New applications of a portable isolation hood for use in several settings and as a clean hood
Introduction
In every winter in the temperate zone as well as in a pandemic situation, hospitals are often overflowed with influenza patients and beds in wards are occupied by these patients. Such situations are inevitably associated with a risk of nosocomial airborne infections (1-4). It is the same with the epidemic of exotic contagious respiratory illness like SARS, MERS, and COVID-19 (5-7).
Such a risk increases in a place like a ward room with multiple numbers of beds or a renal dialysis facility, where many patients are cared for simultaneously in a narrow, closed space, since the virus is usually spread through airborne mists from sneezing, coughing and even from breathing of the patient (8-12).
Physical interventions such as isolation and/or cohort nursing can help to reduce nosocomial transmission (13), although few hospitals have sufficient individual patient isolation rooms available—even fewer with negative pressure ventilation capability (14). Personal protective equipment (PPE) such as face masks (15,16), and increasing ventilation rates (i.e., air-exchanges per hour, ACH) (17-19), with or without stand-alone room air filtration units or air-cleaners (electronic fan-HEPA-filter units, FFUs) can also be employed (20).
However, these methodologies have their own disadvantages. Long-time mask wearing leads to poor quality of life (QoL) for both influenza and non-influenza patients; frequent ventilation of the room air in the winter season is not practical in the temperate area; high-performance is required for air cleaners to filter enough amount of room air compared with the total room air volume, which leads to frequent ventilation to be practically competent for prevention of airborne transmissions.
To resolve those issues, several products that put a patient in a closed space were manufactured for efficient isolation: e.g., a large and heavy, negative-pressure chamber with an FFU, which looked almost the same as a small room, or products with a simple, large FFU panel placed at the head side of a patient's bed. However, they have disadvantages in portability and more importantly, did not provide scientific validity for controlling airborne viruses that are supported by experimental data.
Thus, we proposed a personal, easily portable hood system that included a small fan-filter air-extraction system that covers the top half a patient sitting or lying in bed (21).
It provides good QoL for the patient with low cost, is compact and light enough for high portability for practical use in clinical settings, and above all, is supported with scientific data on the containment or removal of airborne pathogens based on experimental data.
After completing development of the hood with the original idea, we tried other new applications of the hood to cope with the following issues: whether the hood could be applicable for (I) use in ICU where a nurse to perform tracheal suctioning that generates much aerosol from the patient, a risky procedure among clinical practice (22); (II) use in the special outpatient clinic in a hospital for COVID-19 suspected cases without good ventilation, to prevent dissemination of airborne pathogen in its crowded waiting room and (III) isolation of a child in a nursery who suddenly showed influenza-like symptoms and waiting for pick-up by the guardian.
In addition, we explored the possibility that the hood could be easily changed to the positive pressure hood by using its basic structure to convert it to a clean booth by changing the direction of the airflow of the FFU. This is done by reversing its setting, which brings clean air to the inside through the FFU.
Methods
The hood
The details of the structure of the hood named Barrihood, were described in the previous paper (21) In short, it had dimensions height 172 cm, width 97 cm, length 38 cm, constructed out of lightweight pipes, transparent plastic curtains, and an FFU which was easily assembled on the pipe frame by a single person. It is operated on AC power and is warranted to run for at least 1,000 cumulative hours.
Physical analyses
The air speed was measured using an air flow meter, model 6141, KANOMAX Japan Inc. (Osaka, Japan). The air flow rate was obtained from the formula, “air speed x opening area”. The positive pressure inside the hood was measured with a fine differential pressure gauge, DPC-500N12, Okano Works. Ltd. (Osaka, Japan).
Airborne particle experiments for positive pressure hood
Saline (1 wt%) was atomized for 2 s with a nebulizer (NE-C28, Omron Healthcare Co., Ltd., Kyoto, Japan) set outside the hood in 25 m3 chamber. Thereafter, particle concentration in the air was measured for five particle size-ranges from 0.3 to 5.0 µm with a Laser particle counter (KR-12A, Rion, Tokyo) both inside and outside the hood.
Experiments using airborne virus
Details of the chamber, virus and airborne experiments using atomized influenza virus fluid were described in the previous report (21). In short, the double enclosure system of a sealed chamber of 14.4 m3 equipped with FFUs to remove the airborne virus in a short time was placed in a secondary negative-pressure clean room with a larger space-size. The temperature and relative humidity in the chamber were controlled at 21–22 °C and about 30%, respectively.
The difference in viral concentration between the inside and the outside the hood was measured using influenza virus A/Aichi/2/68 (H3N2) grown in the allantoic cavity of fertilized chicken eggs as described previously (21). Collection of the airborne virus was done using an air sampler and a gelatin membrane filter (MD8 AirScan Sartorius AG, Göttingen, Germany) followed by dissolving the membrane in 10 mL of culture medium. The medium was subjected to a conventional plaque assay using Madin-Darby canine kidney cells (23).
Ethics approval
The experiments were approved by the Ethics Committee of Sendai National Hospital (IRB NO.: 17-2). The study conformed to the provisions of the Declaration of Helsinki (as revised in 2013), and conducted in a strict biosafety facility, with safety procedures of frequent monitoring of the airborne particle counts of the working environment followed by air cleaning using FFUs.
Results
New applications of the original, negative pressure hood
The hood had been used in the general ward for isolation of seasonal influenza patients (21), and several requests came from the ICU to be used for an influenza patient because several beds were occupied by patients with illnesses other than influenza. The main concern then was whether or not the setting of the mechanical ventilator and practice of tracheal suctioning was possible while setting the hood to the bed without difficulty. It was resolved by using the revised hood with side curtains having two-way zippers, which can create an opening. The hood was set on the lateral side of the bed over the head, and tubes from the ventilator were extended to be able to reach the patient through the window, and the nurse can do suctioning also through the window (Figure 1A).
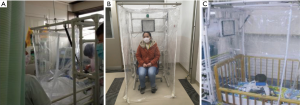
Second, we tried to apply our hood to prevent dissemination of airborne pathogen in a crowded waiting room. Due to the shortage of isolation facilities during the SARS epidemic in 2003, fast-track ventilation strategies for dealing with patient surges was tried in affected countries (24). The same situation was expected in the special outpatient clinic in our hospital for COVID-19 suspected cases.
We used the negative pressure hood set without bed to isolate a suspected case sitting on a chair inside the hood with curtains that reached the floor (Figure 1B).
Physical and biophysical experiments for validation of the hood to be used as a waiting hood
Containment of the virus inside the hood was experimentally confirmed in two ways: physically, the air pressures inside and outside of the waiting hood were measured and air pressure inside was calculated as −0.37 Pa. Experiments using active influenza virus were the same as previously done with the original negative pressure Barrihood (21).
Aerosol containment experiments for the waiting hood use was performed as follows: the virus fluid of about 108-9 plaque forming unit was atomized in the hood in the presence of an active FFU of rated airflow rate 0.42 m3/min. At 0 and 20 min after switching on the FFU, the mists inside and outside the hood were sampled with air for 3 min, followed by titration of the active virus. The virus atomized in the hood did not leak outside the hood using the rated flow of the negative pressure-use (0.42 m3/min) in the FFU (Table 1).
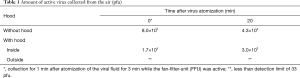
Full table
Third, two nursery schools consulted us whether the hood could be applied to children for their temporary isolation when any of them suddenly showed influenza-like symptom(s) while attending the school and waiting to be picked-up by their guardian. The hood has enough space for a small child to stay inside. A concern was whether children could sit there without problem. We just tried and, consequently, a child in a nursery could stay for at least one hour playing by himself with toys, without any trouble. (Figure 1C).
Physical and biophysical experiments for validation of the hood to be used as a positive-pressure hood
The hood was converted to be used as a positive-pressure hood, as well. It is for use as a clean hood to protect an immunologically incompetent person like a patient after chemotherapy or an individual with respiratory susceptibility to environmental hazardous airborne particles like PM2.5 or allergens like pollen. Theoretically, the hood is easily converted between negative and positive pressure uses because the FFU airflow is reversible. By changing the direction of the setting, the positive pressure use brings clean air to the inside through the FFU, from the contaminated outside air. The conversion can be done by a single non-professional person in 10 minutes.
The inside pressure, airflow velocity at the entrance, and airflow rate of the positive pressure use was 0.26 Pa, 0.1 m/s, 0.39 m3/min, respectively.
Analyses using airborne fine particles generated from saline and viral fluid
The laser particle counters were set outside and inside and experiments using FFU with various airflow rates to monitor the particle counts were conducted after atomization of the saline. As a result, the particle count of size-level more than 0.3 µm decreased drastically to 4–21/cf3 (140–700/m3) with airflow rate at least 0.19 m3/min within 15 min after switching on the FFU (Figure 2), which physiologically qualified for the standards of a bio-clean room class 100 of NHB-5340-2, NASA and the class 4 for particles sized over 0.5 µm of ISO.
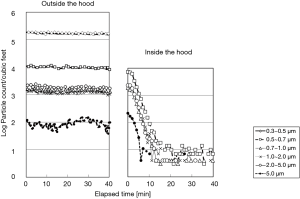
The experiment using atomized saline or active virus fluid with the hood running with a flowrate of 0.39 m3/min showed that concentrations of saline aerosol particles of every size were reduced to the minimum levels (Figure 3) and viral concentration was also confirmed to be reduced to less than the detection-limit level (Figure 4).
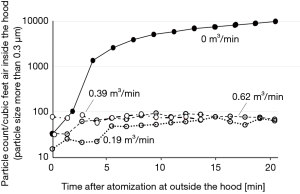
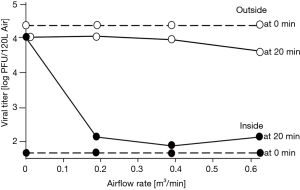
Thus, we suggest that the positive-pressure hood could be applied for individuals to be protected from contaminated air as a portable semi-clean space.
Discussion
We suggested applications of the Barrihood other than as a simple isolation hood that covers the upper body of a patient on a bed that we reported previously. Firstly, it will be useful for isolation of a patient with COVID-19, as well as those with respiratory distress who requires respirator in a bed in ICU without negative pressure function, as has been introduced in our case with influenza. Secondly, it will be also useful for temporary isolation of a COVID-19 suspected out-patient at the waiting area of a special out-patient clinic, with only its curtains rolled-down to the floor and a chair inside. Thirdly, for isolation of a younger child without becoming uneasy in it; the hood size is adequate for children, not so broad but not so narrow and the curtain is transparent. The child feels no fear inside the space, while teachers are working near. These varying applications might be possible because the hood is lightweight, portable, and quick and easy to set-up.
Lastly, another application of the hood is the positive-pressure use. This way of usage would be applicable to many settings: for immunocompromised patients with leukemia or other cancers who return from a heavy-duty clean room after completing chemotherapy, or after receiving immunosuppressing agents for organ transplantation to avoid airborne microbes that cause opportunistic infections. For such high-risk patients in general, the heavy-duty clean room is used even after completing therapy, but such facilities are not available for many patients at once in most settings. The hood would be useful as a second-line clean environment after patients successfully passed the dangerous phase of their illness. In addition, the hood can be applied for individuals susceptible to PM2.5 particles or airborne allergens. It will be a sanctuary for them to avoid pollens or house dusts. They can stay safe inside the hood with better QoL without wearing a stifling, high performance face mask.
Conclusions
The Barrihood is applicable not only in the general hospital ward but also in ICU and a special out-patient clinic for isolation of respiratory contagious disease, as well as in a nursery or daycare facility settings. It can be used as a clean hood for an individual to be protected from microbes or other airborne particles. All of these applications are possible because of its mobile feature. The utility of the hood is supported by laboratory evidence and it will be strengthened by more clinical experience.
Acknowledgments
The authors greatly appreciate Dr. Julian W Tang and Dr. Isolde Dapat for English language review.
Funding: Annual research subsidy from Sendai Medical Center, Sendai, Japan.
Footnote
Data Sharing Statement: Available at http://dx.doi.org/10.21037/jtd-20-1211
Conflicts of Interest: All authors have completed the ICMJE uniform disclosure form (available at http://dx.doi.org/10.21037/jtd-20-1211). SS is a retired ex-employee of Takasago Thermal Engineering Co. LTD. Japan worked as a technical expert. SS was retired three years ago and he has no right of the intellectual property on the Barrihood and does not get any reward from the company on it. The other authors have no conflicts of interest to declare.
Ethical Statement: The authors are accountable for all aspects of the work in ensuring that questions related to the accuracy or integrity of any part of the work are appropriately investigated and resolved. The experiments were approved by the Ethics Committee of Sendai National Hospital (IRB NO.: 17-2). The study conformed to the provisions of the Declaration of Helsinki (as revised in 2013). Informed consent was waived due to the nature of the study.
Open Access Statement: This is an Open Access article distributed in accordance with the Creative Commons Attribution-NonCommercial-NoDerivs 4.0 International License (CC BY-NC-ND 4.0), which permits the non-commercial replication and distribution of the article with the strict proviso that no changes or edits are made and the original work is properly cited (including links to both the formal publication through the relevant DOI and the license). See: https://creativecommons.org/licenses/by-nc-nd/4.0/.
References
- Wong BC, Lee N, Li Y, et al. Possible role of aerosol transmission in a hospital outbreak of influenza. Clin Infect Dis 2010;51:1176-83. [Crossref] [PubMed]
- Lindsley WG, Blachere FM, Davis KA, et al. Distribution of airborne influenza virus and respiratory syncytial virus in an urgent care medical clinic. Clin Infect Dis 2010;50:693-8. [PubMed]
- Blachere FM, Lindsley WG, Pearce TA, et al. Measurement of airborne influenza virus in a hospital emergency department. Clin Infect Dis 2009;48:438-40. [Crossref] [PubMed]
- Leung NH, Zhou J, Chu DK, et al. Quantification of influenza virus RNA in aerosols in patient rooms. PLoS One 2016;5;11:e0148669.
- Cooper BS, Fang LQ, Zhou JP, et al. Transmission of SARS in three Chinese hospitals. Trop Med Int Health 2009;14 Suppl 1:71-8. [Crossref] [PubMed]
- Kim KH, Tandi TE, Choi JW, et al. Middle East respiratory syndrome coronavirus (MERS-CoV) outbreak in South Korea, 2015: epidemiology, characteristics and public health implications. J Hosp Infect 2017;95:207-13. [Crossref] [PubMed]
- Wang D, Hu B, Hu C, et al. Clinical Characteristics of 138 Hospitalized Patients With 2019 Novel Coronavirus-Infected Pneumonia in Wuhan, China. JAMA 2020;323:1061-9. [Crossref] [PubMed]
- Tellier R. Aerosol transmission of influenza A virus: a review of new studies. J R Soc Interface 2009;6 Suppl 6:S783-90. [Crossref] [PubMed]
- Lau LL, Cowling BJ, Fang VJ, et al. Viral shedding and clinical illness in naturally acquired influenza virus infections. J Infect Dis 2010;201:1509-16. [Crossref] [PubMed]
- Hatagishi E, Okamoto M, Ohmiya S, et al. Establishment and clinical applications of a portable system for capturing influenza viruses released through coughing. PLoS One 2014;9:e103560. [Crossref] [PubMed]
- Lindsley WG, Blachere FM, Beezhold DH, et al. Viable influenza A virus in airborne particles expelled during coughs versus exhalations. Influenza Other Respir Viruses 2016;10:404-13. [Crossref] [PubMed]
- Yan J, Grantham M, Pantelic JP, et al. Infectious virus in exhaled breath of symptomatic seasonal influenza cases from a college community. Proc Natl Acad Sci U S A 2018;115:1081-6. [Crossref] [PubMed]
- Jochimsen EM, Fish L, Manning K, et al. Control of vancomycin-resistant enterococci at a community hospital: efficacy of patient and staff cohorting. Infect Control Hosp Epidemiol 1999;20:106-9. [Crossref] [PubMed]
- Miller SL, Clements N, Elliott SA, et al. Implementing a negative-pressure isolation ward for a surge in airborne infectious patients. Am J Infect Control 2017;45:652-9. [PubMed]
- Noti JD, Lindsley WG, Blachere FM, et al. Detection of infectious influenza virus in cough aerosols generated in a simulated patient examination room. Clin Infect Dis 2012;54:1569-77. [Crossref] [PubMed]
- Milton DK, Fabian MP, Cowling BJ, et al. Influenza virus aerosols in human exhaled breath: particle size, culturability, and effect of surgical masks. PLoS Pathog 2013;9:e1003205. [Crossref] [PubMed]
- Escombe AR, Oeser CC, Gilman RH, et al. Natural ventilation for the prevention of airborne contagion. PLoS Med 2007;4:e68. [Crossref] [PubMed]
- Li Y, Leung GM, Tang JW, et al. Role of ventilation in airborne transmission of infectious agents in the built environment - a multidisciplinary systematic review. Indoor Air 2007;17:2-18. [Crossref] [PubMed]
- Beggs CB, Kerr KG, Noakes CJ, et al. The ventilation of multiple-bed hospital wards: review and analysis. Am J Infect Control 2008;36:250-9. [Crossref] [PubMed]
- Ward M, Siegel JA, Corsi RL. The effectiveness of stand alone air cleaners for shelter-in-place. Indoor Air 2005;15:127-34. [Crossref] [PubMed]
- Nishimura H, Sakata S. Development of a lightweight, ‘on-bed’, portable isolation hood to limit the spread of aerosolized influenza and other pathogen. J Thorac Dis 2020;12:3682-7.
- Tablan OC, Anderson LJ, Besser R, et al. Guidelines for preventing health-care-associated pneumonia, 2003: Recommendations of CDC and the Healthcare Infection Control Practices Advisory Committee. MMWR Recomm Rep 2004;53:1-36. [PubMed]
- Matrosovich M, Matrosovich T, Garten W, et al. New low-viscosity overlay medium for viral plaque assays. Virol J 2006;3:63. [Crossref] [PubMed]
- Yuen PL, Yam R, Yung R, et al. Fast-track ventilation strategy to cater for pandemic patient isolation surges. J Hosp Infect 2012;81:246-50. [Crossref] [PubMed]