Bronchoscopic light delivery method for peripheral lung cancer photodynamic therapy
Introduction
Lung cancer has become the most common and fatal cancer owing to smoking and increasing industrial air pollution, and its incidence and mortality rate continue to increase worldwide. The United States National Lung Screening Trial confirmed that low-dose computed tomography (LDCT) could detect early stage lung cancer, and this could effectively reduce the mortality rate and increase the overall survival rate among patients suffering from lung cancer in their study population (1). The increasing use of LDCT has led to the increased detection of patients with early stage lung tumors.
Surgical resection is currently the gold standard treatment for early stage lung cancer. However, minimally invasive surgery still has inherent surgical risks, and thus the effectiveness of less invasive treatments such as radiation therapy, percutaneous ablation therapy, and trans-bronchoscopy ablation treatment which eliminate the risks associated with surgery and reduce normal lung tissue damage while achieving favorable outcomes for early stage lung tumors have been investigated (2).
In photodynamic therapy (PDT), photosensitizers and light are used to destroy cancer cells. Specifically, photosensitizers are selectively absorbed by cancer cells, and light is given to activate absorbed photosensitizers and destroy cancer cells (3). Some clinical trials investigated the use of PDT to treat peripheral lung tumors. In 2004, Okunaka et al. proposed using PDT through a percutaneous puncture method to treat peripheral lung tumors (4). Under the guidance of computed tomography (CT), multiple therapeutic fibers were placed to illuminate the inner part of the tumor. There were no serious complications associated with this method, and good therapeutic effect was achieved for small tumors. Three clinical trials [Usuda et al. in Japan (5), Chen et al. at the National Taiwan University in 2017 and 2018 (6), and the Concordia Corporation in the United States (7)] have also confirmed the feasibility of bronchoscopy-guided PDT for early stage (T1: less than 3 cm) peripheral lung tumors. However, the PDT light can penetrate the tissue only until approximately 0.7 to 1 cm, limiting its application and outcomes (8). Despite the availability of advanced imaging modalities, tumor localization and navigation are not widely available in clinical practice because of the need for specialized training.
Light-tissue interactions including absorption, scattering, refraction, and reflection limit the depth of light penetration in the tissue. In 2003, Friedberg et al. proposed a novel illumination model by infusing high-refractive-index (RI) substances such as mineral oil into the trachea and bronchi (9). The RI of mineral oil, alveolar tissue, air, and water are approximately 1.46, 1.37, 1.0, and 1.33, respectively. Light reflects in the trachea and bronchi filled with mineral oil and creates the optic-fiber transmission effect. Thus, light can be transmitted to distant tissues in the alveolar branches and bronchi without being restricted by the straight-line movement; thereby, forming an illuminated area in the tissues filled with the mineral oil. This novel illumination model has the potential to increase the range of illumination in PDT and expand its use for the treatment of other organs with luminal structures such as the intestine, biliary tract, pancreas, urinary tract, abdominal cavity, and even cerebrospinal vertebra, where fibers and sheaths can be placed through angiography to the tumor site. However, mineral oil has only been clinically approved for oral or anal enemas as a laxative and some clinical studies have reported that mineral oil accidentally inhaled into the airway can cause severe aspiration lipoid pneumonia (10). Thus, ensuring its safety in this bronchoscopic light delivery model is challenging.
The RI of lipiodol is approximately 1.49, which is higher than that of mineral oil with an RI of 1.46. Physically, lipiodol will have a better light-pipe effect than mineral oil in this light delivery model. Additionally, lipiodol is a contrast medium that can be inspected under a fluoroscope to ensure that it has fully covered the target tumor, ensuring complete illumination of the tumor. In this novel PDT model, lipiodol could be used instead of mineral oil.
Lipiodol is an iodinated poppy seed oil first synthesized in 1901. It was first used clinically as a radiographic agent in 1921 by Forestier. Since then, lipiodol has been widely used as the contrast medium for bronchography for almost 50 years (11,12). In recent years, lipiodol has been used in lymphography and embolization treatment for liver tumors as well as for locating lung tumors using radiography via endobronchial infusion (13,14).
Literature has indicated that the use of lipiodol as a contrast medium for bronchography has some disadvantages; it can easily spill into the alveolar space where it is retained in the pulmonary parenchyma. A high incidence of acute pneumonia and a granulomatous reaction to the oil also occurs occasionally (11,12). Hence, lipiodol for bronchography was then abandoned after the invention of the flexible bronchoscope and CT. However, these disadvantages were turned to advantages in this novel model. The spilling of lipiodol into the alveolar space and its retention in the lung parenchyma for a period of time indicates that lipiodol can almost completely occupy the air space (bronchus and alveolar) in the infused area without a significant air-oil interface, which might decrease the effect of light delivery as a light pipe. Lipiodol is viscous and the retention at the infusion area for a period of time without spillage to other non-target regions gives adequate time for ongoing PDT treatment. Compared to other oils tested for bronchography that were reviewed in the literature, lipiodol is less damaging to the lungs, and cases of acute pneumonia were resolved in days without severe consequences (15). Furthermore, a literature review revealed no serious adverse events associated with the use of less than 20 mL of lipiodol infusion through bronchoscopy (12). Although there were some reports of a granulomatous reaction, it was very rare. For this reason, lipiodol was used for almost 50 years as the contrast medium in bronchography.
Five-aminolevulinic acid (5-ALA) is a natural amino acid that is a common precursor of hemoglobin and chlorophyll. Exogenously administered 5-ALA leads to the biosynthesis of protoporphyrin IX (PpIX), the last precursor of heme, in the mitochondria. PpIX is then catalyzed by ferrochelatase and binds with ferrous ion resulting in the production of heme. The ferrochelatase is inactive in various cancer cells, resulting in excess accumulation of PpIX. Since PpIX has photoactivity, excitation at certain wavelengths of light, particularly red light (600–740 nm) and green light (450–580 nm) at low power, allows the cell to absorb light energy and generate photochemical reactions as well as induce apoptosis. 5-ALA is eliminated quickly with a terminal half-life of 1–3 hours. Approximately 30% of an orally administered dose of 20 mg/kg body weight is excreted unchanged in urine within 12 hours. This is the principle on which ALA-PDT is based (16,17).
Although 5-ALA is not currently used in the clinical treatment of lung cancer, it can be used in the photodynamic diagnosis and treatment of brain cancer, esophageal cancer, skin cancer, cervical cancer, and bladder cancer. The Food and Drug Administration (FDA) has approved 5-ALA for brain tumor photodynamic diagnosis during operations performed to detect possible residual tumors; a dosage of 20 mg/kg of 5-ALA was given 2 hours before an operation (16,17). In current clinical medicine, porfimer sodium (Photofrin) is used in in lung cancer PDT. However, 5-ALA has a shorter half-life, can be administered orally and study subjects need to be kept away from light for only 5 hours post-oral administration unlike for 1 month when Photofrin is used. Because of these advantages, the use of 5-ALA is more convenient than that of Photofrin for animal experiments. The main purpose of this experiment was to test the feasibility of using a bronchoscope to navigate and inject lipiodol under imaging equipment. Will there be fatal side effects caused by lipiodol? The possible effects are based on the next stage of testing the relationship between the drug and the light intensity. Therefore, the 5-ALA drug, which is easier to experimentally operate, was used in our experiment. The 5-ALA drug is also a potential photosensitive drug, and it is worth further development and testing.
We hypothesized that lipiodol can be used as a high RI solution in our photodynamic light delivery model based on the model proposed by Friedberg et al. (9), and lipiodol does not cause severe acute fatal lung damage in this treatment mode. To test this hypothesis, we developed a pig model to explore first, whether the bronchoscopy technique was feasible and then, to identify the appropriate light dose that can be tolerated in a healthy lung without severe damage, to determine the maximal toxic dose (MTD) of light to be used in future human clinical trials.
Methods
Development of the pig model
Since there is currently no bronchoscope and image navigation equipment for small animals, we could not use a small animal as a lung cancer model for in vivo testing, and thus pigs were chosen for this study. A pig lung cancer model is difficult to establish. We first tried to find the MTD of light tolerated by a healthy lung, as this light dose will be adapted in future human clinical trials.
All animal experiments were conducted in the laboratory of Pigmodel Animal Technology Company in Taiwan. The laboratory is AAALAC certified (Certification No. 001636); it has a C-arm fluoroscope, X-ray radiographic equipment, and anesthetic operation equipment.
The pigs used in the tests were 2-year-old Lanyu miniature pigs and they weighed 50 kg each. All applicable international, national, and/or institutional guidelines for the care and use of animals were followed. The animal use protocol was reviewed and approved by the Institution of Animal Care and Use Committee (IACUC) of Pigmodel Animal Technology Co., Ltd. (Approval No. PIG-107007).
Equipment and substances
A Diomed 630 nm laser (Biolitec) with fiber, a frontal light source, and side light source fiber was used. The following hardware models were used: for a frontal light distributor; Model FD (Biolitec, UMDNS 17193), cylindrical light diffuser; Model RD-ML (Biolitec, UMDNS 17193), bronchoscope (Fujifilm EB-530S) with a 2.0 mm working channel, guide sheath (Olympus guide sheath K201) and C-arm X-ray fluoroscope (GE OEC Fluorostar 7900 series). The RI value of lipiodol was tested using an Abbemat Wavelength Refractometer (Anton Paar) from the Department of Chemical Engineering of Chung Yuan Christian University, Taoyuan City, Taiwan. We purchased 5-ALA and Lipiodol® Ultra Fluid from Sigma-Aldrich Co. and Guerbet Co., respectively.
In vivo pig lung test
Two in vivo tests were conducted. The purpose of the first test was to verify the feasibility of the technique and identify the safety of lipiodol infusion to the lung tissue and a safe light dose if possible. After cleaning and drying, the pigs were sedated via an intramuscular injection of 3–5 mg/kg azeperonum (40 mg/mL, China Chemical & Pharmaceutical Co., Ltd.) and 0.03–0.05 mg/kg atropine (1 mg/mL, Taiwan Biotech Co., Ltd.), with monitoring of the respiratory rate. After 20 to 30 minutes, anesthesia was introduced via an intramuscular injection of Zoletil®-50 at a dose of 3–5 mg/kg. After 5–10 minutes, the pigs were then moved to the operating table, placed in a prone position for bronchial intubation, and then connected to the anesthesia machine. Then, the pig bronchi were intubated and inflated with oxygen/nitrous oxide gas (2:1), which was further mixed with 0.5–2% isoflurane (from Panion & BF Biotech, Inc.) at a rate of 2–3 L/min. The depth of anesthesia was monitored throughout the procedure.
Using an orogastric tube, the pig was fed with 5-ALA (20 mg/kg) 2 hours before the procedure. After general anesthesia with endobronchial intubation, the guide sheath was placed in the peripheral bronchi under bronchoscope guidance, and the tip position of the sheath was confirmed via X-ray fluoroscopy.
Four different parts were selected on both the left and right sides of the pig lung. Under X-ray fluoroscopy guidance, a guide sheath was inserted through the bronchoscope to the selected peripheral lung region (outer 2/3 of the lung field), and 5 mL lipiodol was infused slowly into each selected part of the lung under careful inspection with the fluoroscope. These selected lung segments were located in different main bronchial trees to avoid spillage contamination of the infused lipiodol. Following this, the frontal light distributors were placed in the bronchi through the guide sheath to the target lung region under the surveillance of fluoroscopy. We could see the tip of the frontal light distributer inserted outside the tip of the guide sheath by pre-measured insertion fiber length under the fluoroscope, although there was no radiopaque marker at the tip of the light distributor. Four different light doses were given for the test in order to find the MTD: no light (control), 400 mW 500 s (200 J), 400 mW 1,000 s (400 J), and 800 mW 1,000 s (800 J). After the operation, the pig was cared for according to the standard animal care protocol. Chest radiography examinations and basic blood biochemical tests were performed on the next day and once weekly thereafter. The pig was sacrificed after 1 month for pathological examination of the treated lung.
For the second test, the purpose was to verify the feasibility and safety of the MTD of light identified in the first test. Thus, we repeated the steps in the first test. The pig was fed with 5-ALA (20 mg/kg) 2 hours before treatment, using an orogastric tube and general anesthesia with endobronchial intubation was introduced. However, in this experiment, only four different parts of the lung were selected (two on each side). We then infused 5 mL lipiodol into each selected part of the lung through the bronchoscope guide sheath. One part of the lung was used as the control group with no light, and in the other three parts the tests were repeated for the highest safe lighting dose. Chest radiography and basic blood biochemical tests were performed the next day and once weekly thereafter. The pig was sacrificed after 1 month of survival, as we did after the first test.
Ex vivo pig lung test
Since a high energy output might induce overheating at the tip of the frontal optic fiber, we presumed that a cylindrical optic fiber with a reduced energy output may prevent the risk of overheating and could provide a similar total energy output. We used a 5 cm cylindrical fiber with an energy output of 200 J/cm (1,000 J in total) in an ex vivo lung model, in which we also infused 5 mL lipiodol to test if this cylindrical fiber and energy output would induce overheating in the fiber.
The lungs used in the ex vivo pig lung test were from pigs sacrificed during other trials in Pigmodel Animal Technology Company. After the pigs were sacrificed, we immediately harvested the lungs and the whole bronchi for our trial. The trachea was clamped to avoid water inflow into the bronchi after harvest. We soaked the lungs in 4 °C normal saline, covered them with gauze to moisturize, and immediately ran the test within 4 hours of sacrifice. An endobronchial tube was inserted into the bronchi and was tied tightly to avoid air leakage. The endobronchial tube was connected using a 3-way connector to an Ambu bag. The bronchoscope was connected to the connector for the test.
Using X-ray fluoroscopy imaging, the guide sheath was placed in four different lung locations via bronchoscopy, and 5 mL lipiodol was instilled into each of the four different parts of the lung. Five-centimeter cylindrical optical fibers were placed in each part of the lung through the therapeutic guide sheath. Once the illumination end of the optical fiber reached the parts infused with lipiodol, 200 J/cm energy was provided to verify whether the illumination energy would interact with lipiodol to cause damage.
Results
RI value of lipiodol
At 20, 25, and 37 °C, the RI value of lipiodol was 1.5025, 1.5005, and 1.4956, respectively.
In vivo pig test
Verifying feasibility and identifying safe light dose
Using X-ray fluoroscopy, we confirmed that lipiodol could enter the bronchi by placing the guide sheath via bronchoscopy. The lipiodol instilled into the bronchi created a wedge shape area. Infusion of 5 mL lipiodol created an area wider than 5 cm depending on the anatomy of the infused lung (Figure 1).
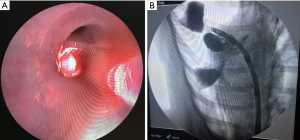
After weekly chest radiography follow-up for a month, we found that small amounts of lipiodol still remained in the lung tissue. No severe pneumonia or progression of lung infiltration was noted in the chest radiograph images (Figure 2).
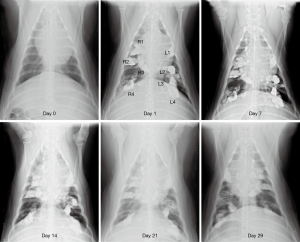
Autopsy revealed that there was still lipiodol retained in the alveolar space, with lymphocyte accumulation, and interstitial edema in the treated area. Although the lung inflammation was very mild compared with that in the untreated area, the severity of inflammation correlated with the light dose illumination (Figure 3). We failed to identify the treated area because of the difficulty in defining the exact margin of inflammation on gross inspection after formalin fixation and identifying the fiber-inserted bronchus (center of the illumination) after 1 month of therapy. However, this study proved that there was no severe lung necrosis noted after lipiodol infusion and even received up to 800 J (800 mW 1,000 s) light dose PDT treatment.
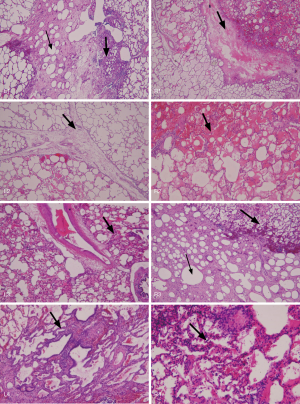
Verify the feasibility and safety of the MTD of light
Triplicate experiments were performed using a frontal light distributor optical fiber with 800 J (800 mW 1,000 s) irradiation. The first and second repeated tests were successfully completed with 800 J (800 mW 1,000 s) illumination energy. However, when 800 mW illumination was provided for the third time, the output end of the frontal light distributor optical fiber overheated, and lipiodol was vaporized causing internal combustion of the bronchi. Severe thermal damage was found in the bronchi and lung tissue parts being tested, and the pig was sacrificed the next day. The results showed that if there was no overheating of the fiber, the illumination energy of 800 mW 1,000 s will not immediately cause serious damage to the normal lung tissue. However, continuous long-term output of 630 nm red light of 800 mW energy might have risks of excessive temperature at the endpoint of the optical fiber because of contact with the tissue or poor heat dissipation in vivo (Figure 4).
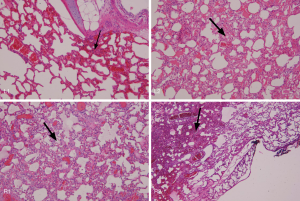
Ex vivo lung test
The ex vivo lung experiment showed that the illumination energy of 400 mW/cm 500 s with 5 cm cylindrical optical fiber (200 J/cm, totally 1,000 J) did not lead to overheating of the fiber and did not cause vaporization of lipiodol. Similar results were obtained in eight different parts of the two lungs, confirming that the illumination energy of 400 mW/cm 500 s with a 5-cm cylindrical optical fiber (200 J/cm, totally 1,000 J) would not lead to thermal damage to the lung tissue infused with lipiodol; 1,000 J energy illumination was still safe (Figure 5).
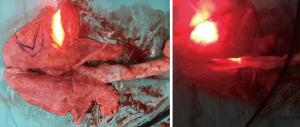
Discussion
PDT can be used to treat many kinds of cancers; however, because of limitations in the depth of light penetration, it can only be used in a few tumors on the surface of the skin or cavity. Friedberg et al. introduced a novel PDT model in 2003; he found that by instilling a solution with high RI (mineral oil) into a specific organ, light can be transmitted through the tubular structures infused with the solution, and the treatment depth can be enhanced by expanding the range of illumination (10). This model has the potential to be applied to tumors that were previously thought to be untreatable with PDT because of the inaccessibility of light.
PDT is not a new medical technology. Some photosensitive drugs such as porfimer sodium and talaporfin, have been investigated for the treatment of peripheral lung treatments in clinical trials (6-8). Lipiodol, which is a high-RI contrast medium, is also an FDA-approved drug, although it is not recommended for bronchoscope infusion in the lung (18). However, lipiodol has a 50-year history of use in lung infusion for bronchography, and its safety is ensured when used in small amounts for lung infusion. Many navigation devices for bronchoscopy imaging have been approved for clinical use. However, this particular illumination model is only a new combination of methods that are currently in clinical use.
We found that at 37 °C, lipiodol has an RI of 1.49, higher than that of mineral oil (1.46) and was used by Friedberg et al. in their study. Physically, lipiodol is also an oil with a high RI and should be a good replacement for mineral oil in this PDT light delivery model.
In this study, we developed a pig model to investigate whether lipiodol can be a suitable replacement for mineral oil in this novel therapeutic technique for peripheral lung tumors, and ultimately treated them with PDT with bronchoscope guidance under the surveillance of fluoroscopy. Using the pig lung model, we successfully demonstrated that bronchoscopy can be used to place the guide sheath in the far end of the lung and that lipiodol can be instilled into the lung through the guide sheath. Lipiodol is used as a contrast medium. X-ray fluoroscopy allowed monitoring of the instillation of lipiodol and ensured its infusion to the correct position. The viscosity of lipiodol allowed it to remain in the infused part for a certain time period without spillage, and this was helpful for the follow-up PDT therapy. Five mL of lipiodol can create an area of approximately 5 cm in diameter using this technique, which should be adequate for covering a small tumor <3 cm, which we intend to treat in the future. Lipiodol infusion was safe in this model. A pig of about 50 kg tolerated a total of about 40 mL of lipiodol infusion and received different doses of PDT without major complications such as acute severe and fatal pneumonia or lung injury and survived for at least 1 month.
It is crucial to determine the proper light dose to be delivered to the target tumor. Previous clinical trials demonstrated the feasibility of using a cylindrical fiber with a light output of 200 J/cm (400 mW/cm 500 s) in porfimer sodium (4,6,7). Instead of examining this light delivery method in a pig lung cancer model, which is difficulty to establish, we assessed the MTD of light that the normal lung could receive. We demonstrated that the normal pig lung can tolerate up to 800 J of light without severe lung injury, under the 20 mg/kg 5-ALA photosensitizer. We did not pinpoint the proper light dose, but our study has revealed possible light illumination doses that can be used in future clinical trials.
Complications due to overheating may have occurred due to the high energy output produced in a short period of time in the frontal light diffuser, or due to the lung tissue coming in contact with the fiber tip; however, this could be avoided by using a cylindrical light diffuser fiber with a low-energy setting and prolonged illumination time. In our study, a 5 cm cylindrical laser fiber with 200 J/cm energy output was safe without overheating, and this light dose is the setting currently used for porfimer sodium PDT.
This was a pilot study to test the technical feasibility of using lipiodol in the PDT light delivery model proposed by Dr. Friedberg and the safety of lipiodol infusion to the lung. We assessed the tolerable light dose in a 5-ALA PDT model. More studies are required to establish the light dosimetry and distribution model as well as the suitable drug-light dose relationship for achieving the best treatment responses without adverse effects. The distribution model of light flux in the lung tissue is very important for the treatment plan. Presently, there are no pre-clinical and clinical trials to discuss the model of light flux distribution in the lung tissue for PDT in the lung. In the next experiment, we plan to perfuse lipiodol in fresh pig lungs that have just been sacrificed, test the luminous flux in sequence at a certain distance from the light source, and find the model relationship of the luminous flux distribution of light in this perfused lipiodol lung tissue just like the radiation energy distribution model in tissue in radiation therapy.
In fact, this novel PDT model is not completely new at all. Photofrin has been used for lung cancer PDT for decades. Lipiodol has also been used clinically for nearly 100 years. This model is just a new combination. Our experiments have confirmed perfusion technology is feasible. Lipiodol will stay in the target lung tissue. We can use the navigational bronchoscope system to place the laser fiber near the tumor or inside the tumor for illumination. We have confirmed that when using a 5-cm cylindrical fiber to give 400 mW 500 s (200 J/cm), there will be no adverse reactions to overheating with lipiodol. It might go directly to the clinical trial using Photofrin as the photosensitizer and add the lipiodol as the light diffusor. The application for Institution Review Board (IRB) is in process now.
If the PDT model can be proven safe and if we can also find a safe and effective photosensitive drug, an illumination dose, and amount of lipiodol to be infused, lipiodol can be infused to the proximal bronchi to fill the lung segment or the whole lobe where the tumor is located. The most attractive feature of PDT is the selective destruction of cancer cells and the preservation of normal organs and tissues. In this way, all micro-metastatic lesions in the lung segment or lobe and the lesions in the vascular and lymphatic system can be killed while preserving normal lung tissues and function. This highlights the feasibility of photodynamic segmentectomy or photodynamic lobectomy for lung cancers.
This PDT model with lipiodol can be used not only in the treatment of lung cancer, but also potentially in the treatment of hepatocellular carcinoma. Currently, lipiodol can be infused into the hepatic artery via angiography for transcatheter arterial embolization (TAE). There could also be cases for which angiography could be used to place the optical fibers in liver tumors for illumination. Transmission effect is formed by light in the liver, infused with lipiodol, which produces an illumination range for PDT of hepatocellular carcinoma that enhances the result of TAE.
In summary, these experiments have proven that lipiodol can be safely infused into the lung and bronchial tissues as a diffuser for the PDT model studied by Friedberg et al. Lipiodol has a high RI and can cause an optic fiber transmission effect in the bronchi, improving the efficiency and range of illumination in the lung and bronchial tissue. Navigational bronchoscopy can be used to place the sheath at the target tumor for lipiodol infusion. A laser fiber can be placed in the lipiodol infusion area and PDT can then be performed. However, a longer illumination time with higher energy may cause the temperature of the optical fiber to rise and lead to the vaporization and burning of lipiodol. Further research is needed to develop safer illumination equipment and to identify safer and more effective energy doses. The distribution model of light in lipiodol-filled lung tissue, as well as the effective dose of photosensitive drugs and illumination energy, also need to be investigated.
Acknowledgments
We thank Dr. Joseph S. Friedberg and Dr. Daniel Sterman for their novel photodynamic model; Dr. Yao-Hon Wang and Prof. Yih-Chih Hsu for their laboratory support; Tracy Lo, Mina Chen, and Melissa Culligan for editing support.
Funding: We thank Taoyuan General Hospital, Ministry of Health and Welfare, Taiwan for their support (grant number PTH10704/PTH10720/PTH108007).
Footnote
Data Sharing Statement: Available at http://dx.doi.org/10.21037/JTD-19-3887
Conflicts of Interest: All authors have completed the ICMJE uniform disclosure form (available at http://dx.doi.org/10.21037/jtd-19-3887). The authors have no conflicts of interest to declare.
Ethical Statement: The authors are accountable for all aspects of the work in ensuring that questions related to the accuracy or integrity of any part of the work are appropriately investigated and resolved. The trial was conducted in accordance with the Harmonized Tripartite Guideline for Good Clinical Practice from the International Conference on Harmonization. All applicable international, national, and/or institutional guidelines for the care and use of animals were followed. The animal use protocol was reviewed and approved by the Institution of Animal Care and Use Committee (IACUC) of Pigmodel Animal Technology Co., Ltd. (Approval No. PIG-107007).
Open Access Statement: This is an Open Access article distributed in accordance with the Creative Commons Attribution-NonCommercial-NoDerivs 4.0 International License (CC BY-NC-ND 4.0), which permits the non-commercial replication and distribution of the article with the strict proviso that no changes or edits are made and the original work is properly cited (including links to both the formal publication through the relevant DOI and the license). See: https://creativecommons.org/licenses/by-nc-nd/4.0/.
References
- National Lung Screening Trial Research Team, Aberle DR, Adams AM, et al. Reduced lung-cancer mortality with low-dose computed tomographic screening. N Engl J Med 2011;365:395-409. [Crossref] [PubMed]
- Harris K, Puchalski J, Sterman D. Recent Advances in Bronchoscopic Treatment of Peripheral Lung Cancers. Chest 2017;151:674-85. [Crossref] [PubMed]
- Dolmans DE, Fukumura D, Jain RK. Photodynamic therapy for cancer. Nat Rev Cancer 2003;3:380-7. [Crossref] [PubMed]
- Okunaka T, Kato H, Tsutsui H, et al. Photodynamic therapy for peripheral lung cancer. Lung Cancer 2004;43:77-82. [Crossref] [PubMed]
- Usuda J, Inoue T, Ibi T, et al. MA05. 11 Photodynamic therapy for peripheral lung cancer using composite-type optical fiberscope of 1.0 mm in diameter. J Thorac Oncol 2017;12:S36.
- Chen KC, Lee JM. Photodynamic therapeutic ablation for peripheral pulmonary malignancy via electromagnetic navigation bronchoscopy localization in a hybrid operating room (OR): a pioneering study. J Thorac Dis 2018;10:S725-30. [Crossref] [PubMed]
- Feasibility study of using navigational bronchoscopy to perform PDT-Photofrin® in unresectable peripheral lung cancer. ClinicalTrials.gov Identifier: NCT02916745.
- Musani AI, Veir JK, Huang Z, et al. Photodynamic therapy via navigational bronchoscopy for peripheral lung cancer in dogs. Lasers Surg Med 2018;50:483-90. [Crossref] [PubMed]
- Friedberg JS, Skema C, Burdick J, et al. A novel technique for light delivery through branched or bent anatomic structures. J Thorac Cardiovasc Surg 2003;126:1963-7. [Crossref] [PubMed]
- Devonshire A, Malhotra S, Nevin M. A case of lipoid pneumonia from mineral oil aspiration with delayed-onset ARDS. Chest 2015;148:632A. [Crossref]
- Leroux BT, Duncan JG. Bronchography with hytrast. Thorax 1964;19:37-43. [Crossref] [PubMed]
- Ballon DH, Ballon HC. The effect of injection of lipiodol and the rate of its disappearance, in normal and diseased lungs. Can Med Assoc J 1927;17:410-6. [PubMed]
- Kwon WJ, Kim HJ, Jeong YJ, et al. Direct lipiodol injection used for a radio-opaque lung marker: stability and histopathologic effects. Exp Lung Res 2011;37:310-7. [Crossref] [PubMed]
- Rose M, Siva S, Ball D, et al. Bronchoscopic delivery of lipiodol as a fiducial marker in lung tumors before radiotherapy. J Thorac Oncol 2014;9:1579-83. [Crossref] [PubMed]
- Yamagami T, Yoshimatsu R, Miura H, et al. Pneumonia occurring after injection of Lipiodol to localize pulmonary nodules before fluoroscopy-aided thoracoscopic resection. Acta Radiol Short Rep 2014;3:2047981613499754. [Crossref] [PubMed]
- Yagi R, Kawabata S, Ikeda N, et al. Intraoperative 5-aminolevulinic acid-induced photodynamic diagnosis of metastatic brain tumors with histopathological analysis. World J Surg Oncol 2017;15:179. [Crossref] [PubMed]
- Mackenzie GD, Dunn JM, Selvasekar CR, et al. Optimal conditions for successful ablation of high-grade dysplasia in Barrett's oesophagus using aminolaevulinic acid photodynamic therapy. Lasers Med Sci 2009;24:729-34. [Crossref] [PubMed]
- Available online: https://www.accessdata.fda.gov/drugsatfda_docs/label/2014/009190s021lbl.pdf