MicroRNAs in mesothelioma: from tumour suppressors and biomarkers to therapeutic targets
Introduction
Malignant pleural mesothelioma (MPM) is an asbestos-induced cancer with poor prognosis (1). Median survival following diagnosis is around 12 months, with only modest response rates and limited survival advantage obtained with currently used therapies (2,3). MPM is most frequently diagnosed in patients over 70 years of age, which poses difficulties for accurate identification of the disease as definitive diagnosis requires a biopsy (4). In some cases MPM can also be difficult to differentially diagnose from cases of adenocarcinoma in the pleura (4). The molecular markers identified to date are not sufficiently accurate to be used routinely in the diagnosis of MPM, and single markers also fail to accurately predict prognosis (5,6). To date the identification of new molecular targets for therapeutic interventions in MPM has also proved elusive. Although MPM cells frequently show overexpression of epidermal growth factor receptor (EGFR) and other targetable proteins, clinical trials of molecular agents targeting these receptors have yielded disappointing results (7). In comparison with non-small cell lung cancer (NSCLC), driver mutations appear to be rare in MPM, which is generally considered to be a cancer primarily caused by loss of tumour suppressor genes rather than resulting from gain in oncogene mutations. Thus, MPM remains a difficult tumour to manage in the clinic, and new biomarkers and therapeutic targets are urgently needed to improve the outlook for patients.
Recent research has revealed that microRNAs play important roles in the biology of MPM, and have the potential to serve as both biomarkers and therapeutic targets. This review will focus on those studies that have reported biologically relevant changes in the microRNA expression found in MPM cells and tumours, and the potential application of these findings in the clinic.
MicroRNAs: small RNAs with a big role in cancer
MicroRNAs are short non-coding RNAs of approximately 18-22 nucleotides in length (8), which function as post-transcriptional regulators of gene expression. Primary microRNAs transcripts (pri-miRNAs) are transcribed either from dedicated upstream promoters, or co-transcribed within introns of protein-coding genes. Following a series of post-transcriptional processing steps, the precursor (pre-miRNA) is exported to the cytoplasm. Final processing yields the mature microRNA duplex, from which one strand is incorporated into the RNA-induced silencing complex (RISC). Within the RISC, microRNAs specifically target sites in the 3’UTR of messenger RNA (mRNA) transcripts, typically via imperfect base pairing, leading to repression of translation or destabilization of the mRNA and ultimately repression of protein levels (8,9). MicroRNAs are estimated to regulate ~30% of protein-coding genes (8) impacting most if not all cellular pathways. Due to the imperfect nature of microRNA target binding a single microRNA can target many mRNAs, and each mRNA can be under the post-transcriptional control of multiple microRNAs (10). The important role of microRNAs in normal biological processes is now clear, and disrupting the highly complex regulatory network of microRNAs within the cell can induce abnormal cell behaviour. As such, dysregulated microRNA expression is a common feature in human diseases, especially cancer (11).
The link between microRNA loss and cancer was first established well over a decade ago, when it was shown that the deletion (or down-regulation) of miR-15a and miR-16-1 encoded at the 13q14 locus occurred in a majority of B-cell chronic lymphocytic leukaemia cases. Soon after it was reported that the alterations in the expression of microRNA in various cancers could be critical to the understanding of cancer pathophysiology (12), and that many microRNAs are frequently encoded at fragile sites and common breakpoint regions in the genome, thus increasing the rates of microRNA deletion and mutation. It was subsequently shown that tumours frequently exhibit global downregulation of mature microRNA, due to a combination of chromosomal deletion, altered expression of the machinery controlling biogenesis, and epigenetic silencing (13). Furthermore, changes in microRNA expression within tumours have been shown to be more accurate classifiers of tumour origin than mRNA profiles (14,15). In MPM, as in other tumours, microRNA expression is dysregulated and there are now around 50 studies reporting on the expression of microRNAs in MPM. These include studies aiming to elucidate functional consequences of changes in microRNA expression, as well as those carried out with the goal of identifying microRNAs able to serve as biomarkers or therapeutic targets for MPM. This review will focus on microRNAs in MPM that fall into one or more of those categories.
MicroRNAs in MPM biology
Growing evidence points to significant alterations in microRNA expression in MPM. While the odd microRNA appears to be upregulated, there is a global suppression of microRNA expression, again in common with other cancer types. Early studies profiling the microRNA expression in MPM identified many changes, and although none were functionally validated, these findings suggested that such changes were likely to affect a variety of the phenotypes characteristic of the tumour, including reduced response to apoptotic signals, elevated rates of metabolism and proliferation, enhanced migration and invasion, and resistance to chemotherapy and radiation (16,17). Subsequent studies have revealed that many microRNAs have important functions in MPM, and those for which a change in expression in MPM tissue or cell lines has been shown to affect MPM biology are listed in Table 1 and are described in more detail below.
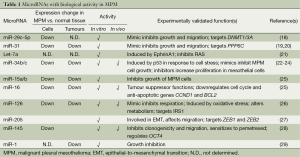
Full table
The first study to demonstrate activity of a microRNA in MPM showed that overexpression of miR-29c-5p—downregulated in MPM cell lines compared with normal mesothelial controls—was able to inhibit proliferation and invasion of MPM cells in vitro (18). DNMT1 and DNMT3A, targets of miR-29c-5p, were shown to be downregulated following overexpression, and this led to increased expression of methylation-silenced genes in these cells. Based on evidence from other cancers, the authors further postulated on a link between the miR-29 family and the NF-κB, apoptosis and PI3K pathways, all of which are altered in MPM, but as yet there is no data to support this hypothesis. In a similar study, miR-31 expression was shown to be reduced in MPM cell lines, in most cases via co-deletion with the CDKN2A gene at 9p21 (19). Re-expressing miR-31 led to reduced proliferation through cell cycle arrest, and inhibited migration and invasion. The introduction of miR-31 also reduced expression of PPP6C, a phosphatase linked with chemotherapy and radiation resistance, although a direct effect of altered miR-31 expression on chemo- and radio-resistance was not demonstrated. Moreover, this protein is associated with chromosomal instability, a common feature in MPM, and expression of PPP6C was highly elevated in tumour samples.
Another microRNA with inhibitory effects on proliferation and migration of MPM cells is let-7a. This and related microRNAs function to attenuate RAS signaling (30), and their tumour suppressor function was first demonstrated in lung cancer (31). While there have been no reports of downregulation of the let-7 family in MPM, in vitro evidence suggests that upregulation of let-7 family members is involved in the tumour suppressor activity of EphrinA1. Membrane-bound EphrinA1 signals via its receptor EphA2 to attenuate RAS activity and impair anchorage-independent growth of MPM cells, and this signaling was later shown to induce transcription of several let-7 family members in MPM (21). Moreover, a let-7-specific antisense reversed these effects, whereas a let-7 pre-miR could reproduce the growth inhibition and RAS downregulation in the absence of EphrinA1 (21).
More recently, altered expression of the well-known tumour suppressor microRNAs of the miR-34 and miR-15 families have been reported in MPM cell lines and tumours. The co-expressed miR-34b and miR-34c are silenced by methylation in the majority (85%) of MPM tumours, with a lesser degree of silencing observed for miR-34a (22). Stable transfection of MPM cells with a miR-34b/c construct decreased colony-forming ability, associated with an increase of cells in the G0-G1 phase of the cell cycle, and also inhibited motility, migration and invasion. Transient re-expression of miR-34b/c with an adenoviral vector led to increased apoptosis and inhibition of cell growth. In a subsequent study, the same group showed that stable transfection with miR-34b/c led to radiosensitisation of MPM cells, via reduced phosphorylation of histone H2AX, in part due to reduced expression of the miR-34b/c target CCND1 (23).
In addition to the effects of increasing levels of miR-34-b/c in MPM cells, the importance of this family has also been demonstrated by inhibiting the miR-34 family in mesothelial cell lines and primary cells (32). Transfecting mesothelial cells with inhibitors specific for miR-34a, -34b or -34c, led to increased proliferation, ability to form colonies, and invasive potential in these cells, associated with an increase in the protein expression of the miR-34 targets Bcl-2 and c-Met (32). Further evidence for a role of miR-34a was provided by an elegant study using genetically modified mice. In this study, mice with heterozygous inactivation of both Nf2 and Cdkn2a were generated and found to have an accelerated rate of malignant mesothelioma development upon exposure to asbestos via intraperitoneal injection (33). Cell lines derived from tumours harbouring these genetic lesions were also more metastatic than wild-type cell lines or those with single mutations, and metastatic potential was associated with increased presence of cancer stem cells. The metastatic lines were found to have increased expression and activation of c-Met, a therapeutic target in MPM. Activation was linked to an Mdm2-mediated inhibition of p53 leading to reduction of miR-34a levels. Conversely, re-expression of miR-34a via induction of p53 or with a miR-34a mimic reversed c-Met activation. This study thus implicates a role of miR-34a in both the carcinogenesis and aggressiveness of MPM.
The miR-15 family has also been shown to be downregulated in MPM (25). These microRNAs regulate cell cycle and anti-apoptotic genes (34), and loss or decreased expression was previously reported in prostate cancer (35) and lung adenocarcinoma (36). The members of this family for which expression could be detected—miR-15a, miR-15b, miR-16 and miR-195—were all found at significantly lower levels in MPM samples compared with those from normal pleura (25). This reduced expression was also found in cell lines. Mimics of miR-15a, miR-15b and miR-16 were able to inhibit MPM cell proliferation and induce cell cycle arrest and apoptosis, through regulation of gene targets including CCND1 and BCL-2. Restoring levels of miR-16 was also shown to sensitise cells to the antimetabolite chemotherapy agents gemcitabine and pemetrexed.
Another microRNA shown to be downregulated in MPM tumours is miR-1. Overexpression of this microRNA in MPM cell in vitro inhibited proliferation and this was at least in part due to induction of apoptosis (29). Interestingly, increasing miR-1 led to downregulation of survivin and Bcl-2, despite neither being a predicted target of this microRNA. However, no confirmatory experiments were performed, meaning that the true relationship between these observations remains unclear. Moreover, transfection with miR-1 resulted in increased expression of p16, p21 and p53, suggesting that all of the observed changes are an indirect result of the effect of miR-1 on other, as yet unidentified target genes.
In addition to alterations in proliferation, MPM cells also exhibit a propensity to migrate and locally invade surrounding tissue. Compared with the more differentiated epithelioid subtype, the less differentiated sarcomatoid subtype expresses increased levels of markers of epithelial-to-mesenchymal transition (EMT), which is thought to be associated with the increased invasive nature and poorer prognosis of sarcomatoid MPM (37). Recent evidence suggests that dysregulated microRNA expression in MPM is associated with changes in a number of EMT-related genes. Increased expression of the mesenchymal markers including vimentin, S100A4 and ZEB1 were higher in sarcomatoid tumours, and this was inversely correlated with miR-205 expression (27). Moreover, when the biphasic cell line MSTO was transfected with a miR-205 mimic, ZEB1 and ZEB2 mRNA levels were decreased and migration and invasion were significantly reduced.
Another microRNA influencing EMT in MPM is miR-145. Previously shown to be downregulated in a variety of solid tumours (38,39), miR-145 expression was shown to be downregulated in both MPM tumours and cell lines (28). Restoring levels of miR-145 with a mimic inhibited proliferation, induced senescence, and reduced migration and invasion and tumour growth in vivo. These effects were at least partly due to miR-145-induced downregulation of OCT4, a gene involved in EMT and chemoresistance, which in turn controls levels of ZEB1. There was an inverse correlation between miR-145 and OCT4 expression in tumour samples, and miR-145 also attenuated the pemetrexed-induced upregulation of OCT4, suggesting a role in drug resistance.
The metabolism of MPM cells is another biological feature influenced by changes in microRNA expression. The expression of miR-126 was shown to be differentially regulated by oxidative stress in MPM and mesothelial cells, with various stresses inducing downregulation of this microRNA (26). Stably transfected MPM cells exhibited impaired respiration and a glycolytic shift. This was related to a miR-126-induced downregulation of IRS1, which in turn suppressed activation of Akt signaling. Furthermore, miR-126 also reversed citrate-dependent HIF-1A activation and downstream VEGF-A expression.
In summary, the reduced expression of a variety of microRNAs in MPM seems to be associated with many of the characteristic biological changes observed in this cancer. The majority of these downregulated microRNAs appear to have tumour-suppressor activity when ectopically expressed in vitro, controlling proliferation of MPM cells via changes in cell cycle, apoptotic response and metabolism, as well as influencing the migratory/invasive capacity of the cells and their sensitivity to chemotherapeutic drugs.
MicroRNAs as biomarkers in MPM
The stability of microRNAs in a range of clinical samples has prompted investigations into their ability to serve as biomarkers for cancer (40). Many of the studies describing aberrant microRNA expression in MPM have investigated their potential to provide diagnostic or prognostic information, either in tumours themselves, or in blood samples. The studies are summarized in Table 2 and described in more detail below.
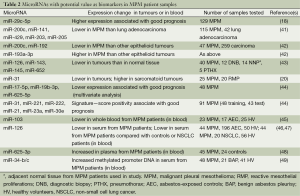
Full table
Tumour diagnostic markers
Two independent studies demonstrating that microRNAs could be a useful tool to aid diagnosis focused on the ability of microRNA expression to discriminate MPM from adenocarcinoma metastatic to the pleura (41,42). These reports followed the appearance of a diagnostic test marketed by Rosetta Genomics claiming to achieve highly accurate discrimination between these two diseases. In the first of these studies to be published, 15 MPM and ten adenocarcinoma biopsies were used in microarray-based discovery experiments which revealed significantly lower expression of five members of the miR-200 family together with miR-203 and miR-205 (41). These results were confirmed in a validation series of 100 MPM and 32 adenocarcinomas, with expression of these microRNAs shown to be from 6- to 42-fold lower in MPM samples. Using receiver operator characteristic (ROC) curve analysis, the miR-200 family microRNAs were able to distinguish the different samples with an accuracy of greater than 0.9. The authors further developed a random forest classifier which, when applied to the original discovery sample set led to a misclassification rate of only 10%.
The second paper appeared soon after, and was authored by Rosetta Genomics (42). In this study, microarrays were used to compare microRNA expression in seven MPM samples with 97 samples from a range of adenocarcinomas involving the lung or pleura. This identified 11 microRNAs present at significantly different levels in the two groups. Seven of these were lower in MPM and were members of the miR-200 and miR-192 families, while members of the miR-193 and miR-152 families were higher in MPM than other epithelial cancers. Based on RT-qPCR validation studies, a signature involving miR-192, miR-200c and miR-193a-3p was developed, and this was able to identify blinded samples from an independent set (14 MPM, 49 adenocarcinomas) with 95% accuracy (42). This assessment is the basis of the CLIA-compliant diagnostic test offered by Rosetta Genomics.
In a more recent attempt to identify microRNAs that are able to aid distinguish MPM from benign conditions, a panel of four microRNAs were shown to have diagnostic potential (43). This study was unique in that it compared matched diagnostic biopsies, MPM and non-neoplastic pleura (NNP) in the discovery set. Using a RT-qPCR-based approach, miR-126, -143, -145 and -652 were found to be significantly lower in MPM compared with NNP, and these data were validated in a larger set of tumours and normal pleural tissue samples. The diagnostic accuracy of each individual microRNA ranged from 0.76 to 0.93 in ROC analysis, and this was improved to a highly accurate figure of 0.96 by combining all 4 into a signature using logistic regression analysis. Additional small-scale studies have revealed a modestly significant reduction in miR-126 expression in ten MPM versus five normal pleura samples (46), and reduced miR-17-5p expression in 12 matched samples of MPM and normal tissue (50). While promising, independent validation of these data is needed to determine the true diagnostic value of these microRNA expression changes.
Tumour prognostic microRNAs
Accurately predicting the course of disease following diagnosis is also an unmet clinical need in the case of MPM, and a number of studies have investigated the ability of microRNA expression to aid in prognosis. An early report identified differentially expressed microRNAs in MPM cell lines and analysis in tumour samples suggested that high expression of miR-17-5p and miR-30c was associated with shorter survival in patients with sarcomatoid tumours, but this was based on a very small sample set (n=8) (16). In the first large scale study of MPM tumours, a training set of 37 and a validation set of 92 were used to identify miR-29c-5p as an independent prognostic factor (18). Expression of this microRNA was significantly higher in epithelioid tumours and was also associated with longer time to progression and greater overall survival. As described above, increasing expression of this microRNA had inhibitory effects on MPM cell proliferation and migration (18). The study identifying the four-microRNA diagnostic panel discussed in the previous section also assessed prognostic value of this signature using binary logistic regression analysis and log ranking (43). In Kaplan-Meier analysis, a low (less than median) logit(P) value was associated with poor prognosis. This study also found that higher than median levels of miR-193b expression were associated with a statistically significant shorter survival; both findings, however, require validation as the survival data was not complete in this series (43).
More recently, two groups of surgical specimens were used to analyse the association between survival and microRNA expression (44). Microarray analysis comparing expression in samples from long and short survivors following extrapleural pneumonectomy identified a number of microRNAs that were associated with differences in survival. RT-qPCR-based validation in a training set of 48 samples identified nine microRNAs with significant association with survival in univariate analysis, of which three—miR-21, miR-625-3p and miR-19b-3p—remained significant in multivariate analysis. In order to identify microRNAs able to predict good prognosis (survival ≥20 months), microRNA expression (alone and in combination) was assessed by binary logistic regression. This revealed a signature consisting of six microRNAs (miR-21-5p, -23a-3p, -30e-5p, -221-3p, -222-3p and -31-5p) that upon ROC analysis produced an AUC of 0.867, and which following Kaplan-Meier analysis showed a statistically significant difference of 22.9 months between score positive and negative groups. This signature was validated in samples from a group of patients who underwent pleurectomy/decortication and was associated with a survival difference of 8.9 months.
The latest addition to the literature surrounding tumour microRNA expression with prognostic value focused on miR-31 (20), frequently co-deleted with the CDKN2A locus in MPM cell lines (19). This study found a significantly lower expression of miR-31 in samples from MPM patients compared with those from patients with reactive mesothelial proliferations (20). In terms of survival, patients with normal or lower miR-31 expression than the average of the controls had longer survival (30.1 and 27.3 months, respectively), whereas those with high expression had the shortest survival (5.3 months). Interestingly, of the five cases with higher miR-31 levels, four were biphasic or sarcomatoid, and the single biphasic case with very low levels had longer survival than the epithelioid cases. Whether this observation will be confirmed in independent series remains to be seen, but it is intriguing that miR-31 targets LATS2 (51), a tumour suppressor frequently dysregulated in MPM (52) and part of the Hippo pathway also affected by Nf2 (Merlin) mutations and YAP1 upregulation (53).
Blood-based microRNA markers
It is generally accepted that a definitive diagnosis of MPM requires a biopsy to confirm invasive growth (6). As MPM affects mainly elderly patients, tumour biopsies are not always available, and much effort has focused on identifying blood-based diagnostic markers (54). Although several proteins present in the blood are found at elevated levels in MPM patients, none are sensitive or specific enough to aid in diagnosis or prognosis. In recent years it has become clear that microRNAs are present and readily measurable in blood and other body fluids, and that they have potential as biomarkers (55). Consequently, a handful of papers have proposed that microRNAs present in plasma, serum or whole blood may have value as biomarkers for MPM.
The first study identifying a circulating microRNA biomarker analysed whole blood from MPM patients and a mix of asbestos-exposed and unexposed healthy controls using microarray (45). This approach yielded a number of candidates, both up- and down-regulated, but only two reached statistical significance. Validation using RT-qPCR confirmed that lower levels of miR-103 were present in patients, and ROC analysis showed that these levels were better able to discriminate MPM from healthy controls (AUC =0.871) than patients from asbestos-exposed controls (AUC =0.757), although the numbers of patients (n=23) and controls (n=17) were relatively small. While the authors postulated on the significance of this change with regard to MPM cell biology, it is unclear how a microRNA detected in peripheral blood cells relates to expression in the tumour. Nevertheless, and regardless of biology and functional significance, decreased levels of miR-103 (or other microRNAs) in peripheral blood may indeed be useful biomarkers, but this requires independent validation.
In a study involving analysis of a much larger series (44 MPM patients, 196 asbestos-exposed and 50 healthy controls), data from a discovery series in tumours was used to select candidates for analysis. The results showed that miR-126 was present at significantly lower levels in serum samples from MPM patients versus either asbestos-exposed or healthy controls, with moderate power to discriminate between the groups (46). This finding reflected the results obtained in tissue samples, in which miR-126 was lower in tumour tissue than in adjacent normal pleura from the same patient. Furthermore, levels of miR-126 were correlated to those of VEGF and SMRP, with risk of disease increasing with higher SMRP and lower miR-126. In a follow up study, RT-qPCR analysis was used to assess levels of miR-126 in patients with MPM, NSCLC or healthy controls (47). The levels of miR-126 in this study were significantly lower in MPM patients than in either NSCLC patients or controls, and within the MPM group, lowest levels of miR-126 were associated with significantly shorter survival.
The third study to assess circulating microRNAs also began with a microarray-based comparison, using plasma from MPM patients and controls (48). Based on the array, only 3 of 90 microRNAs previously associated with MPM were present at altered levels in the patient samples. Of the candidate microRNAs found to be differentially present in the two groups and validated by RT-qPCR, only miR-625-3p remained statistically significant, with 3.8-fold higher levels in patients (15 MPM vs. 14 normal). In the analysis of a second set of serum samples (30 MPM vs. 10 asbestosis patients), miR-625-3p was again higher in MPM. ROC analysis in each set gave AUC values of around 0.8, suggesting that miR-625-3p levels have potential diagnostic value. Interestingly, miR-625-3p was also found to be higher in tumours (18 MPM vs. 7 non-malignant pleura), meaning the elevated levels in the circulation might originate in the tumours.
Together, these three studies provide tantalizing evidence that circulating microRNAs may have value as non-invasive biomarkers for MPM. However, as well as the requirement for independent validation, either in larger retrospective or preferably in prospective studies, methodology needs to be standardized. For example, it is now abundantly clear that the analysis of plasma and serum is beset by a number of potential sources of error, including hemolysis (56), contamination with blood cells (57) and platelets (58), and difficulties in selecting appropriate normalizer microRNAs (or genes) (59). An alternate to measuring microRNAs themselves has used the observation that miR-34-b/c is frequently methylated in MPM (22), to investigate a digital-PCR-based assay to detect the methylated promoter DNA in the blood (49). This study showed that methylated DNA corresponding to the miR-34-b/c promoter was more frequently detected in patients than those with benign asbestos-related conditions or healthy controls, with an AUC of 0.77 in ROC analysis. Although this initial finding requires further validation, it again shows that a biological change in MPM tumours has the potential to be detected in the peripheral blood.
In summary, the measurement of microRNA levels in patient samples has the potential to help in providing answers to a range of clinical questions. The differential diagnostic test offered by Rosetta Genomics is the most advanced application, but there is significant potential also for microRNA, either singly or as part of a signature, to contribute to diagnosis and prognosis of MPM. Clearly this requires some standardization of methodology and independent validation of reported findings, but this should eventuate with greater collaboration between groups in the field. In addition, combining microRNA quantification with measurements of existing markers (such as mesothelin) may further increase the utility of microRNAs as biomarkers in the clinic.
MicroRNAs as therapeutic targets in MPM
The growing literature surrounding microRNA expression changes in MPM suggests a general downregulation of microRNAs. Many of the studies described in this review have shown that restoring levels of a downregulated microRNA has an inhibitory effect on the proliferation of MPM cells, providing evidence that the microRNAs in question have some degree of tumour suppressor activity. In turn, this suggests that manipulation of microRNA can be developed as a therapeutic approach for MPM. This is particularly attractive as microRNAs typically have many hundreds of gene targets, meaning that altering expression of a single microRNA can potentially re-exert control over multiple pathways dysregulated in MPM.
While the majority of reports describing functional consequences of ectopic microRNA (re-)expression have been limited to in vitro studies, four have shown an in vivo effect of microRNA replacement. The effects of miR-145 were evaluated by implanting mimic-transfected cells into nude mice (28). Only two of eight mice developed tumours compared with 8/8 implanted with control-transfected cells, and the tumours that did form were much smaller. Similarly, when miR-126 transfected MPM cells were implanted in nude mice, they failed to form the tumours seen following implantation of control-plasmid transfected cells (26). However, considering the potent effects of miR-145 (induction of senescence) and miR-126 (inhibition of proliferation and colony formation) shown in vitro, it is likely that these cells were growth arrested shortly after implantation, a common problem with this type of experimental design. A study of the effects of re-expressing miR-34-b/c used an adenoviral vector that was directly injected into established tumours (24). Although the injection yielded a significant increase in tumour miR-34-b/c expression and an inhibition of tumour growth was seen out to 20 days, there was also a considerable effect following injection with adenovirus encoding luciferase. Moreover, it is difficult to envisage that administration via intratumoral injection will be widely applicable to patients.
In the most clinically relevant study to date, a miR-16 mimic was systemically administered to MPM xenograft-bearing mice using an EGFR-targeted bacterial minicell as delivery vehicle (25). The minicells, known as EDVTMnanocells (EDVs), are derived from asymmetric bacterial cell division (60). The targeting is achieved via the use of bispecific antibodies, with one arm specific for the bacterial outer membrane and the other for a tumour antigen, in this case EGFR. EDVs can be loaded with a variety of cargoes, including cytotoxic drugs, plasmids, and siRNA or miRNA mimics (61). In the MPM study, miR-16-loaded EDVs were able to control tumour growth in a dose- and frequency-dependent manner, with the highest dose (administered 4× per week) completely inhibiting tumours (25). This was related to an increase in apoptosis in the tumours, reflecting the in vitro observations. On the basis of these data, a phase I study in MPM and NSCLC patients (‘MesomiR 1’) is currently underway in Sydney, Australia (ACTRN12614001248651). This represents first-in-man studies of a microRNA replacement strategy for thoracic malignancies, and results from the safety and dose-escalation study are eagerly awaited.
Conclusions
The last 5 years has seen a rapid advance in our knowledge of the role of microRNAs in MPM biology. There is now considerable evidence to implicate microRNAs in MPM growth, invasion and drug resistance, and microRNAs in the tumours and blood of patients has revealed a number of biomarker candidates, and the potential application of microRNA-based therapy to the treatment of MPM is another exciting area of research. The coming years will see further development of these findings, and will hopefully be accompanied by improvements in the outcomes for MPM patients.
Acknowledgements
The author gratefully acknowledges the support of a Translational Program Grant from the Cancer Institute NSW, a project grant from the Cancer Council NSW, and previous grants from the Dust Diseases Board NSW. I further thank my colleagues, especially Michaela Kirschner and Nico van Zandwijk for helpful discussions.
Disclosure: The author declares no conflict of interest.
References
- Kao SC, Reid G, Lee K, et al. Malignant mesothelioma. Intern Med J 2010;40:742-50. [PubMed]
- van Meerbeeck JP, Scherpereel A, Surmont VF, et al. Malignant pleural mesothelioma: the standard of care and challenges for future management. Crit Rev Oncol Hematol 2011;78:92-111. [PubMed]
- Tsao AS, Wistuba I, Roth JA, et al. Malignant pleural mesothelioma. J Clin Oncol 2009;27:2081-90. [PubMed]
- van Zandwijk N, Clarke C, Henderson D, et al. Guidelines for the diagnosis and treatment of malignant pleural mesothelioma. J Thorac Dis 2013;5:E254-307. [PubMed]
- Henderson DW, Reid G, Kao SC, et al. Challenges and controversies in the diagnosis of malignant mesothelioma: Part 2. Malignant mesothelioma subtypes, pleural synovial sarcoma, molecular and prognostic aspects of mesothelioma, BAP1, aquaporin-1 and microRNA. J Clin Pathol 2013;66:854-61. [PubMed]
- Henderson DW, Reid G, Kao SC, et al. Challenges and controversies in the diagnosis of mesothelioma: Part 1. Cytology-only diagnosis, biopsies, immunohistochemistry, discrimination between mesothelioma and reactive mesothelial hyperplasia, and biomarkers. J Clin Pathol 2013;66:847-53. [PubMed]
- Jakobsen JN, Sorensen JB. Review on clinical trials of targeted treatments in malignant mesothelioma. Cancer Chemother Pharmacol 2011;68:1-15. [PubMed]
- Bartel DP. MicroRNAs: genomics, biogenesis, mechanism, and function. Cell 2004;116:281-97. [PubMed]
- He L, Hannon GJ. MicroRNAs: small RNAs with a big role in gene regulation. Nat Rev Genet 2004;5:522-31. [PubMed]
- Friedman RC, Farh KK, Burge CB, et al. Most mammalian mRNAs are conserved targets of microRNAs. Genome Res 2009;19:92-105. [PubMed]
- Garzon R, Calin GA, Croce CM. MicroRNAs in Cancer. Annu Rev Med 2009;60:167-79. [PubMed]
- Calin GA, Croce CM. MicroRNA-cancer connection: the beginning of a new tale. Cancer Res 2006;66:7390-4. [PubMed]
- Jansson MD, Lund AH. MicroRNA and cancer. Molecular Oncology 2012;6:590-610. [PubMed]
- Calin GA, Croce CM. MicroRNA signatures in human cancers. Nat Rev Cancer 2006;6:857-66. [PubMed]
- Lu J, Getz G, Miska EA, et al. MicroRNA expression profiles classify human cancers. Nature 2005;435:834-8. [PubMed]
- Busacca S, Germano S, De Cecco L, et al. MicroRNA signature of malignant mesothelioma with potential diagnostic and prognostic implications. Am J Respir Cell Mol Biol 2010;42:312-9. [PubMed]
- Guled M, Lahti L, Lindholm PM, et al. CDKN2A, NF2, and JUN are dysregulated among other genes by miRNAs in malignant mesothelioma -A miRNA microarray analysis. Genes Chromosomes Cancer 2009;48:615-23. [PubMed]
- Pass HI, Goparaju C, Ivanov S, et al. hsa-miR-29c* is linked to the prognosis of malignant pleural mesothelioma. Cancer Res 2010;70:1916-24. [PubMed]
- Ivanov SV, Goparaju CM, Lopez P, et al. Pro-tumorigenic effects of miR-31 loss in mesothelioma. J Biol Chem 2010;285:22809-17. [PubMed]
- Matsumoto S, Nabeshima K, Hamasaki M, et al. Upregulation of microRNA-31 associates with a poor prognosis of malignant pleural mesothelioma with sarcomatoid component. Med Oncol 2014;31:303. [PubMed]
- Khodayari N, Mohammed KA, Goldberg EP, et al. EphrinA1 inhibits malignant mesothelioma tumor growth via let-7 microRNA-mediated repression of the RAS oncogene. Cancer Gene Ther 2011;18:806-16. [PubMed]
- Kubo T, Toyooka S, Tsukuda K, et al. Epigenetic silencing of microRNA-34b/c plays an important role in the pathogenesis of malignant pleural mesothelioma. Clin Cancer Res 2011;17:4965-74. [PubMed]
- Maki Y, Asano H, Toyooka S, et al. MicroRNA miR-34b/cenhances cellular radiosensitivity of malignant pleural mesothelioma cells. Anticancer Res 2012;32:4871-5. [PubMed]
- Ueno T, Toyooka S, Fukazawa T, et al. Preclinical evaluation of microRNA-34b/c delivery for malignant pleural mesothelioma. Acta Med Okayama 2014;68:23-6. [PubMed]
- Reid G, Pel ME, Kirschner MB, et al. Restoring expression of miR-16: a novel approach to therapy for malignant pleural mesothelioma. Ann Oncol 2013;24:3128-35. [PubMed]
- Tomasetti M, Nocchi L, Staffolani S, et al. MicroRNA-126 suppresses mesothelioma malignancy by targeting IRS1 and interfering with the mitochondrial function. Antioxid Redox Signal 2014;21:2109-25. [PubMed]
- Fassina A, Cappellesso R, Guzzardo V, et al. Epithelial-mesenchymal transition in malignant mesothelioma. Mod Pathol 2012;25:86-99. [PubMed]
- Cioce M, Ganci F, Canu V, et al. Protumorigenic effects of mir-145 loss in malignant pleural mesothelioma. Oncogene 2014;33:5319-31. [PubMed]
- Xu Y, Zheng M, Merritt RE, et al. miR-1 induces growth arrest and apoptosis in malignant mesothelioma. Chest 2013;144:1632-43. [PubMed]
- Johnson SM, Grosshans H, Shingara J, et al. RAS is regulated by the let-7 microRNA family. Cell 2005;120:635-47. [PubMed]
- Takamizawa J, Konishi H, Yanagisawa K, et al. Reduced expression of the let-7 microRNAs in human lung cancers in association with shortened postoperative survival. Cancer Res 2004;64:3753-6. [PubMed]
- Tanaka N, Toyooka S, Soh J, et al. Downregulation of microRNA-34 induces cell proliferation and invasion of human mesothelial cells. Oncol Rep 2013;29:2169-74. [PubMed]
- Menges CW, Kadariya Y, Altomare D, et al. Tumor suppressor alterations cooperate to drive aggressive mesotheliomas with enriched cancer stem cells via a p53-miR-34a-c-Met axis. Cancer Res 2014;74:1261-71. [PubMed]
- Aqeilan RI, Calin GA, Croce CM. miR-15a and miR-16-1 in cancer: discovery, function and future perspectives. Cell Death Differ 2010;17:215-20. [PubMed]
- Bonci D, Coppola V, Musumeci M, et al. The miR-15a-miR-16-1 cluster controls prostate cancer by targeting multiple oncogenic activities. Nat Med 2008;14:1271-7. [PubMed]
- Bandi N, Zbinden S, Gugger M, et al. miR-15a and miR-16 are implicated in cell cycle regulation in a Rb-dependent manner and are frequently deleted or down-regulated in non-small cell lung cancer. Cancer Res 2009;69:5553-9. [PubMed]
- Schramm A, Opitz I, Thies S, et al. Prognostic significance of epithelial-mesenchymal transition in malignant pleural mesothelioma. Eur J Cardiothorac Surg 2010;37:566-72. [PubMed]
- Slaby O, Svoboda M, Fabian P, et al. Altered expression of miR-21, miR-31, miR-143 and miR-145 is related to clinicopathologic features of colorectal cancer. Oncology 2007;72:397-402. [PubMed]
- Zaman MS, Chen Y, Deng G, et al. The functional significance of microRNA-145 in prostate cancer. Br J Cancer 2010;103:256-64. [PubMed]
- Nouraee N, Calin GA. MicroRNAs as cancer biomarkers. Microrna 2013;2:102-17. [PubMed]
- Gee GV, Koestler DC, Christensen BC, et al. Downregulated microRNAs in the differential diagnosis of malignant pleural mesothelioma. Int J Cancer 2010;127:2859-69. [PubMed]
- Benjamin H, Lebanony D, Rosenwald S, et al. A diagnostic assay based on microRNA expression accurately identifies malignant pleural mesothelioma. J Mol Diagn 2010;12:771-9. [PubMed]
- Andersen M, Grauslund M, Ravn J, et al. Diagnostic potential of miR-126, miR-143, miR-145, and miR-652 in malignant pleural mesothelioma. J Mol Diagn 2014;16:418-30. [PubMed]
- Kirschner MB, Cheng YY, Armstrong NJ, et al. MiR-score: a novel 6-microRNA signature that predicts survival outcomes in patients with malignant pleural mesothelioma. Mol Oncol 2015;9:715-26. [PubMed]
- Weber DG, Johnen G, Bryk O, et al. Identification of miRNA-103 in the cellular fraction of human peripheral blood as a potential biomarker for malignant mesothelioma--a pilot study. PLoS One 2012;7:e30221. [PubMed]
- Santarelli L, Strafella E, Staffolani S, et al. Association of MiR-126 with soluble mesothelin-related peptides, a marker for malignant mesothelioma. PLoS One 2011;6:e18232. [PubMed]
- Tomasetti M, Staffolani S, Nocchi L, et al. Clinical significance of circulating miR-126 quantification in malignant mesothelioma patients. Clin Biochem 2012;45:575-81. [PubMed]
- Kirschner MB, Cheng YY, Badrian B, et al. Increased circulating miR-625-3p: a potential biomarker for patients with malignant pleural mesothelioma. J Thorac Oncol 2012;7:1184-91. [PubMed]
- Muraoka T, Soh J, Toyooka S, et al. The degree of microRNA-34b/c methylation in serum-circulating DNA is associated with malignant pleural mesothelioma. Lung Cancer 2013;82:485-90. [PubMed]
- Andersen M, Grauslund M, Muhammad-Ali M, et al. Are differentially expressed microRNAs useful in the diagnostics of malignant pleural mesothelioma? APMIS 2012;120:767-9. [PubMed]
- Liu X, Sempere LF, Ouyang H, et al. MicroRNA-31 functions as an oncogenic microRNA in mouse and human lung cancer cells by repressing specific tumor suppressors. J Clin Invest 2010;120:1298-309. [PubMed]
- Murakami H, Mizuno T, Taniguchi T, et al. LATS2 is a tumor suppressor gene of malignant mesothelioma. Cancer Res 2011;71:873-83. [PubMed]
- Sekido Y. Molecular pathogenesis of malignant mesothelioma. Carcinogenesis 2013;34:1413-9. [PubMed]
- van der Bij S, Schaake E, Koffijberg H, et al. Markers for the non-invasive diagnosis of mesothelioma: a systematic review. Br J Cancer 2011;104:1325-33. [PubMed]
- Reid G, Kirschner MB, van Zandwijk N. Circulating microRNAs: Association with disease and potential use as biomarkers. Crit Rev Oncol Hematol 2011;80:193-208. [PubMed]
- Kirschner MB, Kao SC, Edelman JJ, et al. Haemolysis during sample preparation alters microRNA content of plasma. PLoS One 2011;6:e24145. [PubMed]
- Pritchard CC, Kroh E, Wood B, et al. Blood cell origin of circulating microRNAs: a cautionary note for cancer biomarker studies. Cancer Prev Res (Phila) 2012;5:492-7. [PubMed]
- Diehl P, Fricke A, Sander L, et al. Microparticles: major transport vehicles for distinct microRNAs in circulation. Cardiovasc Res 2012;93:633-44. [PubMed]
- Kirschner MB, van Zandwijk N, Reid G. Cell-free microRNAs: potential biomarkers in need of standardized reporting. Front Genet 2013;4:56. [PubMed]
- MacDiarmid JA, Mugridge NB, Weiss JC, et al. Bacterially derived 400 nm particles for encapsulation and cancer cell targeting of chemotherapeutics. Cancer Cell 2007;11:431-45. [PubMed]
- MacDiarmid JA, Brahmbhatt H. Minicells: versatile vectors for targeted drug or si/shRNA cancer therapy. Curr Opin Biotechnol 2011;22:909-16. [PubMed]