Anticancer mechanism of breviscapine in non-small cell lung cancer A549 cells acts via ROS-mediated upregulation of IGFBP4
Introduction
Worldwide, lung cancer has high morbidity and mortality (1). Almost 85% of lung cancer cases are non-small cell lung cancer (NSCLC), but because of limited early diagnosis and drug resistance to the chemotherapy of NSCLC, the overall 5-year survival rate is no more than 15% (2,3). Therefore, the development of safe and effective drugs is urgently required to treat NSCLC.
As a natural flavonoid, breviscapine (BVP) is mainly extracted from Erigeron breviscapus. Its main components include breviscapine A, breviscapine B, and caffeinylquinate esters (4). BVP displays a variety of pharmacological effects, including renoprotective, cardiovasculoprotective, neuroprotective, antiplatelet aggregation, anti-inflammatory, and anti-oxidative properties (5-8). Studies have shown that BVP has a significant anticancer effect, including against hepatocarcinoma, colorectal cancer, prostate cancer, gastric cancer, and other malignant tumors (9,10). The anticancer effect of BVP is related to its induction of apoptosis (9), the induction of DNA damage and inhibition of cell proliferation (11). Our previous study demonstrated that BVP could induce apoptosis and suppress the growth of A549 cells (adenocarcinomic human alveolar basal epithelial cells) (12), but the molecular mechanisms remain unclear.
The insulin-like growth factor binding protein family (IGFBP) comprises a group of high affinity IGF binding proteins, including six main members (IGFBP1–6), which have three structural components: N-terminus, C-terminus, and middle region (13). The IGFBP4 gene is located at 17q12-q21.1, is approximately 2246 bp in length, and encodes a protein of 237 amino acids. IGFBP4 is widely distributed in multiple organs of the body and is also secreted into the bloodstream. As a negative regulatory factor, IGFBP4 correlates negatively with tumor growth (14). It can increase the proportion of apoptotic cells in tumor tissue (15). Low expression of IGFBP4 in lung cancer tissue might underlie the proliferation and migration of lung cancer cells (16,17). In recent years, IGFBP4 has been proven to inhibit A549 cell invasion and migration in the tumor microenvironment of lung cancer (18). In addition, the expression of IGFBPs is closely related to cellular reactive oxygen species (ROS) levels. In 1999, Lang et al. confirmed that increased ROS could enhance the expression of IGFBP1 in HepG2 cells (19). Palozza et al. confirmed that lycopene promoted the expression of IGFBP3 and the apoptosis of malignant tumor cells by increasing cellular ROS levels. These observations suggested a regulatory effect between ROS and IGFBPs (20).
In the present study, we analyzed the anticancer effect of BVP on A549 cells in vitro, and investigated whether BVP could inhibit the growth, and induce apoptosis of, NSCLC by upregulating ROS and ROS-mediated upregulation of IGFBP4.
We present the following article in accordance with the MDAR reporting checklist (available at http://dx.doi.org/10.21037/jtd-21-551).
Methods
Cell lines
The Institute of Biochemistry and Cell Biology of Chinese Academy of Science (Shanghai, China) provided the A549 cells.
Drug and agents
BVP was purchased from Shanghai Ronghe Pharmaceutical Technology Development Co., Ltd. (Shanghai, China; purity ≥98%), and dissolved in dimethylsulfoxide (DMSO; the final DMSO concentration was <0.1%). Rabbit antihuman polyclonal antibodies recognizing BCL2-associated X, apoptosis regulator (BAX) and B-cell CLL/lymphoma 2 (BCL2) were bought from Abcam (Cambridge, MA, USA). The ROS scavenger N-acetylcysteine (NAC) was purchased from Selleck (Houston, TX, USA). ROS detection kit (S0033S) and Annexin V-FITC apoptosis detection kit (C1062) were bought from Beyotime. Human IGFBP-4 ELISA kit (SEKH-0215) was purchased from Solarbio.
3-(4,5-dimethylthiazol-2-yl)-2,5-diphenyltetrazolium bromide (MTT) assay
The MTT assay was used to analyze the number of viable cells. Briefly, A549 cells were seeded in the wells of 96-well microtiter plates at a density of 5×103 cells/well. The cells were treated with BVP (0, 25, 50, and 100 µM), and then the MTT solution (20 µL) was added to each well and incubation continued. The formazan generated from MTT was then dissolved in 150 µL of DMSO and the supernatant was aspirated off. The plates were then analyzed using an enzyme-linked immunosorbent assay (ELISA) plate reader at a wavelength of 490 nm.
Apoptosis assay
A549 cells were subjected to BVP pretreatment (0, 25, 50, and 100 µM) and then cultured at (5×105/well) in 6-well plates. After 48 h, the A549 cells were harvested and counted. Cells (1×105) were resuspended in 100 µL binding buffer, before 10 µL of Annexin V and 5 µL of pI were added, and incubated in the dark for 15 min at room temperature, according to the manufacturer’s instruction. The proportion of apoptotic cells was analyzed using a FACSCalibur flow cytometer (BD Biosciences, Franklin Lakes, NJ, USA).
ELISA detection of IGFBP4
A549 cells were subjected to BVP pretreatment (0, 25, 50, and 100 µM) and then cultured at (5×106/well) in 6-well plates. After 48 h, the supernatant of the cell culture medium was collected into a sterile tube and centrifuged for 20 min (2,000–3,000 r/min). Next, the supernatant was carefully collected and an IGFBP4 ELISA kit was used according to the manufacturer’s instructions, with the wavelength of the enzyme marker set to 450 nm, to measure the absorbance of each sample. The IGFBP4 relative ratio = (average absorbance of the experimental group/average absorbance of the control group).
Measurement of intracellular ROS
Intracellular ROS generation was tested by analyzing cellular hydrogen peroxide and superoxide levels using flow cytometry after staining cells with 6-carboxy-2, 7-dichlorodihydrofluorescein diacetate (DCFH-DA) according to a previous study (21). A549 cells were seeded at 1×106 cells/well in 6-well plates and cultured in the presence of BVP (0, 25, 50, and 100 µM) for 48 h. Next, the cells were washed twice and then suspended in 500 µL of 10 µM DCFH-DA for 30 min at 37 °C in the dark, followed by flow cytometry analysis. For each sample, approximately 10,000 cells were assessed. The experiment was performed in triplicate to determine the mean.
Western blotting analysis
In vitro, BVP was used to treat A549 cells in culture flasks for 48 h, after which we extracted the intracellular proteins. Western blotting was then performed according to a previously described method (22). Antibodies recognizing IGFBP4 (1:1,500 dilution; Santa Cruz Biotechnology, Santa Cruz, CA, USA), BAX (1:1,500 dilution), and BCL2 (1:1,500 dilution) were used. Data were normalized by the level of β-actin.
Quantitative real-time reverse transcription polymerase chain reaction (qRT-PCR)
After BVP treatment (0, 25, 50, and 100 µM) for 48 h, qRT-PCR was used to detect IGFBP4 mRNA expression. The Trizol assay was used to extract the total RNA from tissue and cDNA was synthesized. Next, fluorescent quantitative real-time PCR was carried out, using glutaraldehyde-3-phosphate dehydrogenase (GAPDH) as the internal reference. The primers used were as follows: IGFBP4: 5'-GGGTGTTCTCTTTGGTGTTA-3' and 5'-TGTTTTTAGGTGGCTGGATG-3'; GAPDH: 5'-CTCCTCCTGTTCGACAGTCAGC-3' and 5'-CCCAATACGACCAAATCCGTT-3'.
Adenovirus transfection
IGFBP4-vector (for overexpression) and IGFBP4-siRNA (for IGFBP4 silencing) adenovirus constructs were transfected separately into A549 cells. A549 cells overexpressing IGFBP4 and cells silenced for IGFBP4 expression were collected. The MTT assay was used to analyze cell proliferation after treatment with BVP at 100 µM for 48 h, to investigate the role of IGFBP4 on the inhibition of cell proliferation by BVP. The levels of BAX and BCL2 were detected using western blotting to study the role of IGFBP4 on apoptosis in A549 cell under BVP treatment.
Statistical analysis
All experiments were performed independently in triplicate. The results are provided as the mean ± the standard deviation (SD). For comparisons between two groups, Student’s t-test was used. For comparisons among multiple groups, analysis of variance was used. SPSS 16.0 (IBM Corp., Armonk, NY, USA) was used to perform the statistical analyses. Statistical significance was accepted at P<0.05.
Results
Effect of BVP treatment on apoptosis and proliferation of A549 cells
The results of the MTT assay were used to evaluate viable cells showed that treatment with BVP at 25, 50, and 100 µM significantly inhibited A549 cell proliferation dose-dependently (Figure 1A). The apoptosis assay showed that after treatment with BVP at 25, 50, 100 µM for 48 h, there was a dose-dependent increase in cell apoptosis, especially the rate of early apoptosis (Figure 1B,C).
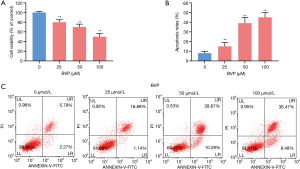
Effect of BVP treatment on IGFBP4 expression in A549 cells
The western blotting and qRT-PCR results showed that BVP treatment increased both the protein and mRNA expressions of IGFBP4 in A549 cells (Figure 2A,B,C). ELISA detection showed that treatment with BVP at 25, 50 and 100 µM significantly increased the IGFBP4 content in the culture medium (Figure 2D).
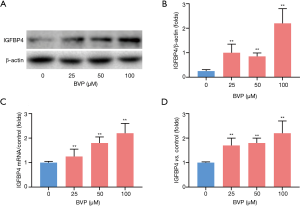
Effect of BVP treatment on the ROS content in A549 cells (Figure 3)
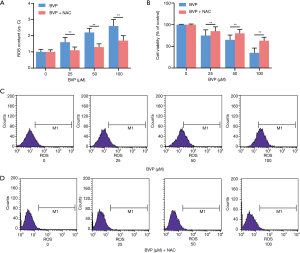
Flow cytometry analysis showed that the number of DCFH-DA-positive cells (containing intracellular ROS) increased significantly after treatment with BVP at 25, 50, and 100 µM for 48 h, which could be reversed using 1 mM NAC . The results of the MTT assay showed that BVP effectively inhibited A549 cells proliferation, which was alleviated by 1 mM NAC.
Effect of ROS on the expression of IGFBP4 in A549 cells induced by BVP
A549 cells were divided into a control group, a hydrogen peroxide group (positive control), a 100 µM BVP group, and a 100 µM BVP +1 mM NAC group. Western blotting, ELISA and qRT-PCR were used to analyze IGFBP4 expression in the cells to investigate the role of ROS in expression of IGFBP4 induced by BVP. Compared with that observed after treatment with 100 µM BVP, the expression of IGFBP4 decreased significantly in A549 cells treated with 100 µM BVP +1 mM NAC, suggesting that ROS mediated the expression of IGFBP4 in A549 cells under BVP treatment (Figure 4).
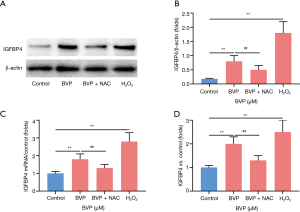
Effect of overexpression of IGFBP4 on proliferation inhibition and apoptosis promotion induced by BVP in A549 cells
The MTT cell proliferation assay results showed that the IGFBP4-vector significantly enhanced the inhibition of cell proliferation (Figure 5A). Western blotting showed that the BAX level increased, but the BCL-2 level decreased, in IGFBP4-vector cells (Figure 5B,C). After treatment with BVP at 100 µM for 48 h, compared with the IGFBP4-NC (negative control) group, the BAX level increased, while the BCL2 level decreased in IGFBP4-vector cells (Figure 5B,C).
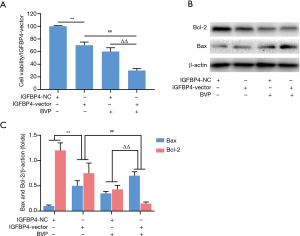
Effect of IGFBP4 silencing on proliferation inhibition and apoptosis promotion induced by BVP in A549 cells
The MTT cell proliferation assay results showed that IGFBP4-siRNA significantly alleviated the proliferation inhibition induced by BVP in A549 cells (Figure 6A). The BCL2 level increased significantly, while the BAX level decreased in IGFBP4-siRNA cells (Figure 6B,C). After 100 µM of BVP treatment for 48 h, compared with the NC-siRNA group, the BAX level decreased and the BCL2 level increased in IGFBP4-siRNA cells (Figure 6B,C).
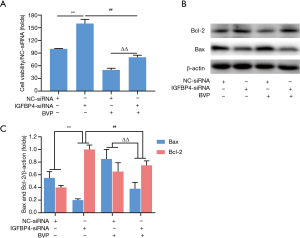
Discussion
The development of phytochemistry has led to the identification of many safe and effective plant-derived drugs, representing an alternative source of novel anticancer agents. BVP, which is mainly extracted from Erigeron breviscapus (23), has demonstrated potential anticancer properties. For instance, Wu et al. revealed that BVP could inhibit the proliferation of hepatoma cells by upregulating the expression of CYTC, BAX, and caspase 3, and downregulating the expression of BCL2 (9). BVP could also inhibit metabolic transformation during the induction of carcinogenesis by DMBA (24). In our previous study, we found that in A549 cells, BVP could induce apoptosis and suppress cell growth (12). However, because the mechanism was unclear, in the present study, we investigated the anticancer effect of BVP in NSCLC cells in vitro. The results suggested that BVP could inhibit the growth of NSCLC effectively through the promotion of apoptosis via ROS-mediated upregulation of IGFBP4.
IGFBP4 has become a research focus in studies of malignant tumors. Durai et al. found that IGFBP4 could increase the incidence of apoptosis in colorectal cancer tumor tissue, suggesting that abnormally low IGFBP4 levels might be the key reason for excessive cell proliferation (15). Damon et al. found that the formation of prostate cancer xenografts was inhibited significantly by increasing the expression of IGFBP4 (14). Ueno et al. confirmed that the proliferation and migration of renal cancer cells were enhanced significantly by IGFBP4 (25). Xiao et al. found a negative correlation between IGFBP4 and the long-term prognosis of patients with lung cancer (16). In addition, Sato et al. found that low expression of IGFBP4 is a key factor in Kas mutation-induced lung cancer (26). These studies indicated IGFBP4 as a key regulatory factor and target in NSCLC to regulate the growth, invasion, and metastasis of lung cancer, and suggested its potential research value. However, the involvement of IGFBP4 has not been reported in previous BVP-related lung cancer research. In the present study, we found that 25, 50, and 100 µM BVP could increase IGFBP4 expression. To further demonstrate that the BVP-induced inhibition of the proliferation of NSCLC is related to induction of IGFBP4 expression, we used adenovirus transfection to obtain IGFBP4 overexpressing or IGFBP4 silenced A549 cells. Western blotting and the MTT assay were used to assess the expression of apoptosis-related proteins and cell proliferation, respectively. The results confirmed that IGFBP4 overexpression significantly inhibited the proliferation of A549 cells, increased pro-apoptotic protein BAX levels, and decreased the levels of anti-apoptotic BCL2. These results indicated that IGFBP4 acts as a negative factor to inhibit NSCLC cell proliferation. Moreover, the survival of IGFBP4 overexpressing cells was significantly lower than that of control cells after BVP treatment, suggesting that IGFBP4 overexpression could increase the sensitivity of A549 cells to BVP. In contrast, compared with NC-siRNA cells, the survival of IGFBP4-silenced cells increased significantly. After BVP treatment, IGFBP4-silenced cells showed increased proliferation compared with NC-siRNA cells, indicating that changes in the IGFBP4 content in NSCLC cells are closely related to the anticancer effect of BVP.
ROS are intracellular metabolites, mainly superoxide anions (O-2), hydroxyl radicals (OH), and hydrogen peroxide (H2O2), that are key factors in the stability of the intracellular environment. As chemically active molecules, ROS have important functions in the body. They participate in various signal pathways, and a slight rise in ROS can enhance cell differentiation and proliferation. However, excessive ROS levels can result in cell lipid oxidation, DNA damage, and apoptosis (27,28). Sun et al. confirmed that apigetrin could promote apoptosis and play an anticancer role by regulating the ROS-mediated signal transducer and activator of transcription 3 (STAT3)/Janus kinase 2 (JAK2) pathway in gastric cancer cells (29). Cui et al. showed that in hepatoma cells, britannin plays an anticancer role by regulating ROS-mediated apoptosis (30). Wu and colleagues found that colon cancer cell apoptosis could be induced by ginsenoside, which could also inhibit transplanted tumor growth by activating the ROS/JNK/p53 pathway (31). In the present study, we found that 25, 50, and 100 µM of BVP increased the number of A549 DCFH-DA fluorescent cells, suggesting an increase in intracellular ROS levels. Interestingly, the ROS scavenger NAC could effectively reduce the number of A549 DCFH-DA fluorescent cells, and the MTT results showed that 1 mM NAC combined with BVP treatment significantly increased the cell survival rate compared with BVP treatment alone. This suggested that NAC could reduce the inhibitory effect of BVP on A549 cells.
Studies have confirmed that the expression levels of IGFBPs are closely related to intracellular ROS levels. Lang et al. confirmed that enhanced intracellular ROS increased the expression of IGFBP1 in liver cancer HepG2 cells (19). Palozza et al. confirmed that lycopene promotes IGFBP3 expression and the apoptosis of malignant tumor cells by increasing intracellular ROS levels (20). We speculated that the BVP-induced increase in both ROS content and IGFPB4 expression might be related. The results of western blotting, qRT-PCR, and ELISA showed that the IGFBP4 levels in both cells and the culture medium were significantly lower than those in cells treated with BVP after 48 h of cotreatment with 1 mM NAC and BVP. The abnormally increased expression of IGFBP4 in hydrogen peroxide-treated cells indicated that the BVP-induced increase in IGFBP4 expression of IGFBP4 was mediated by ROS.
In conclusion, BVP could promote the expression of IGFBP4 via upregulation of intracellular ROS levels, resulting in cell apoptosis and proliferation inhibition of NSCLC A549 cells.
Acknowledgments
Funding: The Natural Science Foundation of Zhejiang Province, China (Grant No. LQ20H310001) and Zhejiang Province Medical and health Science and Technology Platform Project of China (Grant No. 2019RC128).
Footnote
Reporting Checklist: The authors have completed the MDAR reporting checklist. Available at http://dx.doi.org/10.21037/jtd-21-551
Data Sharing Statement: Available at http://dx.doi.org/10.21037/jtd-21-551
Conflicts of Interest: All authors have completed the ICMJE uniform disclosure form (available at http://dx.doi.org/10.21037/jtd-21-551). The authors have no conflicts of interest to declare.
Ethical Statement: The authors are accountable for all aspects of the work in ensuring that questions related to the accuracy or integrity of any part of the work are appropriately investigated and resolved.
Open Access Statement: This is an Open Access article distributed in accordance with the Creative Commons Attribution-NonCommercial-NoDerivs 4.0 International License (CC BY-NC-ND 4.0), which permits the non-commercial replication and distribution of the article with the strict proviso that no changes or edits are made and the original work is properly cited (including links to both the formal publication through the relevant DOI and the license). See: https://creativecommons.org/licenses/by-nc-nd/4.0/.
References
- Siegel R, Naishadham D, Jemal A. Cancer statistics, 2012. CA Cancer J Clin 2012;62:10-29. [Crossref] [PubMed]
- Hayashi H, Okamoto I, Morita S, et al. Postprogression survival for first-line chemotherapy of patients with advanced non-small-cell lung cancer. Ann Oncol 2012;23:1537-41. [Crossref] [PubMed]
- Hotta K, Kiura K, Fujiwara Y, et al. Role of survival post-progression in phase III trials of systemic chemotherapy in advanced non-small-cell lung cancer: a systematic review. PLoS One 2011;6:e26646 [Crossref] [PubMed]
- Wang C, Li Y, Gao S, et al. Breviscapine Injection Improves the Therapeutic Effect of Western Medicine on Angina Pectoris Patients. PLoS One 2015;10:e0129969 [Crossref] [PubMed]
- Qi XM, Wu GZ, Wu YG, et al. Renoprotective effect of breviscapine through suppression of renal macrophage recruitment in streptozotocin-induced diabetic rats. Nephron Exp Nephrol 2006;104:e147-57. [Crossref] [PubMed]
- Yiming L, Wei H, Aihua L, et al. Neuroprotective effects of breviscapine against apoptosis induced by transient focal cerebral ischaemia in rats. J Pharm Pharmacol 2008;60:349-55. [Crossref] [PubMed]
- Jia JH, Chen KP, Chen SX, et al. Breviscapine, a traditional Chinese medicine, alleviates myocardial ischaemia reperfusion injury in diabetic rats. Acta Cardiologica 2008;63:757-62. [Crossref] [PubMed]
- Wang Y, Ji M, Chen L, et al. Breviscapine reduces acute lung injury induced by left heart ischemic reperfusion in rats by inhibiting the expression of ICAM-1 and IL-18. Exp Ther Med 2013;6:1322-6. [Crossref] [PubMed]
- Wu Y, Fan Q, Lu N, et al. Breviscapine-induced apoptosis of human hepatocellular carcinoma cell line HepG2 was involved in its antitumor activity. Phytother Res 2010;24:1188-94. [Crossref] [PubMed]
- Wang Q, Wang Q, Wang SF, et al. Oral Chinese herbal medicine as maintenance treatment after chemotherapy for advanced non-small-cell lung cancer: a systematic review and meta-analysis. Curr Oncol 2017;24:e269-76. [Crossref] [PubMed]
- Guan YB, Yang DR, Nong SJ, et al. Breviscapine (BVP) inhibits prostate cancer progression through damaging DNA by minichromosome maintenance protein-7 (MCM-7) modulation. Biomed Pharmacother 2017;93:103-16. [Crossref] [PubMed]
- Zeng J, Cai S. Breviscapine suppresses the growth of non-small cell lung cancer by enhancing microRNA-7 expression. J Biosci 2017;42:121-9. [Crossref] [PubMed]
- Underwood LE. Nutritional regulation of IGF-I and IGFBPs. J Pediatr Endocrinol Metab 1996;9:303-12. [PubMed]
- Damon SE, Maddison L, Ware JL, et al. Overexpression of an inhibitory insulin-like growth factor binding protein (IGFBP), IGFBP-4, delays onset of prostate tumor formation. Endocrinology 1998;139:3456-64. [Crossref] [PubMed]
- Durai R, Yang SY, Seifalian AM, et al. Role of insulin-like growth factor binding protein-4 in prevention of colon cancer. World J Surg Oncol 2007;5:128. [Crossref] [PubMed]
- Xiao Y, Zhu S, Yin W, et al. IGFBP-4 expression is adversely associated with lung cancer prognosis. Oncol Lett 2017;14:6876-80. [Crossref] [PubMed]
- Sato H, Sakaeda M, Ishii J, et al. Insulin-like growth factor binding protein-4 gene silencing in lung adenocarcinomas. Pathol Int 2011;61:19-27. [Crossref] [PubMed]
- Li W, Sun D, Lv Z, et al. Insulin-like growth factor binding protein-4 inhibits cell growth, migration and invasion, and downregulates COX-2 expression in A549 lung cancer cells. Cell Biol Int 2017;41:384-91. [Crossref] [PubMed]
- Lang CH, Nystrom GJ, Frost RA. Regulation of IGF binding protein-1 in hep G2 cells by cytokines and reactive oxygen species. Am J Physiol 1999;276:G719-27. [PubMed]
- Palozza P, Parrone N, Simone R, et al. Role of lycopene in the control of ROS-mediated cell growth: implications in cancer prevention. Curr Med Chem 2011;18:1846-60. [Crossref] [PubMed]
- Sahu RP, Zhang R, Batra S, et al. Benzyl isothiocyanate-mediated generation of reactive oxygen species causes cell cycle arrest and induces apoptosis via activation of MAPK in human pancreatic cancer cells. Carcinogenesis 2009;30:1744-53. [Crossref] [PubMed]
- Wu L, Yang W, Zhang SN, et al. Alpinetin inhibits lung cancer progression and elevates sensitization drug-resistant lung cancer cells to cis-diammined dichloridoplatium. Drug Des Devel Ther 2015;9:6119-27. [PubMed]
- Wang J, Ji SY, Liu SZ, et al. Cardioprotective effect of breviscapine: inhibition of apoptosis in H9c2 cardiomyocytes via the PI3K/Akt/eNOS pathway following simulated ischemia/reperfusion injury. Die Pharmazie 2015;70:593-7. [PubMed]
- Wei J, Xie G, Ge S, et al. Metabolic transformation of DMBA-induced carcinogenesis and inhibitory effect of salvianolic acid b and breviscapine treatment. J Proteome Res 2012;11:1302-16. [Crossref] [PubMed]
- Ueno K, Hirata H, Majid S, et al. IGFBP-4 activates the Wnt/beta-catenin signaling pathway and induces M-CAM expression in human renal cell carcinoma. Int J Cancer 2011;129:2360-9. [Crossref] [PubMed]
- Sato H, Yazawa T, Suzuki T, et al. Growth regulation via insulin-like growth factor binding protein-4 and -2 in association with mutant K-ras in lung epithelia. Am J Pathol 2006;169:1550-66. [Crossref] [PubMed]
- Boonstra J, Post JA. Molecular events associated with reactive oxygen species and cell cycle progression in mammalian cells. Gene 2004;337:1-13. [Crossref] [PubMed]
- Sharma V, Anderson D, Dhawan A. Zinc oxide nanoparticles induce oxidative DNA damage and ROS-triggered mitochondria mediated apoptosis in human liver cells (HepG2). Apoptosis 2012;17:852-70. [Crossref] [PubMed]
- Sun Q, Lu NN, Feng L. Apigetrin inhibits gastric cancer progression through inducing apoptosis and regulating 7ROS-modulated STAT3/JAK2 pathway. Biochem Biophys Res Commun 2018;498:164-70. [Crossref] [PubMed]
- Cui YQ, Liu YJ, Zhang F. The suppressive effects of Britannin (Bri) on human liver cancer through inducing apoptosis and autophagy via AMPK activation regulated by ROS. Biochem Biophys Res Commun 2018;497:916-23. [Crossref] [PubMed]
- Wu Q, Deng J, Fan D, et al. Ginsenoside Rh4 induces apoptosis and autophagic cell death through activation of the ROS/JNK/p53 pathway in colorectal cancer cells. Biochem Pharmacol 2018;148:64-74. [Crossref] [PubMed]
(English Language Editor: K. Brown)