Retrolaminar versus epidural block for postoperative analgesia after minor video-assisted thoracic surgery: a retrospective, matched, non-inferiority study
Introduction
Thoracic epidural analgesia (TEA) or thoracic paravertebral block (TPVB) have been used as gold standard analgesia for thoracic surgery (1-5). TEA and TPVB provide comparable pain relief after thoracic surgery (4,6). TEA protect against pulmonary complications than intravenous opioid administration (2). However, TEA carries the risk of complications such as hypotension, epidural abscess, spinal hematoma and neurological injuries (1). TPVB reduces the risks of hypotension, urinary retention and other minor complications compared to TEA (5). However, TPVB has similar contraindications including coagulation disorders and anticoagulation (3,7), and other major complications such as serious neurologic injuries same as TEA (3).
Video-assisted thoracic surgery (VATS) is a minimally invasive technique and has been widely performed with its advantages of reduced postoperative morbidity and early recovery. VATS reduces postoperative pain scores compared with that of open thoracotomy. However, VATS is still associated with moderate to severe postoperative pain requiring treatment (8,9). Several studies described different analgesic technique for VATS. There is no consensus regarding optimal postoperative pain control after VATS. Although, the role of TEA and TPVB for postoperative analgesia after VATS is controversial (10), the majority still prefer TEA compared with other regional analgesia for the postoperative analgesia after VATS (11).
Minor VATS procedures, including VATS bullectomy and wedge resection for lung cancer, were performed using smaller incisions and leading to shorter hospital stay compared to those of VATS lobectomy (12). Although it is not known whether minor VATS procedures are associated with less perioperative pain scores compared with that of VATS lobectomy, it should have less-invasive analgesia with less complications.
Ultrasound-guided retrolaminar block (RLB) (13,14) are new thoracic truncal blocks. RLB are considered to be easy, less invasive and safe maneuvers compared to TEA, erector spinae plane block (ESPB) and TPVB (15). Although RLB can be performed without ultrasonography, ultrasound images allow for visualization of the needle, catheter and local anesthetic distribution. Some studies reported the application of RLB for chest wall surgery (16-19). RLB provided effective analgesia in cases of multiple rib fractures (13). Continuous infusion of local anesthetics through catheter prolongs the analgesic effects. However, there were no clinical reports that compare the analgesic efficacy of continuous RLB with that of TEA after thoracic surgery. We hypothesized that ultrasound-guided continuous RLB might provide analgesia as effective as that of TEA for minor VATS procedures. Thus, the purpose of this retrospective propensity-matched study was to compare the postoperative analgesic efficacy and adverse effects of ultrasound-guided continuous RLB with those of TEA in patients undergoing minor VATS procedures.
We present the following article in accordance with the STROBE reporting checklist (available at http://dx.doi.org/10.21037/jtd-21-238).
Methods
Ethics, study participant enrollment
This study was conducted in accordance with the Declaration of Helsinki (as revised in 2013). This study protocol was examined and approved by the Research Review Board at St. Mary’s Hospital on 12 January, 2017 (Approval No., #16-1205). Written informed consent was obtained from all patients who participated in this study.
We enrolled the patients with American Society of Anesthesiologists physical status (ASA-PS) 1–2 who were scheduled for minor VATS procedures (bullectomy, partial lung resection and mediastinal tumor resection) at St. Mary’s Hospital from February 2017 to December 2019. Exclusion criteria included patients with bilateral pneumothorax, bilateral thoracic surgery, redo surgery, chronic pain, preoperative use of opioid or pain medication, history of substance use, alcohol abuse, ASA-PS 3 or greater, younger than 15 years old, coagulation abnormalities (platelet count less than 100×103/µL, prothrombin time-international normalized ratio (PT-INR) greater than 1.2 or activated partial thromboplastin time (APTT) longer than normal range), allergy to any drug used in this study, and inability to provide informed consent.
Ultrasound-guided RLB and TEA
Ultrasound-guided RLB catheterization was performed under general anesthesia after the end of surgery in the lateral position using a linear ultrasound probe (15–6 MHz; S-NerveTM, FUJIFILM SonoSite, Bothell, Washington).
The RLB was performed as previously described (13). In the RLB group, a 17-G Tuohy epidural needle (Perican®; B-Braun Medical, Melsungen GA, Germany) was inserted using in-plane ultrasound view, from cephalad to caudal direction at 1 cm lateral to the spinous process at T4 or T5, where the main surgical incision was made. After the confirmation hydro-dissection (normal saline) of the interfascial plane, the mixture of ropivacaine 0.75% 10 mL plus lidocaine 1% with 1:100,000 epinephrine 10 mL were administered when the needle tip reached the lamina. After the placement of the catheter (FX catheter®; B-Braun Medical, Melsungen GA, Germany) 5.0 cm beyond the needle tip, ropivacaine 0.2% (8–12 mL/h) was continuously administered using a balloon injector (Rakuraku fuser ambulatory disposable pump®, Smiths Medical, Minneapolis, MN, USA) until the chest tube was removed.
The epidural catheter was placed without sedation before induction of general anesthesia via a paramedian approach in the lateral decubitus position. After local anesthesia with 1% lidocaine, a Tuohy 17-G epidural needle was inserted at T4/5 or T5/6 intervertebral space. Epidural space was identified by the loss of resistance technique. The catheter was advanced 4 to 5 cm into the epidural space. After a test aspiration was negative, a 3-mL test dose of 1% lidocaine was administered. Postoperative analgesia was initiated with a single shot administration of ropivacaine 0.375% (3–6 mL) at the end of surgery. Ropivacaine 0.2% (3–6 mL/h) was continuously administered until the chest tube was removed.
The responsible anesthesiologists decided the choice of analgesia and dose for continuous infusion of local anesthetics. Patient controlled regional analgesia was not performed in this study. If adverse events such as post-operative nausea and vomiting (PONV) occurred, the local anesthetic flow was stopped or decreased until the event subsided, and then the flow was resumed. The decision was made by the anesthesiologists or surgeons.
General anesthesia
Premedication and pre-operative multi-modal pain medication were not given to the patients. A standard monitor was attached to the patient after entering the operating room. General anesthesia was induced with propofol (1–2 mg/kg), fentanyl (50 µg) and rocuronium (0.6 mg/kg). A left-sided double-lumen endobronchial tube (ShileyTM, Covidien, Mansfield, MA, USA) was placed for one lung ventilation, and its correct position was confirmed by auscultation and fiber-optic bronchoscopy. The maintenance of anesthesia consisted of balanced anesthesia of inhalational anesthesia (sevoflurane, desflurane) with remifentanil, or propofol with remifentanil. Intravenous fentanyl and rocuronium were administered as needed. Acetaminophen or flurbiprofen were administered intravenously at skin closure. Administration of inhalational anesthesia, propofol and remifentanil were discontinued upon completion of the surgery, and sugammadex was administered to reverse the muscle relaxation induced by rocuronium. Fentanyl (50 µg) or pethidine (10 mg) was administered incrementally if there was request of rescue analgesia after awaking from anesthesia. The patient was discharged from the operating theater and sent to the ward after achieving an appropriate level of consciousness and analgesia. Narcotic rescue usage is standardized to morphine milligram equivalent (MME; morphine:pethidine:fentanyl =1:10:0.01) (20). Narcotics were only available for patients in the operating room.
Postoperative pain management
In our institute, preoperative multimodal pain management was not performed. Regular postoperative analgesics consisted of loxoprofen sodium hydrate (60 mg) three times daily. Intravenous flurbiprofen or acetaminophen were used for rescue analgesia when the pain was not well controlled (NRS
The postoperative pain intensity was assessed by the numerical rating scale (NRS) scores at 6 and 12 h after surgery, the morning and evening of postoperative day (POD) 1 and the morning of POD 2. NRS scores were recorded at rest (during quiet breathing) and when coughing.
Surgical procedures
In our institute, a three-port technique was used for VATS port-site strategy. The 6th to 8th intercostal spaces were used for the placement of the inferior port for videoscope access and chest tube placement. The 4th to 5th intercostal spaces were used for the placement of the anterior and posterior ports. The incision site was limited between the 4th and 8th intercostal spaces. All surgeries were performed by the same operation team.
Outcomes
The primary outcome of this study was the median differences in the NRS scores between the TEA and RLB groups during rest at the morning of postoperative day 1 (POD 1m). The secondary outcome parameters were NRS scores at rest and when coughing, postoperative initial narcotic rescue usage, rescue analgesics requirement in the ward, and adverse effects including incidence of hypotension, pruritus, urinary retention, PONV, and the length of postoperative hospitalization. In routine practice, the nursing staff asked the patients about pruritus, urinary retention, nausea and pain intensity. NRS scores were recorded 6 and 12 h after surgery, POD 1m, the evening of POD 1 (POD 1e) and the morning of POD 2 (POD 2m). Hypotension was defined as systolic blood pressure less than 90 mmHg.
Propensity-matched design
Patients who received TEA were matched to those who received RLB at a ratio using propensity score matching at a ratio of 1:1. This matching was used to obtain groups of patients corresponding to the 2 analgesic methods that were balanced with regard to potential confounding baseline variables. To estimate the propensity score, a multivariable logistic regression analysis was used based on the following covariates: age, sex, height, body weight, ASA class, surgical procedures, and procedural duration of surgery. We did not include the surgeon as a confounder, because the same operation team conducted all our surgeries.
Propensity matching was performed by the nearest-neighbor algorithm without replacement and patients who received TEA (TEA group) and RLB (RLB group) were matched by a 1:1 ratio. This matching was performed using a caliper width of 0.2 standard deviations of the logit of the estimated propensity score. The standardized difference was estimated to evaluate covariate balance, whereby an absolute standardized difference above 0.1 represents meaningful imbalance.
Statistical analysis
Data are expressed as median [interquartile range (IQR)] and numbers with percentage. Statistical analysis was performed using, χ2 test, Fisher’s exact test and Mann-Whitney U test. The level of significance was set at P value <0.05. Statistical analyses were performed with EZR (Saitama Medical Center, Jichi Medical University, Saitama, Japan), which is a graphical user interface for R (The R Foundation for Statistical Computing, Vienna, Austria). More precisely, it is a modified version of R commander designed to add statistical functions frequently used in biostatistics.
We calculated the 95% CI of the median differences in NRS scores using the Hodges-Lehmann estimator. We defined an acceptable non-inferiority margin as 1.0 according to the previous study (21). For the non-inferiority evaluation, we used the R statistical package (version 3.5.2, R Foundation for Statistical Computing).
Results
We reviewed electronic medical records of patients undergoing VATS under general anesthesia from February 1, 2017 to December 31, 2019. A total of 438 relevant records of patients were reviewed in this study. Of these, 344 records were excluded (Figure 1), and 94 patients were successfully matched on a 1:1 basis, based on predetermined confounders and baseline characteristics, including 47 patients in each of the RLB and the TEA groups. Table 1 summarizes patient baseline characteristics after propensity score matching. Postoperative NRS scores between the TEA group and the RLB group before propensity score matching is available in the Supplemental material (Table S1). Patients in the matched population were similar with respect to matched baseline characteristics.
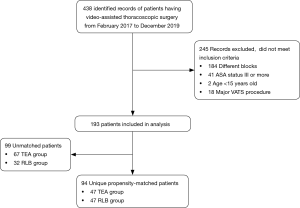
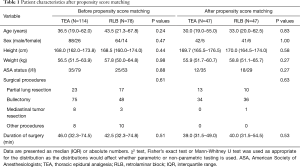
Full table
Patient demographics
There were no significant differences in the type of surgery and the duration of operation between the two groups. The duration of general anesthesia was significantly longer in the RLB group than in the TEA group [124.0 (112.5–133.0) min in the RLB group and 96.0 (85.0–111.0) min in the TEA group (P<0.01)]. Intraoperative remifentanil consumption was significantly higher in the RLB group than in the TEA group [1.3 (1.1–1.7) mg in the RLB group and 1.0 (0.7–1.3) mg in the TEA group (P<0.01)]. There were no significant differences in the intraoperative fentanyl consumption between the two groups [200.0 (100.0–250.0) µg in the RLB group and 150.0 (100.0–200.0) µg in the TEA group (P=0.13)]. There were no significant differences in the duration of postoperative thoracic drainage between the two groups [2.0 (2.0–4.0) days in the RLB group and 2.0 (2.0–3.5) days in the TEA group (P=0.345)]. There were no significant differences in the length of postoperative hospitalization between the two groups [6.0 (5.0–8.5) days in the RLB group and 5.0 (4.0–8.0) days in the TEA group (P=0.226)].
Analgesic efficacy
Postoperative NRS scores and median differences for NRS scores between the two group at rest and when coughing are shown in Table 2. There were no significant differences in the NRS scores at rest and when coughing between the two groups at 6 and 12 h after surgery, POD 1m, POD 1e and POD 2m.
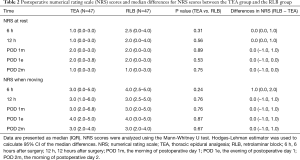
Full table
The 95% CI of the median differences in NRS scores between the two groups are shown in Figure 2. The 95% CI of the median differences in NRS scores during rest between the two groups at POD 1m were under 1, which indicates non-inferiority of RLB. The 95% CI of the median differences in NRS scores when coughing between the two groups except for 6 h after surgery were under 1. The 95% CI of the median differences in NRS scores when coughing at 6 h after surgery was over 1.

Analgesic consumption and adverse effects after surgery
There were no significant differences in the narcotic rescue usage (MME) in the operating room between the two groups [0.0 (0.0–0.0) vs. 0.0 (0.0–0.0) mg, respectively, P=0.1].
The initial infusion dose of local anesthetics was significantly higher in the RLB group than in the TEA group [10.0 (9.0–10.0) mL/h in the RLB group and 5.0 (4.0–5.0) mL/h in the TEA group (P<0.01)]. The infusion dose of local anesthetics at POD 1m were significantly higher in the RLB group than in the TEA group [8.0 (6.0–10.0) mL/h in the RLB group and 4.0 (3.0–5.0) mL/h in the TEA group (P<0.01)].
Table 3 shows frequency of rescue analgesics use and incidence of PONV after surgery in the ward. There were no significant differences in the frequency of rescue analgesics use between the two groups (P=0.87). There were no significant differences in the incidence of PONV between the two groups (P=0.17). There were no patients who developed hypotension between the two groups. There was no occurrence of pruritus or urinary retention between the two groups.
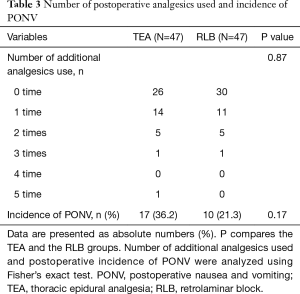
Full table
Discussion
This retrospective study compared the postoperative analgesic efficacy and adverse effects of ultrasound-guided RLB with those of TEA in patients undergoing minor VATS procedures. The analgesic effects of RLB after minor VATS procedures were similarly effective in reducing postoperative pain compared to those of TEA except for 6 h after surgery when coughing. There were no significant differences in the incidence of adverse effects or rescue doses of analgesics between the two groups. These results suggested that continuous RLB provided effective analgesia comparable to that of TEA for minor VATS procedures.
The analgesic effects of thoracic wall blocks is dependent on passive spread of local anesthetics. Local anesthetics injected into a tissue plane passively spread within the tissue plane or to adjacent tissue compartments containing nerves. Total injected mass of local anesthetics that reach and act on target nerves exert analgesia (22). Damjanovska et al. (23) reported that the spread of injectate from retrolaminar to paravertebral space is volume dependent in porcine cadaver models. Local anesthetics administered as an initial bolus dose of 20 mL, followed by continuous infusion of 8–12 mL/h in our study. Although our dosage used in this study provided effective analgesia comparable to that of TEA for minor VATS procedures, the optimal dosage of local anesthetic required for achieving effective analgesia is still not clear.
RLB are the alternatives to TEA and TPVB. Local anesthetics are injected into the fascial plane between the posterior surface of the lamina and the overlying transversospinalis muscles at 1–1.5 cm lateral to the spinous process in RLB (22). It was considered that local anesthetics penetrate the superior costotransverse ligament and spread to the thoracic paravertebral space (24-26). Sabouri et al. demonstrated that the dye injected at the retrolaminar space spread into paravertebral space, epidural space and intervertebral foramina (25). Adhikary et al. reported injectate spread to the epidural and neural foraminal spaces over 2 to 5 levels (26), and confirmed the existence of anatomical pathways for anterior spread of local anesthetics. Due to the nature of the cadaveric studies, it is hard to clarify the exact mechanisms of RLB. Furthermore, the determinant of the clinical efficacy of RLB in actual patients is still not clear.
In our study, the duration of general anesthesia was significantly longer in the RLB groups than in the TEA group. The longer duration of anesthesia was accompanied with higher intraoperative remifentanil consumption in the RLB group compared to that of TEA group. There were no significant differences in the duration of surgery. The epidural catheter was placed without sedation before induction of general anesthesia, on the other hand, RLB catheterization was performed under general anesthesia after the end of surgery. The methodological differences of catheterization might be responsible for the differences of duration of anesthesia and intraoperative remifentanil consumption between the two groups.
RLB can be performed easily and safely under ultrasound guidance. The fourth edition of the American Society of Regional Anesthesia and Pain Medicine Evidence-Based Guidelines (7) suggested that for procedures other than peri-neuraxial, deep plexus, or deep peripheral blocks, management should be based on the consideration of site compressibility, vascularity and consequence of bleeding. The RLB injects local anesthetics between the thoracic laminae and the deep paraspinous muscles. There are no major vessels at the site of injection. The site of injection of RLB are compressible because of the superficiality of the injection site and bony floor. Theoretically RLB could reduce the risk of epidural hematoma, spinal cord and nerve injury which are considered as a serious complication of TEA. RLB could also reduce the risk of pneumothorax and incidental epidural injection of local anesthetics considered as a complication of TPVB (15). RLB are considered to be safer maneuvers than TEA and TPVB.
There are several limitations in this study that include those inherent to retrospective study. First, this is a single-center study with small sample size. The population examined in this study may not be representative of the general population. Second, different individual anesthetist decided the method of analgesia. Propensity score matching analysis may have reduced the risk of bias and improved the validity of our analysis; selection bias was not eliminated. Third, we did not assess the dermatomal distribution after either block. Furthermore, different doses between the RLB and TEA may cause bias the result. Fourth, our database was insufficient to allow us to comment on outcomes such as PONV intensity. Fifth, the NRS scores were recorded by the nursing staffs, it might bias the results by which patient would overrate their pain in order to ensure rescue medications administered by the same nursing team.
However, despite the limitations, we hope to improve this observation in prospective clinical trials.
Conclusions
Our study suggested that the analgesic effects of continuous ultrasound-guided RLB were non-inferior to those of TEA for minor VATS procedures.
Acknowledgments
We appreciate the contribution of the colleagues who participated in the research.
Funding: None.
Footnote
Reporting Checklist: The authors have completed the STROBE reporting checklist. Available at http://dx.doi.org/10.21037/jtd-21-238
Data Sharing Statement: Available at http://dx.doi.org/10.21037/jtd-21-238
Peer Review File: Available at http://dx.doi.org/10.21037/jtd-21-238
Conflicts of Interest: All authors have completed the ICMJE uniform disclosure form (available at http://dx.doi.org/10.21037/jtd-21-238). The authors have no conflicts of interest to declare.
Ethical Statement: The authors are accountable for all aspects of the work in ensuring that questions related to the accuracy or integrity of any part of the work are appropriately investigated and resolved. This study was conducted in accordance with the Declaration of Helsinki (as revised in 2013). This study protocol was examined and approved by the Research Review Board at St. Mary’s Hospital on 12 January 2017 (Approval No, #16-1205). Written informed consent was obtained from all patients who participated in this study.
Open Access Statement: This is an Open Access article distributed in accordance with the Creative Commons Attribution-NonCommercial-NoDerivs 4.0 International License (CC BY-NC-ND 4.0), which permits the non-commercial replication and distribution of the article with the strict proviso that no changes or edits are made and the original work is properly cited (including links to both the formal publication through the relevant DOI and the license). See: https://creativecommons.org/licenses/by-nc-nd/4.0/.
References
- Freise H, Van Aken HK. Risks and benefits of thoracic epidural anaesthesia. Br J Anaesth 2011;107:859-68. [Crossref] [PubMed]
- Pöpping DM, Elia N, Marret E, et al. Protective effects of epidural analgesia on pulmonary complications after abdominal and thoracic surgery: A meta-analysis. Arch Surg 2008;143:990-9. [Crossref] [PubMed]
- D'Ercole F, Arora H, Kumar PA. Paravertebral Block for Thoracic Surgery. J Cardiothorac Vasc Anesth 2018;32:915-27. [Crossref] [PubMed]
- Scarfe AJ, Schuhmann-Hingel S, Duncan JK, et al. Continuous paravertebral block for post-cardiothoracic surgery analgesia: A systematic review and meta-analysis. Eur J Cardiothorac Surg 2016;50:1010-8. [Crossref] [PubMed]
- Yeung JH, Gates S, Naidu BV, et al. Paravertebral block versus thoracic epidural for patients undergoing thoracotomy. Cochrane Database Syst Rev 2016;2:CD009121 [Crossref] [PubMed]
- Davies RG, Myles PS, Graham JM. A comparison of the analgesic efficacy and side-effects of paravertebral vs epidural blockade for thoracotomy - A systematic review and meta-analysis of randomized trials. Br J Anaesth 2006;96:418-26. [Crossref] [PubMed]
- Horlocker TT, Vandermeuelen E, Kopp SL, et al. Regional Anesthesia in the Patient Receiving Antithrombotic or Thrombolytic Therapy: American Society of Regional Anesthesia and Pain Medicine Evidence-Based Guidelines (Fourth Edition). Reg Anesth Pain Med 2018;43:263-309.
- Obuchi T, Yoshida Y, Moroga T, et al. Postoperative pain in thoracic surgery: Re-evaluating the benefits of VATS when coupled with epidural analgesia. J Thorac Dis 2017;9:4347-52. [Crossref] [PubMed]
- Steinthorsdottir KJ, Wildgaard L, Hansen HJ, et al. Regional analgesia for video-assisted thoracic surgery: A systematic review. Eur J Cardiothorac Surg 2014;45:959-66. [Crossref] [PubMed]
- Sondekoppam RV, Tsui BCH. "Minimally invasive" regional anesthesia and the expanding use of interfascial plane blocks: the need for more systematic evaluation. Can J Anaesth 2019;66:855-63. [Crossref] [PubMed]
- Shanthanna H, Moisuik P, O'Hare T, et al. Survey of Postoperative Regional Analgesia for Thoracoscopic Surgeries in Canada. J Cardiothorac Vasc Anesth 2018;32:1750-5. [Crossref] [PubMed]
- von Meyenfeldt EM, Marres GMH, van Thiel E, et al. Variation in length of hospital stay after lung cancer surgery in the Netherlands. Eur J Cardiothorac Surg 2018;54:560-4. [Crossref] [PubMed]
- Voscopoulos C, Palaniappan D, Zeballos J, et al. The ultrasound-guided retrolaminar block. Can J Anaesth 2013;60:888-95. [Crossref] [PubMed]
- Zeballos JL, Voscopoulos C, Kapottos M, et al. Ultrasound-guided retrolaminar paravertebral block. Anaesthesia 2013;68:649-51. [Crossref] [PubMed]
- Onishi E, Toda N, Kameyama Y, et al. Comparison of Clinical Efficacy and Anatomical Investigation between Retrolaminar Block and Erector Spinae Plane Block. Biomed Res Int 2019;2019:2578396 [Crossref] [PubMed]
- Murouchi T, Yamakage M. Retrolaminar block: analgesic efficacy and safety evaluation. J Anesth 2016;30:1003-7. [Crossref] [PubMed]
- Onishi E, Murakami M, Nishino R, et al. Analgesic effect of double-level retrolaminar paravertebral block for breast cancer surgery in the early postoperative period: A placebo-controlled, randomized clinical trial. Tohoku J Exp Med 2018;245:179-85. [Crossref] [PubMed]
- Hwang BY, Kim E, Kwon JY, et al. The analgesic efficacy of a single injection of ultrasound-guided retrolaminar paravertebral block for breast surgery: A prospective, randomized, double-blinded study. Korean J Pain 2020;33:378-85. [Crossref] [PubMed]
- Sotome S, Sawada A, Wada A, et al. Erector spinae plane block versus retrolaminar block for postoperative analgesia after breast surgery: a randomized controlled trial. J Anesth 2021;35:27-34. [Crossref] [PubMed]
- Woodhouse A, Hobbes AFT, Mather LE, et al. A comparison of morphine, pethidine and fentanyl in the postsurgical patient-controlled analgesia environment. Pain 1996;64:115-21. [Crossref] [PubMed]
- Taketa Y, Irisawa Y, Fujitani T. Comparison of ultrasound-guided erector spinae plane block and thoracic paravertebral block for postoperative analgesia after video-assisted thoracic surgery: a randomized controlled non-inferiority clinical trial. Reg Anesth Pain Med 2019;45:10-5. [Crossref] [PubMed]
- Chin KJ. Thoracic wall blocks: From paravertebral to retrolaminar to serratus to erector spinae and back again - A review of evidence. Best Pract Res Clin Anaesthesiol 2019;33:67-77. [Crossref] [PubMed]
- Damjanovska M, Pintaric TS, Cvetko E, et al. The ultrasound-guided retrolaminar block: Volume-dependent injectate distribution. J Pain Res 2018;11:293-9. [Crossref] [PubMed]
- Costache I, Sinclair J, Farrash FA, et al. Does paravertebral block require access to the paravertebral space? Anaesthesia 2016;71:858-9. [Crossref] [PubMed]
- Sabouri AS, Crawford L, Bick SK, et al. Is a Retrolaminar Approach to the Thoracic Paravertebral Space Possible?: A Human Cadaveric Study. Reg Anesth Pain Med 2018;43:864-8. [Crossref] [PubMed]
- Adhikary SD, Bernard S, Lopez H, et al. Erector Spinae Plane Block Versus Retrolaminar Block: A Magnetic Resonance Imaging and Anatomical Study. Reg Anesth Pain Med 2018;43:756-62. [Crossref] [PubMed]