Respiratory sleep disorders in patients with congestive heart failure
Introduction
Sleep has a crucial role in defining the pattern of ventilation in patients with congestive heart failure (CHF). This is due to several factors (see Table 1). First, ventilation is under chemical control (arterial CO2 levels) during sleep, where small changes such as 1-2 mmHg can alter respiratory drive enormously and lead to conditions such as central sleep apnea (CSA). In contrast during wakefulness, ventilation is under dual control—cortical and chemical. Cortical control allows speech, swallowing, laughter etc. to synchronize with ventilation.
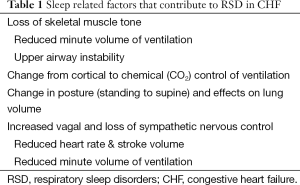
Full table
Second, sleep is characterized by a reduction in skeletal muscle tone which results in loss of postural tone and need to sleep horizontally, but also reduces the respiratory pump muscle activity. The loss of upper airway muscle tone can potentially narrow the upper airway where there is an absence of bone, cartilage or other stabilizing structure causing snoring and/or obstructive sleep apnea (OSA). This will be more common in patients with small airways, such as those with “Asian” craniofacial appearance (1). Moreover, sleep is associated with a change in body position (standing to supine) which has an effect of reducing lung volume.
Finally, sleep is associated with changes to the autonomic nervous system (reduction in sympathetic, rise in parasympathetic activity) and a ~25% reduction in cardiac output and minute volume of ventilation. Each of these factors underpins the potential development of respiratory sleep disorders (RSD) in CHF. RSD occur in ~50% of patients with CHF, equally spread between obstructive and central apnea in type (2). Unfortunately, most cases of apnea are unrecognized (3).
Obstructive sleep apnea (OSA)
Loss of upper airway muscle tone during sleep results in OSA. This condition is characterized by an imbalance between maintaining an open upper airway and the efforts of the respiratory pump musculature. When the upper airway collapses, the respiratory pump (intercostals and diaphragm) vigorously contract yet are unable to create airflow. This can result in significant hypoxemia and small rise in CO2 associated with large powerful negative, yet futile, intrathoracic pleural pressure swings (4) terminated usually 10-60 s later by a rapid change in sleep state, from deep to light sleep, associated with an arousal and restoration of airflow. Huge swings in systemic blood pressure occur plus release of inflammatory proteins and antioxidants which are thought to cause vascular damage. Localized vibrational damage to the carotid endothelium due to the energy produced during snoring, may also lead to atherosclerosis (5).
Central sleep apnea (CSA)
RSD caused by abnormal CO2 control of ventilation can be divided into those with either a reduced ventilatory response to CO2 or an increased ventilatory response to CO2. The clinical scenario of a reduced response is characterized by hypoventilation associated with hypercapnia: this is seen in conditions such as chest wall disease (e.g., kyphoscoliosis), neurological diseases (e.g., motor neurone disease), drug induced (e.g., excessive sedatives) or mechanical factors (e.g., obesity hypoventilation syndrome).
RSD due to an increased ventilatory response to CO2, leading to hypocapnia, is a little more complex and involve usually at least two of three physiological abnormalities: (I) prolonged lung to brain circulatory delay; (II) reduced lung capacity to prevent changes to PaO2 and PaCO2 and (III) an increased ventilatory response to CO2. The prolonged lung to brain circulatory delay is commonly seen in CHF due to reduced cardiac output. In CHF, the circulatory delay is doubled (10-20 s). Reduced lung capacity to buffer swings in PaO2 and PaCO2 levels can be impaired if the lungs are small (restricted) or have impaired gas exchange properties (thickened basement membrane).
The heightened ventilatory response to CO2 can involve the central (brainstem) or peripheral (carotid body) chemoreceptors (6). RSD associated with heightened ventilatory response to CO2 are commonly seen in CHF, where it is known as CSA with a Cheyne Stokes pattern of Respiration (CSA-CSR). PaCO2 levels oscillate above and below the apneic threshold thereby causing the typical hyperventilation and central apnea pattern. Hyperventilation and hypocapnia are common in such patients and is a useful guide to identify CSA-CSR (7). RSD of a similar pattern are seen in non-CHF conditions, such as periodic breathing at high altitude in normal subjects, premature infancy, and continuous positive airway pressure (CPAP) emergent CSA and narcotic ingestion.
Congestive heart failure (CHF)
CHF is defined as a syndrome of inadequate cardiac output to meet requirements, which is associated with symptoms (dyspnea, fatigue) and an objective cardiac abnormality. It can be caused by disorders of the left ventricular pump (commonly classified as either systolic vs. diastolic failure or idiopathic vs. ischaemic), arrhythmias (bradycardia, sick sinus syndrome, atrial fibrillation, heart block) and valvular disorders (8). Less common disorders such as pericardial diseases and myocardial infiltrative (e.g., amyloid) also need to be considered.
Understanding the cause of CHF is important when dealing with RSD, as there are bidirectional effects between CHF and RSD. Moreover some therapies common to sleep physicians (e.g., CPAP) may be effective in treating some causes of CHF (e.g., dilated cardiomyopathy) but not in others (e.g., aortic stenosis, mitral valve prolapse) (see Figure 1). In some cases, the CHF contributes to RSD, whereas in other RSD contribute to CHF. Table 2 provides clinical indicators of OSA and CSA in patients with CHF.
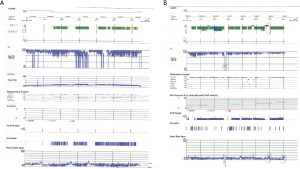
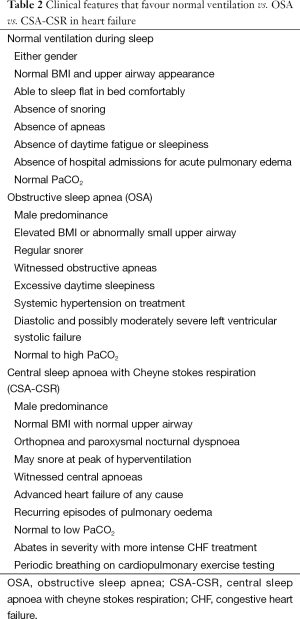
Full table
Respiratory sleep disorders (RSD) contributing to CHF
OSA is the main RSD of concern in relation to cause of CHF. Research has provided the greatest understanding of its downstream cardiovascular effects.
Development of systemic hypertension as a precursor to CHF has been attributed to impaired baroreceptor control, impaired release of vasodilator endothelial nitric oxide and a generalized increase in sympathetic activity (9). Initially patients with OSA lose the usual nocturnal dip in systemic blood pressure and later they develop awaked hypertension and thereafter drug resistant hypertension (defined as needing at least three antihypertensive drug therapies). Thickening of the vascular media wall in the carotid arteries, whilst sparing the femoral arteries, has raised the possibility of snoring and vibrational damage to the endothelium (5).
Episodic atrial fibrillation is also common in patients with untreated OSA possibly related to surges in sympathetic activity whilst hypoxia and hypercapnia are present and the atrial walls are stretched. Atrial fibrillation is associated with ~25% reduction in cardiac output.
Coronary artery disease is not only more common in OSA patients, but it may be detected during polysomnography with ST depression on electrocardiograph (ECG). In patients with an established myocardial infarction, the relative risk of OSA is equivalent to a history of cigarette smoking (10).
Stroke also occurs more commonly in OSA patients although the responsible mechanisms are not well understood. Theories include paradoxical emboli via transient opening of shunts (e.g., Patent Foramen Ovale), extreme swings in blood pressure beyond the levels that cerebral autoregulation can cope with, atrial fibrillation and atheromatous plaque rupture due to large intravascular and extravascular pressure swings.
CHF induced by OSA has also been observed based upon animal experiments (11), cross sectional and prospective epidemiological studies and human interventional studies. A toxic combination of repetitive hypoxemia, increases in afterload (negative intrathoracic pressures and increased systemic blood pressure) and recurring arousals are thought to be contributory.
The evidence in favor of treating patients with both CHF and OSA is based primarily upon two randomized controlled trials (12,13): which in combination highlight improvement in objective markers and symptoms of cardiac function, whilst improvements in mortality are yet to be determined (Table 3). The SAVE trial (NCT00738179) of long term treatment of OSA with CPAP in high cardiovascular risk patients may provide the answer (14).
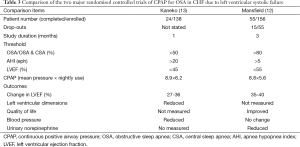
Full table
CHF contributing to RSD
CHF may result in fluid retention and deranged autonomic control. Commonly, patients with CHF complain of dyspnea on exertion, then at rest or during sleep, where it is known as orthopnea or paroxysmal nocturnal dyspnea. Fluid retention results in interstitial edema, pleural effusion and alveolar edema. There may also be pedal edema and at times gut wall edema may lead to malabsorption.
Deranged autonomic control (heightened sympathetic and diminished vagal tone) is thought initially to be compensatory for the low cardiac output. This has an effect upon sleep quality [reduced sleep efficiency, paucity of slow wave and REM sleep, periodic limb movements] in addition to the development of tachyarrhythmias (atrial fibrillation, ventricular tachycardia, and ventricular fibrillation). Poor sleep quality can lead to daytime “fatigue”, rather than true “sleepiness” (15).
Fluid retention can contribute to RSD via several mechanisms. First edema of the upper airway can narrow the lumen (and increase nasal resistance), and possibly the surface tension of the upper airway lining thus predisposing to upper airway collapse (snoring and obstructive apneas and hypopneas).
Second, shifting of fluid from lower limbs and abdomen to the thorax can contribute to transient pulmonary edema. This phenomenon is called “rostral fluid shift” (16) and if concentrated to the lungs cause CSA-CSR, or to the upper airway, OSA. Given that 50% of the oxygen stores are kept within the lungs, a reduction in size (total lung capacity) or function (altered gas exchange due to interstitial edema) can exacerbate periodic breathing (17).
Classically, lung function tests of patients with recurring episodes of acute heart failure have a restrictive ventilatory defect with a reduced diffusing capacity. In more extreme cases (e.g., mitral stenosis in youth) calcified pulmonary nodules and interstitial pulmonary fibrosis may be seen and represent the most severe form of pulmonary damage due to recurring pulmonary edema (17).
Third, treatments directed at fluid retention (e.g., diuretics) can alter upper airway surfactant properties (dry mouth) predisposing to upper airway collapse. Also, diuretic induced metabolic alkalosis can occasionally cause a compensatory hypoventilation and respiratory acidosis.
Deranged autonomic nervous system is most likely due to underlying CHF with a secondary RSD (18). Increased sympathetic activity causes hyperventilation, which underpins CSA-CSR. Whether the heightened sympathetic activity is caused by CSA-CSR or simply the underlying CHF is a point of debate. In the one study to address the issue (18), the severity of heart failure was a greater contributor to sympathetic activity than was the severity of CSA-CSR.
The increased ventilatory response to CO2, coupled with prolonged lung to brain circulatory delay (low cardiac output) and abnormal lung function (pulmonary edema) leads to a cyclic breathing pattern (19). Thirty seconds periods of hyperventilation are interspersed with 20-30 s periods of central apnea in a crescendo decrescendo pattern. CSA is often triggered by an arousal or sudden state change. An arousal occurs at the peak of ventilation. Often there is an absence of hypoxemia. Concurrent periodic limb movements are common. Usually CSA-CSR is worse with transition from wake to stages 1 and 2 non-REM sleep and less so in slow wave and REM sleeps. Of interest, as with OSA, it is more common in the supine position, less in the lateral position (20) and diminished further when the head of the bed is raised (21).
If one measure heart rate and blood pressure continuously in patients with CSA-CSR, one will see that the cardiorespiratory pattern has a cycle length of about 60 s with rise of ventilation associated with a rise in blood pressure and heart rate (Figure 2). Males appear to be over represented in the CSA-CSR populations for reasons that are unclear: perhaps related to a greater respiratory muscle mass and the ability to increase minute volume of ventilation sufficiently to drive the CO2 level below the apneic threshold.
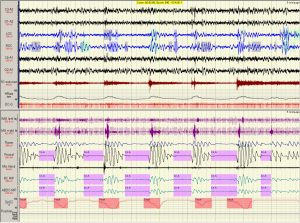
An important distinction with CSA-CSR is the cycle length, which includes the apnea and the hyperventilation (hyperpnoea) component (Figure 3) (22,23). Two aspects need highlighting. The first is that the ratio of the hyperpnoea to apnea is usually >1 in CHF. Moreover the duration of the cycle length is 45-75 s in CHF (Figure 3A). In contrast, non CHF causes of CSA have a hyperpnoea to apnea ration of <1 and a cycle length 20-40 s (Figure 3B). This ratio can assist in understanding loop gain, a descriptive term for periodic breathing (24,25). The cause of non-CHF CSA, such as that due to narcotic ingestion, or unknown causes (so called “idiopathic”) can be distinguished from CHF induced CSA-CSR by the pattern of ventilation seen on the polysomnogram. Similar short cycle length CSA can be seen with CPAP initiation (which usually resolves over 1-3 months duration), at high altitude in normal and premature infants.
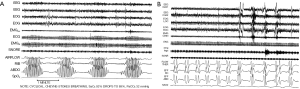
Is CSA-CSR detrimental?
Whether CSA-CSR contributes to the development of CHF has not been proven (26). Some evidence exists that the periodic hyperventilation indeed assists cardiac output (27,28) and that the hyperventilation results in an increase in end-expiratory lung volume (29) thereby offsetting the restrictive ventilatory defect and creating a respiratory alkalosis which may prevent the development of hypercapnic acidosis. Acidosis has a far worse effect on the heart’s contractility in the setting of hypoxemia than does alkalosis (26). Recent unpublished research from our laboratory, where we compared minute volume of ventilation and intrathoracic pressure swings during sleep in CSA-CSR and OSA indicated that both apnea types have similar degrees of work of breathing (pressure × volume), however CSA-CSR does so with greater efficiency (pressure × time).
Instead, CSA-CSR is a marker of advanced heart failure. Elevated pulmonary capillary wedge pressure (30), BNP and noradrenaline levels (31,32), low left ventricular ejection fraction (LVEF) and other markers of unstable or advanced CHF have been shown to occur with CSA-CSR. Thus when recognized, it should trigger attention to determine whether more aggressive therapy is required to combat the CHF. This might include sleeping upright (19), or laterally (20), greater afterload reduction (vasodilators), preload reduction [diuretics such as acetazolamide (33)], inotropes, surgery (coronary artery graft surgery, valvular repair or replacement), rhythm correction, pacemakers [atrial overdrive (34), biventricular (35)], left ventricular assist device (36) or even heart transplantation (37).
Continuous positive airway pressure (CPAP)
Included in the regime of more aggressive therapy towards CHF is CPAP. Although commonly used for OSA, the mechanisms of action in CHF with CSA-CSR differ and may be limited to a subset of patients in whom the cause of CHF is amenable to CPAP (such as a dilated cardiomyopathy, but not aortic stenosis (Figure 1). The actions of CPAP can be divided into (I) upper airway pneumatic dilating; (II) pulmonary and (III) cardiac (Table 4).
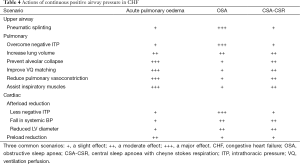
Full table
The pulmonary effects of CPAP include increasing lung volume, preventing alveolar collapse and improving oxygen storage and gas transfer and bronchodilation (38). Assistance with respiratory muscles and abolition of negative intrathoracic pressure has an estimated 40% reduction in the work of breathing (39), which will minimize the cardiac output required by the respiratory muscles. Thus, CPAP may reduce the cardiac output “steal” phenomenon by the overworked respiratory muscles during sleep (40). It is also possible that CPAP induces improved ventilation perfusion (VQ) matching, reduces acute pulmonary hypertension and thereby prevents intermittent shunting through patent foramen ovale (or similar). In addition CPAP may also provide a small but significant rise in dead space, thus increasing CO2 levels. Importantly minute ventilation falls and PaCO2 levels rise in patients with CSA-CSR treated with CPAP therapy.
The cardiac effects of CPAP include reductions in left ventricular afterload (38) and preload. The afterload falls because the negative intrathoracic pressure is replaced by positive pressure, upstream blood pressure falls (less sympathetic activity) and the cardiac chamber size is reduced (41). Reductions in cardiac sympathetic activity (42) and myocardial oxygen demand have been reported immediately (43) and the long term (44). Preload may also fall by reducing venous return to the thorax.
Thus the effects of CPAP are complex and are likely to differ in the following settings: OSA, CSA-CSR and acute cardiogenic pulmonary edema (Table 4). Also some CHF may not be amenable to CPAP particularly if dry or CHF due to some valvular or rhythm disturbances (45).
The main trial conducted to date of 2-years of CPAP treatment in 258 CHF patients with CSA-CSR (CANPAP trial) did show improvements in apnea hyponea index (AHI), oxygenation, 6 minutes-walk distance, LVEF and plasma norepinephine, however there was no overall difference in hospitalizations, quality of life or transplant free survival (46). A post hoc analysis, dividing those patients who had a fall in AHI to <15 at 3 months with CPAP did have a survival benefit (47).
Whether adaptive servo controlled ventilation (ASV) support is better than CPAP has not been proven (47). ASV provides CPAP with variable ventilatory support which increases during the central apneas (48). Two large clinical trials comparing ASV with best practice (NCT01128816 & NCT00733343) are underway. The preliminary results of one study with 1325 subjects followed for over 2 years in 267 study locations, the SERVE-HF study indicated a 2.5% absolute increased annual risk of cardiovascular mortality for those randomised to ASV therapy compared with the control group (49). However the primary endpoint (all-cause mortality or unplanned hospitalization for worsening heart failure) was not significantly different. Reasons for the increased cardiovascular mortality with ASV are unclear; possibilities include inadequate preload, which is necessary for positive airway pressure therapy.
Oxygen therapy
Oxygen therapy is often considered in patients with CHF. Correction of hypoxemia (SpO2 <90%) is important and can be achieved usually with CPAP or supplemental oxygen therapy. An optimal SpO2 value for patients with CHF is 92-95%. However, most patients with CHF are not hypoxic and if they are (such as during acute pulmonary edema), the oxygen levels promptly rise to SpO2 values >92% with CPAP (at a level of approximately 10% of body weight, i.e., 70 kg body weight =7 cmH2O CPAP). Moreover, supplemental oxygen therapy has not been shown to improve cardiac function in any clinical trial. Indeed the converse is true. Two studies of oxygen therapy in CHF have shown the cardiac output to fall and pulmonary capillary wedge pressure to rise (50,51). In addition, supplemental oxygen has not been shown to be of benefit in myocardial infarction. Complications of excessive supplemental oxygen use are starting to emerge beyond the well-recognized adverse effects of hypercapnia and pulmonary fibrosis. Thus supplemental oxygen is not routinely recommended in normoxic CHF patients (52).
Case study
A 68-year-old male, previous smoker developed a myocardial infarct in 2004, then atrial fibrillation and pulmonary edema in 2005 requiring a pacemaker. Associated iron deficient anemia related to peptic ulcer was noted. He had progressive dyspnea needing hospitalization in 2014 for heart transplant assessment. There was a history of snoring and witnessed apneas extending for more than 10 years. New York Heart Association class 3. His Epworth sleepiness score was 7 (normal <10, abnormal 10-24).
Examination revealed BMI 24 and crowded oropharynx (Mallampati 3). LVEF by nuclear gated scan was 15%, and a right heart catheter study revealed an elevated pulmonary capillary wedge pressure of 21 mmHg. Lung function tests indicated normal spirometry and static lung volumes, yet reduced TLCO at 62% predicted. High resolution scan did not identify emphysema (Figure 4). Cardiopulmonary exercise test indicated a heightened VE to VO2 slope, an absence of hypoxemia and reduced maximal oxygen consumption (VO2 peak) of 12.6 mL/min/kg (Figure 5).
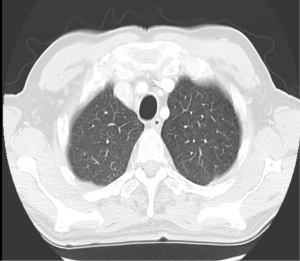
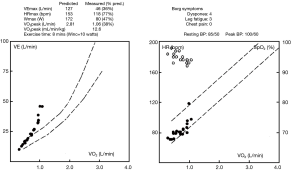
Two overnight polysomnograms indicated severe sleep apnoea on the diagnostic night: central during non REM sleep and obstructive during REM sleep and PaCO2 levels were 37 and 35 mmHg at the beginning and end of the diagnostic study. Transcutaneous PCO2 levels were <37 mmHg across the night. There was a marked improvement during the CPAP study less than 4 weeks later (Figures 6-8). Note there was no significant weight change (84-86 kg). With CPAP, there was an increase in supine position (56-96% of the night) and slow wave (11-28% of total sleep time) sleep. The AHI fell (50-16 events per hour) and minimum SpO2 levels rose (70-89%). Significant objective and symptomatic improvements in heart failure followed.
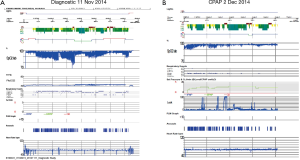
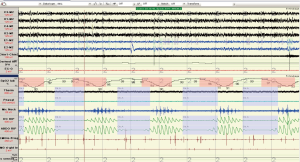
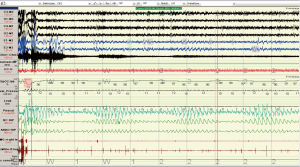
This case highlights (I) the under recognition of disorders of respiration during sleep in high risk patients (ischemic heart disease, atrial fibrillation); (II) the overlap of central and obstructive sleep apnoea within the same patient and (III) the immediate response to CPAP in patients with ischemic dilated cardiomyopathy.
Conclusions
Identification of RSD is important in patients with CHF. On one hand, identification and treatment of OSA may improve cardiac function both objectively and symptomatically. On the other hand, identification of CSA is indicative of advanced heart failure with subacute pulmonary edema and the need to augment therapy directed towards the underlying heart failure. This therapy may include pharmacological, surgical and device options (including CPAP). Oxygen therapy should not be used routinely, unless hypoxemic and unresponsive to standard CHF therapies mentioned above.
Acknowledgements
None.
Footnote
Conflicts of Interest: The author has no conflicts of interest to declare.
References
- Liu Y, Lowe AA, Zeng X, et al. Cephalometric comparisons between Chinese and Caucasian patients with obstructive sleep apnea. Am J Orthod Dentofacial Orthop 2000;117:479-85. [PubMed]
- Yumino D, Wang H, Floras JS, et al. Prevalence and physiological predictors of sleep apnea in patients with heart failure and systolic dysfunction. J Card Fail 2009;15:279-85. [PubMed]
- Javaheri S, Caref EB, Chen E, et al. Sleep apnea testing and outcomes in a large cohort of Medicare beneficiaries with newly diagnosed heart failure. Am J Respir Crit Care Med 2011;183:539-46. [PubMed]
- Suzuki M, Ogawa H, Okabe S, et al. Digital recording and analysis of esophageal pressure for patients with obstructive sleep apnea-hypopnea syndrome. Sleep Breath 2005;9:64-72. [PubMed]
- Lee SA, Amis TC, Byth K, et al. Heavy snoring as a cause of carotid artery atherosclerosis. Sleep 2008;31:1207-13. [PubMed]
- Solin P, Roebuck T, Johns DP, et al. Peripheral and central ventilatory responses in central sleep apnea with and without congestive heart failure. Am J Respir Crit Care Med 2000;162:2194-200. [PubMed]
- Naughton M, Benard D, Tam A, et al. Role of hyperventilation in the pathogenesis of central sleep apneas in patients with congestive heart failure. Am Rev Respir Dis 1993;148:330-8. [PubMed]
- Dickstein K, Cohen-Solal A, Filippatos G, et al. ESC guidelines for the diagnosis and treatment of acute and chronic heart failure 2008: the Task Force for the Diagnosis and Treatment of Acute and Chronic Heart Failure 2008 of the European Society of Cardiology. Developed in collaboration with the Heart Failure Association of the ESC (HFA) and endorsed by the European Society of Intensive Care Medicine (ESICM). Eur Heart J 2008;29:2388-442. [PubMed]
- Brooks D, Horner RL, Kozar LF, et al. Obstructive sleep apnea as a cause of systemic hypertension. Evidence from a canine model. J Clin Invest 1997;99:106-9. [PubMed]
- Hung J, Whitford EG, Parsons RW, et al. Association of sleep apnoea with myocardial infarction in men. Lancet 1990;336:261-4. [PubMed]
- Parker JD, Brooks D, Kozar LF, et al. Acute and chronic effects of airway obstruction on canine left ventricular performance. Am J Respir Crit Care Med 1999;160:1888-96. [PubMed]
- Mansfield DR, Gollogly NC, Kaye DM, et al. Controlled trial of continuous positive airway pressure in obstructive sleep apnea and heart failure. Am J Respir Crit Care Med 2004;169:361-6. [PubMed]
- Kaneko Y, Floras JS, Usui K, et al. Cardiovascular effects of continuous positive airway pressure in patients with heart failure and obstructive sleep apnea. N Engl J Med 2003;348:1233-41. [PubMed]
- Antic NA, Heeley E, Anderson CS, et al. The sleep apnea cadiovascular endpoints (SAVE) trial: Rationale, Ethics, Design and Progress. Sleep 2015;38:1247-57. [PubMed]
- Arzt M, Young T, Finn L, et al. Sleepiness and sleep in patients with both systolic heart failure and obstructive sleep apnea. Arch Intern Med 2006;166:1716-22. [PubMed]
- Yumino D, Redolfi S, Ruttanaumpawan P, et al. Nocturnal rostral fluid shift: a unifying concept for the pathogenesis of obstructive and central sleep apnea in men with heart failure. Circulation 2010;121:1598-605. [PubMed]
- Kee K, Naughton MT. Heart failure and the lung. Circ J 2010;74:2507-16. [PubMed]
- Mansfield D, Kaye DM, Brunner La Rocca H, et al. Raised sympathetic nerve activity in heart failure and central sleep apnea is due to heart failure severity. Circulation 2003;107:1396-400. [PubMed]
- Szollosi I, Thompson BR, Krum H, et al. Impaired pulmonary diffusing capacity and hypoxia in heart failure correlates with central sleep apnea severity. Chest 2008;134:67-72. [PubMed]
- Szollosi I, Roebuck T, Thompson B, et al. Lateral sleeping position reduces severity of central sleep apnea/Cheyne-Stokes respiration. Sleep 2006;29:1045-51. [PubMed]
- Altschule MD, Iglauer A. The effect of position on periodic breathing in chronic cardiac decompensation. N Engl J Med 1958;259:1064-6. [PubMed]
- Solin P, Jackson DM, Roebuck T, et al. Cardiac diastolic function and hypercapnic ventilatory responses in central sleep apnoea. Eur Respir J 2002;20:717-23. [PubMed]
- Solin P, Roebuck T, Swieca J, et al. Effects of cardiac dysfunction on non-hypercapnic central sleep apnea. Chest 1998;113:104-10. [PubMed]
- Naughton MT. Loop gain in apnea: gaining control or controlling the gain? Am J Respir Crit Care Med 2010;181:103-5. [PubMed]
- Sands SA, Edwards BA, Kee K, et al. Loop gain as a means to predict a positive airway pressure suppression of Cheyne-Stokes respiration in patients with heart failure. Am J Respir Crit Care Med 2011;184:1067-75. [PubMed]
- Naughton MT. Cheyne-Stokes respiration: friend or foe? Thorax 2012;67:357-60. [PubMed]
- Maze SS, Kotler MN, Parry WR. Doppler evaluation of changing cardiac dynamics during Cheyne-Stokes respiration. Chest 1989;95:525-9. [PubMed]
- Yumino D, Kasai T, Kimmerly D, et al. Differing effects of obstructive and central sleep apneas on stroke volume in patients with heart failure. Am J Respir Crit Care Med 2013;187:433-8. [PubMed]
- Brack T, Jubran A, Laghi F, et al. Fluctuations in end-expiratory lung volume during Cheyne-Stokes respiration. Am J Respir Crit Care Med 2005;171:1408-13. [PubMed]
- Solin P, Bergin P, Richardson M, et al. Influence of pulmonary capillary wedge pressure on central apnea in heart failure. Circulation 1999;99:1574-9. [PubMed]
- Naughton MT, Benard DC, Liu PP, et al. Effects of nasal CPAP on sympathetic activity in patients with heart failure and central sleep apnea. Am J Respir Crit Care Med 1995;152:473-9. [PubMed]
- Solin P, Kaye DM, Little PJ, et al. Impact of sleep apnea on sympathetic nervous system activity in heart failure. Chest 2003;123:1119-26. [PubMed]
- Javaheri S. Acetazolamide improves central sleep apnea in heart failure: a double-blind, prospective study. Am J Respir Crit Care Med 2006;173:234-7. [PubMed]
- Garrigue S, Bordier P, Jaïs P, et al. Benefit of atrial pacing in sleep apnea syndrome. N Engl J Med 2002;346:404-12. [PubMed]
- Sinha AM, Skobel EC, Breithardt OA, et al. Cardiac resynchronization therapy improves central sleep apnea and Cheyne-Stokes respiration in patients with chronic heart failure. J Am Coll Cardiol 2004;44:68-71. [PubMed]
- Harun NS, Leet A, Naughton MT. Improvement in sleep-disordered breathing after insertion of left ventricular assist device. Ann Am Thorac Soc 2013;10:272-3. [PubMed]
- Mansfield DR, Solin P, Roebuck T, et al. The effect of successful heart transplant treatment of heart failure on central sleep apnea. Chest 2003;124:1675-81. [PubMed]
- Barach AL, Swenson P. Effect of breathing gases under positive pressure on lumens of small and medium sized bronchi. Arch Intern Med 1939;63:946-48.
- Naughton MT, Rahman MA, Hara K, et al. Effect of continuous positive airway pressure on intrathoracic and left ventricular transmural pressures in patients with congestive heart failure. Circulation 1995;91:1725-31. [PubMed]
- Robertson CH Jr, Pagel MA, Johnson RL Jr. The distribution of blood flow, oxygen consumption, and work output among the respiratory muscles during unobstructed hyperventilation. J Clin Invest 1977;59:43-50. [PubMed]
- Leithner C, Podolsky A, Globits S, et al. Magnetic resonance imaging of the heart during positive end-expiratory pressure ventilation in normal subjects. Crit Care Med 1994;22:426-32. [PubMed]
- Kaye DM, Mansfield D, Aggarwal A, et al. Acute effects of continuous positive airway pressure on cardiac sympathetic tone in congestive heart failure. Circulation 2001;103:2336-8. [PubMed]
- Kaye DM, Mansfield D, Naughton MT. Continuous positive airway pressure decreases myocardial oxygen consumption in heart failure. Clin Sci (Lond) 2004;106:599-603. [PubMed]
- Hall AB, Ziadi MC, Leech JA, et al. Effects of short-term continuous positive airway pressure on myocardial sympathetic nerve function and energetics in patients with heart failure and obstructive sleep apnea: a randomized study. Circulation 2014;130:892-901. [PubMed]
- Forrester JS, Diamond GA, Swan HJ. Correlative classification of clinical and hemodynamic function after acute myocardial infarction. Am J Cardiol 1977;39:137-45. [PubMed]
- Bradley TD, Logan AG, Kimoff RJ, et al. Continuous positive airway pressure for central sleep apnea and heart failure. N Engl J Med 2005;353:2025-33. [PubMed]
- Arzt M, Floras JS, Logan AG, et al. Suppression of central sleep apnea by continuous positive airway pressure and transplant-free survival in heart failure: a post hoc analysis of the Canadian Continuous Positive Airway Pressure for Patients with Central Sleep Apnea and Heart Failure Trial (CANPAP). Circulation 2007;115:3173-80. [PubMed]
- Aurora RN, Chowdhuri S, Ramar K, et al. The treatment of central sleep apnea syndromes in adults: practice parameters with an evidence-based literature review and meta-analyses. Sleep 2012;35:17-40. [PubMed]
- Available online: http://www.resmed.com/content/dam/resmed/global/documents/serve-hf/Healthcare_providers_SERVE-HF_FAQs.pdf
- Haque WA, Boehmer J, Clemson BS, et al. Hemodynamic effects of supplemental oxygen administration in congestive heart failure. J Am Coll Cardiol 1996;27:353-7. [PubMed]
- Mak S, Azevedo ER, Liu PP, et al. Effect of hyperoxia on left ventricular function and filling pressures in patients with and without congestive heart failure. Chest 2001;120:467-73. [PubMed]
- Clark AL, Johnson MJ, Squire I. Does home oxygen benefit people with chronic heart failure? BMJ 2011;342:d234. [PubMed]