancet Respiratory Medicine 2018;6:636-46. [Crossref] [PubMed]
- Mazzone SB, Farrell MJ. Heterogeneity of cough neurobiology: Clinical implications. Pulm Pharmacol Ther 2019;55:62-6. [Crossref] [PubMed]
- Turner RD, Birring SS. Chronic cough: ATP, afferent pathways and hypersensitivity. Eur Respir J 2019;54. [Crossref] [PubMed]
- Garceau D, Chauret N. BLU-5937: A selective P2X3 antagonist with potent anti-tussive effect and no taste alteration. Pulm Pharmacol Ther 2019;56:56-62. [Crossref] [PubMed]
- Niimi A, Ishihara H, Hida H, et al. Late Breaking Abstract - Phase 2a randomised, double-blind, placebo-controlled, crossover study of a novel P2X3 receptor antagonist S-600918 in patients with refractory chronic cough. Eur Respir J 2019;54:RCT452.
- Couto M. Cough Reflex Testing With Inhaled Capsaicin and TRPV1 Activation in Asthma and Comorbid Conditions. J Investig Allergol Clin Immunol 2013;23:289-301. [PubMed]
- Button B, Picher M, Boucher RC. Differential effects of cyclic and constant stress on ATP release and mucociliary transport by human airway epithelia. J Physiol 2007;580:577-92. [Crossref] [PubMed]
- Takahara N, Ito S, Furuya K, et al. Real-time imaging of ATP release induced by mechanical stretch in human airway smooth muscle cells. Am J Respir Cell Mol Biol 2014;51:772-82. [Crossref] [PubMed]
- Niimi A. Structural changes in the airways: cause or effect of chronic cough? Pulm Pharmacol Ther 2011;24:328-33. [Crossref] [PubMed]
- Ma J, Altomare A, Rieder F, et al. ATP: a mediator for HCl-induced TRPV1 activation in esophageal mucosa. Am J Physiol Gastrointest Liver Physiol 2011;301:G1075-82. [Crossref] [PubMed]
- Sadananda P, Kao FC, Liu L, et al. Acid and stretch, but not capsaicin, are effective stimuli for ATP release in the porcine bladder mucosa: Are ASIC and TRPV1 receptors involved? Eur J Pharmacol 2012;683:252-9. [Crossref] [PubMed]
- Tominaga M, Moriyama T. Functional Interaction Between ATP and TRPV1 Receptors. In: Wang DH (eds) Molecular Sensors for Cardiovascular Homeostasis. Springer, Boston, MA 2003:133-40.
- Gu Q, Moss CR, Kettelhut KL, et al. Activation of TRPV4 Regulates Respiration through Indirect Activation of Bronchopulmonary Sensory Neurons. Front Physiol 2016;7:65. [Crossref] [PubMed]
- Buday T, Kovacikova L, Ruzinak R, et al. TRPV4 antagonist GSK2193874 does not modulate cough response to osmotic stimuli. Respir Physiol Neurobiol 2017;236:1-4. [Crossref] [PubMed]
- Baxter M, Eltom S, Dekkak B, et al. Role of transient receptor potential and pannexin channels in cigarette smoke-triggered ATP release in the lung. Thorax 2014;69:1080-9. [Crossref] [PubMed]
- Bonvini SJ, Birrell MA, Grace MS, et al. Transient receptor potential cation channel, subfamily V, member 4 and airway sensory afferent activation: Role of adenosine triphosphate. J Allergy Clin Immunol 2016;138:249-61.e12. [Crossref] [PubMed]
- McAlexander MA, Luttmann MA, Hunsberger GE, et al. Transient receptor potential vanilloid 4 activation constricts the human bronchus via the release of cysteinyl leukotrienes. J Pharmacol Exp Ther 2014;349:118-25. [Crossref] [PubMed]
- Rahman M, Sun R, Mukherjee S, et al. TRPV4 Stimulation Releases ATP via Pannexin Channels in Human Pulmonary Fibroblasts. Am J Respir Cell Mol Biol 2018;59:87-95. [Crossref] [PubMed]
- Ludbrook V, Hanrott K, Marks-Konczalik J, et al. S27 A placebo-controlled, double-blind, randomised, crossover study to assess the efficacy, safety and tolerability of TRPV4 inhibitor GSK2798745 in participants with chronic cough. Thorax 2019;74:A18.
- Fredholm BB. International Union of Basic and Clinical Pharmacology. LXXXI. Nomenclature and classification of adenosine receptors--an update. Pharmacol Rev 2011;63:1-34. [Crossref] [PubMed]
- Merighi S, Bencivenni S, Vincenzi F, et al. A2B adenosine receptors stimulate IL-6 production in primary murine microglia through p38 MAPK kinase pathway. Pharmacol Res 2017;117:9-19. [Crossref] [PubMed]
- Schwiebert EM, Zsembery A. Extracellular ATP as a signaling molecule for epithelial cells. Biochim Biophys Acta 2003;1615:7-32. [Crossref] [PubMed]
- Biber K, Tsuda M, Tozaki-Saitoh H, et al. Neuronal CCL21 up-regulates microglia P2X4 expression and initiates neuropathic pain development. EMBO J 2011;30:1864-73. [Crossref] [PubMed]
- Winkelmann VE, Thompson KE, Neuland K, et al. Inflammation-induced upregulation of P2X4 expression augments mucin secretion in airway epithelia. Am J Physiol Lung Cell Mol Physiol 2019;316:L58-L70. [Crossref] [PubMed]
- Collo G, North R, Kawashima E, et al. Cloning OF P2X5 and P2X6 receptors and the distribution and properties of an extended family of ATP-gated ion channels. J Neurosci 1996;76:2495-507. [Crossref] [PubMed]
- Lucattelli M, Cicko S, Muller T, et al. P2X7 receptor signaling in the pathogenesis of smoke-induced lung inflammation and emphysema. Am J Respir Cell Mol Biol 2011;44:423-9. [Crossref] [PubMed]
- Cicko S, Lucattelli M, Muller T, et al. Purinergic receptor inhibition prevents the development of smoke-induced lung injury and emphysema. J Immunol 2010;185:688-97. [Crossref] [PubMed]
- Jung SM, Moroi M. Platelet collagen receptor integrin alpha2beta1 activation involves differential participation of ADP-receptor subtypes P2Y1 and P2Y12 but not intracellular calcium change. Eur J Biochem 2001;268:3513-22. [Crossref] [PubMed]
- Wildman SS, Unwin RJ, King BF. Extended pharmacological profiles of rat P2Y2 and rat P2Y4 receptors and their sensitivity to extracellular H+ and Zn2+ ions. Br J Pharmacol 2003;140:1177-86. [Crossref] [PubMed]
- Di Virgilio F, Vuerich M. Purinergic signaling in the immune system. Auton Neurosci 2015;191:117-23. [Crossref] [PubMed]
- Cekic C, Linden J. Purinergic regulation of the immune system. Nat Rev Immunol 2016;16:177-92. [Crossref] [PubMed]
- Elliott MR, Chekeni FB, Trampont PC, et al. Nucleotides released by apoptotic cells act as a find-me signal to promote phagocytic clearance. Nature 2009;461:282-6. [Crossref] [PubMed]