Anticoagulation management in mechanical circulatory support
Introduction
The field of mechanical circulatory support has advanced dramatically, with an accelerated trend away from large pulsatile devices to the use of smaller continuous-flow left ventricular assist devices (CF-LVADs). However, the incidence of bleeding and thromboembolic complications in patients with mechanical circulatory support remains high. In addition, there has been an increase in serious adverse events with CF-LVADs since their approval by the US Food and Drug Administration (FDA) and subsequent commercialization. Compared with the initial experience, an increase in device thrombosis has been reported among patients who receive the HeartMate II (HMII) LVAD (Thoratec Corporation, Pleasanton, CA, USA), from an initial occurrence rate of 2-4% to 8.4% of implanted devices at 3 months (1) and 6% of implanted devices at 6 months (2). Najjar et al. (3) analyzed rates of pump thrombosis with the HeartWare Ventricular Assist Device (HVAD; HeartWare, Framingham, MA, USA) in the HeartWare bridge to transplant (BTT) trial database, reporting a rate of pump thrombosis of 8.1% of patients or 0.08 events per patient-year (EPPY), with pump thrombosis requiring exchange, in 4.2% or 0.04 EPPY. In addition, investigators recently reported that 28.7% of patients with the HeartWare HVAD experienced one or more strokes over 2 years (4). Bleeding complications are also a major cause of morbidity in patients treated with CF-LVADs and have been reported with both the HMII and the HVAD. The FDA has recently issued a safety communication regarding both devices, however the net benefits of both devices are generally accepted to outweigh the risks in the group of patients with stage D heart failure (5).
The need for anticoagulation varies widely among patients with CF-LVADs (6), and the standard “one-size-fits all” anticoagulation protocols cannot be employed. The situation is further complicated by temporal variation in coagulation status, from the immediate postoperative coagulopathy to the procoagulant environment. The need to seek a constant balance between bleeding events and thrombotic events forces us to move toward individualization of therapy.
Bleeding
When a VAD is implanted, the interaction between the device material and blood affects the delicate balance of the hematologic system, and major complications of thrombosis, and bleeding are likely related to incompatibility between the VAD surface and blood (7). Efforts to reduce pump complications are directed toward increasing hemocompatibility and maximizing blood flow without clinically significant hemolysis, areas of stasis, or turbulent or retrograde flow (7).
Bleeding complications with VADs occur at a higher rate than would be expected based solely on systemic anticoagulation. Factors implicated in an increased risk of bleeding with VADs include acquired von Willebrand syndrome, impairment in platelet aggregation, and lack of pulsatility (8,9).
von Willebrand syndrome
Evidence suggests a major role for acquired von Willebrand factor (vWF) deficiency in bleeding complications with CF-LVADs. In 1958, Heyde (10) reported a high incidence of gastrointestinal (GI) bleeding in patients with aortic stenosis (Heyde syndrome). Warkentin et al. (11) found that a severely stenotic aortic valve (AV) predisposes patients to the development of acquired von Willebrand syndrome (type 2A) characterized by the loss of large vWF multimers. This is thought to be the mechanism of high bleeding rates from GI angiodysplasia in patients with aortic stenosis. vWF abnormalities are directly related to the severity of AV disease and are improved by valve replacement (12). A loss of large, high molecular weight vWF multimers similar to that described in Heyde syndrome in patients with critical aortic stenosis has been observed in patients with implanted CF-LVADs (9,13). In a series of 101 patients, Crow and colleagues reported that GI bleeding was higher in patients with CF-LVADs than in those with pulsatile devices (14). Transfusion requirements at heart transplantation were twice as high in patients with the HMII CF-LVAD as in patients with the Heartmate XVE pulsatile device (9). All patients with HMII had reduced high molecular weight vWF multimers. Thus, this hemostatic abnormality is a contributor to excessive, typically mucosal bleeding in patients with CF-LVADs. Meyer et al. studied whether centrifugal (HVAD) versus axial (HeartMate II) may lead to differences in the von Willebrand profile (15). The degree of decrease in large molecular weight multimers of vWF was similar across both devices. However, device speed appeared to affect the vWF profile in HVAD patients, but not in patients implanted with the HeartMate II (15). Importantly, the vWF profile did not correlate with the incidence of bleeding or thromboembolic events (15). vWF multimeter deficiency is present in virtually all CF-VAD patients, but bleeding is not a universal complication, implying that there are additional factors operative in risk of bleeding (9,13,15).
The primary mechanism of acquired type 2A von Willebrand syndrome is believed to be structural changes in the shape of the vWF induced by increased shear stress, leading to exposure of the bond between amino acids 842 and 843 (9). This exposure results in proteolysis of the highest molecular weight multimers of vWF, which are the most effective in platelet-mediated hemostasis under conditions of high shear stress (16). Impaired hemostasis through the vWF-platelet pathway resulting from an acquired von Willebrand syndrome produced a bleeding diathesis in 30% to 75% of patients with CF-LVADs (9,13,17). Furthermore, shear stress potentially increases the activity and amplifies the effect of ADAMTS-13 on vWF breakdown. To investigate the mechanism of breakdown of vWF multimers, Bartoli et al. (18) created an in vitro model to mimic LVAD shear forces (18) and demonstrated both a mechanical and an enzymatic mechanism of vWF degradation during mechanical circulatory support. Under conditions of supraphysiologic shear stress, purified vWF plus recombinant ADAMTS-13 (vWF protease) produced the same profile of vWF degradation seen in patients with CF-LVAD support. Thus, these authors concluded that ADAMTS-13 is a major mechanism of vWF degradation during mechanical circulatory support and suggested it may be a therapeutic target (18).
Role of pulse pressure
The other shared characteristic present in patients with aortic stenosis and those supported by CF-LVADs is narrow pulse pressure, which has been hypothesized to dilate mucosal veins, increase smooth muscle relaxation and cause arteriovenular dilation leading to arteriovenous malformation and bleeding (19-21). Reduced pulsatility has been implicated as a significant contributor to non-surgical bleeding with continuous flow assist devices. Wever-Pinzon et al. assessed different degrees of pulsatility on the incidence of non-surgical bleeding (22). In the retrospective analysis non-surgical bleeding in the first 3 months post LVAD implantation was 4-fold higher in patients with a low pulsatility index (PI) as compared to patients with high PI (22). AV opening assessed by echocardiography during the first 3 months trended towards higher incidence of bleeding in patients whom AV was closed compared to those whom had full AV opening (22). It remains to be seen if aiming for increased pulsatility, may be a strategy to reduce non-surgical bleeding. The Momentum 3 IDE clinical study protocol (HM3tm clinical trials.gov NCT02224755) is ongoing, and is investigating the safety and efficacy of the HeartMate III. The HeartMate III is a new investigational centrifugal pump programmed with an artificial pulse, however it remains to be seen if this modification will have an impact on non-surgical bleeding.
Role of angiogenesis
Zhang et al. (23) suggested that increased angiogenesis provides a mechanistic link between LVAD support, GI angiodysplasia, and bleeding. When endothelial cells were grown with LVAD-associated vWF degradation fragments, all indices of angiogenesis were abnormal. These authors suggested that LVAD-associated vWF degradation alters angiogenesis in the bowel mucosa, which proliferates continuously, thus promoting GI angiodysplasia and predisposing LVAD patients to bleed.
Thrombosis
The spike in the incidence of pump thrombosis with the HMII was noted in early 2011. Both Starling et al. (1) and Kirklin et al. (2) reported an approximately 6-fold increase in the incidence of device thrombosis in the period from 2011 to 2012 compared with the period from 2008 to 2009. Analyses so far have not been able to identify a specific causal factor for the elevated thrombosis risk with CF-LVADs.
Compared with earlier devices, newer CF-LVADs are much smaller and designed with smaller gaps between the various components of the device. These characteristics predispose CF-LVADs to thrombosis, leading to increased hemolysis and device malfunction. However, the root cause is likely not one but multiple factors, ranging from changes in patient selection and management trends to changes in pump design and implantation techniques (Figure 1) (24). A clinical trial investigating this issue is currently underway (Prevention of HMII Pump Thrombosis through Clinical Management, ClinicalTrials.gov NCT02158403).
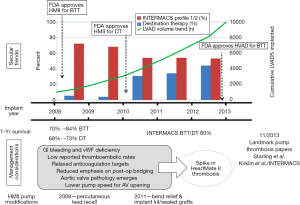
Risk factors for bleeding and thrombotic events
Boyle et al. (25) published a retrospective analysis of 956 HMII LVAD patients, investigating preoperative risk factors for hemorrhagic and thrombotic events during LVAD support in outpatients. The risk of bleeding, hemorrhagic stroke, ischemic stroke, and pump thrombosis differed by patient demographics, including sex, age, body mass index (BMI), history of diabetes, and etiology of heart failure (Figure 2) (25). The investigators hoped to identify high-risk patients and potentially develop individualized anticoagulation and anti-platelet strategies. Female sex was identified as a risk factor for post discharge bleeding, pump thrombosis, and hemorrhagic and ischemic stroke. When stratified by age, younger women were at higher risk for hemorrhagic stroke and older women at higher risk for ischemic stroke. Although retrospective, this analysis comprised the largest population to date and provided some insight regarding perioperative risk factors.
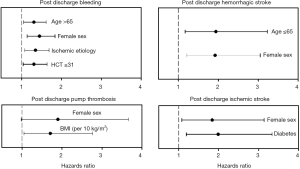
Goldstein et al. (26) described thrombosis risk factors related to the pump itself (i.e., heat, shearing forces, and malposition), to the patient (i.e., atrial fibrillation, preexistent thrombus, and hypercoagulable state), and to management (i.e., suboptimal anticoagulation). Ballew et al. (27) investigated survival in patients supported with the HMII LVAD in relation to treatment and lactate dehydrogenase (LDH) levels. Patients with LDH levels greater than 1,000 U/L treated medically with anticoagulants other than argatroban had a mortality of 50% compared with 100% survival in patients who had surgical intervention.
In the HeartWare BTT LVAD trial, Najjar et al. (3) found the following risk factors for device thrombosis: subtherapeutic INR, suboptimal anticoagulation and antiplatelet therapy, and elevated blood pressure (3).
Although risk scoring to predict risk of bleeding is not widely used, Koene et al. applied the HAS-BLED and CHA2DS2-VASc scores retrospectively to a cohort of patients implanted with HMII, and found that scores ≥3 conferred higher risks of bleeding and thrombosis, respectively (28).
Anticoagulation
Multiple factors drive anticoagulation practices, including device type, pump flow, coagulation measurements used, and institutional experience. Tailoring anticoagulant to individual needs is at the forefront in therapeutic decisions.
For example, consideration may need to be given to the relationship between the manifestation of thrombosis and the type of thrombus (29). Acute catastrophic pump thromboses are generally caused by red thrombi, which consist mainly of red blood cells trapped in a fibrin mesh, whereas more slowly developing subacute thrombi are more likely to be due to white thrombi, which are composed of aggregated platelets along with debris. Because red thrombi are produced by coagulation of stagnant blood, thrombolytic treatment may be most successful, while white thrombi, which are formed by activation of platelets due to shear stress, may respond better to glycoprotein (GP) IIb/IIIa inhibitors.
In the setting of contemporary risk of early device thrombosis, bridging protocols during the perioperative period and the way we monitor anticoagulation may need revision. The ongoing PREVENT trial standardized the bridging protocol in the perioperative period, and we await interim results. The recommended practices are focused on implantation technique, anticoagulation regimen, pump speed, and blood pressure management.
The clinical problem is complicated by uncertainty regarding which therapeutic targets to use to measure the multifaceted components of the coagulation cascade in patients. Individual variability in responsiveness to acetylsalicylic acid (ASA), anticoagulant drug metabolism, circulating levels of coagulation drug metabolism, circulating levels of coagulation factors, platelet count, fibrinolytic activity, and etiology of cardiac disease all affect coagulation status and response to anticoagulants and antiplatelet agents. This variation predicts a failure of one-size-fits-all anticoagulation strategies and drives the need for further research.
Management of bleeding
A recently published 1-year analysis of the US arm of the international multicenter TRACE study (Study of Reduced Anticoagulation/Anti-platelet Therapy in Patients with the HMII LVADs) analyzed the effects of reducing antithrombotic therapy in patients with the HMII LVAD (30). Pharmacotherapy at initiation included warfarin only (38%), aspirin only (28%), or no antithrombotic therapy (34%). The percentage of patients free from ischemic stroke at 1 year was 93.8±2.5%, and the percentage free from device thrombosis was 92.7±2.7%. Despite reduction in antithrombotic therapy, 52% of patients had a recurrent bleeding event (30). These interim results from the US arm of this study suggest that reducing antithrombotic therapy on a chronic basis to manage select patients with recurrent nonsurgical bleeding may be safe but bleeding events continue to occur. Furthermore, although reduction of antithrombotic therapy did not increase ischemic stroke, the results cannot support an empiric strategy of reduced antithrombotic therapy for all patients. Finally, identification of patients who could potentially tolerate reduced anticoagulation was only done in reaction to recurrent bleeding, highlighting the challenge of prospectively predicting who is at high risk of hemorrhagic complications after CF-VAD.
Management of thrombosis
In 2013, Goldstein et al. (26) proposed an algorithm for the diagnosis and management pump thrombosis according to clinical presentation, suggesting consideration of intravenous heparin in the setting of hemolysis. However, medical management for pump thrombosis is associated with a high proportion of treatment failures, recurrent admission for hemolysis, and ultimately the need for pump exchange. Starling et al. (1) reported a mortality rate of 50% in the 38 patients (implanted with HMII) in their study who were treated medically.
In the HeartWare BTT and subsequent Continued Access Protocol (CAP) trials (3), one year survival was lower in patients with a thrombosis event vs. none (69.4% vs. 85.4%, P=0.21). Medical therapy was attempted first in 30 of 34 events and failed in 15 patients, of whom 12 required pump exchange (8 survived and 4 expired). Medical therapy was not standardized and left to the discretion of the individual centers, consisted of IV heparin, GP 2b/3a antagonists (such as eptifibatide), and tPA (3). Eptifibatide alone or in combination with heparin succeeded in 50% (3 of 6), heparin alone was never successful (0 of 5), and tPA was successful in treating pump thrombosis in 12 out of 19 patients (63%).
Intravenous unfractionated heparin (UFH)
Intravenous UFH has been the mainstay therapy for bridging patients and for initial management of suspected device thrombosis. However, there is a paucity of data defining the optimal method for monitoring UFH. In a prospective observational study, Adatya et al. (31) reported a high incidence of discordance between activated partial thromboplastin time (aPTT) and anti-factor Xa (anti-FXa). Anti-FXa is a more direct measure of UFH activity, as it measures the inhibition of a single enzyme: the ability of heparin-antithrombin complex to inhibit activated coagulation factor X (Figure 3) (32). In contrast, aPTT reflects the intrinsic coagulation and final common pathway and can be affected by warfarin administration, lupus anticoagulant, liver disease, and factor deficiencies (32). In patients with an INR ≥1.5 and in patients admitted with suspected device obstruction, discordant rates were high, and the most common observation was a supratherapeutic aPTT value with a therapeutic anti-FXa level (31). Despite supratherapeutic aPTT levels, major bleeding events were low. Hemolysis and warfarin administration may falsely elevate aPTT, resulting in overestimation of heparin concentration and under anticoagulation (31). This has major implications, particularly in the medical management of device thrombosis.
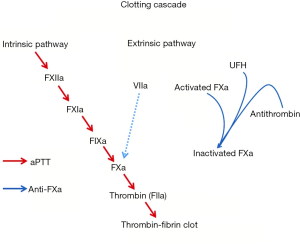
Low molecular weight heparin (LMWH)
Because of the increased risk of early device thrombosis, use of unopposed vitamin K antagonists should be avoided, and when surgical bleeding risk does not prevent their use, intravenous UFH or LMWH should be used for bridging. Low doses to bridge patients may alleviate the procoagulant state, which can occur with the initiation of warfarin therapy. Sandner et al. (33) administered UFH <24 hours after LVAD with enoxaparin or dalteparin, targeting anti-FXa levels of 0.2 to 0.4 IU/mL in the early postoperative period to bridge patients to a therapeutic INR of 2-2.5. This strategy was demonstrated to be safe with a low bleeding risk.
GP 11b/IIIa inhibitors for VAD thrombosis
Small case series have suggested the use of GP inhibitor in addition to or as an alternate to an IV anticoagulant for suspected device obstruction (34-37). Tellor et al. (37) reported on a retrospective series of 17 patients (16 implanted with HMII) receiving 22 separate administration of eptifibatide. More than 60% of patients received concomitant heparin therapy, eptifibatide dose ranges from 0.1 to 2 µg/kg/min but was associated with excessive bleeding events including four hemorrhagic strokes (37). The occurrence of major bleeding events suggests careful screening of selected patients before the use of eptifibatide. The conclusion was the risk of medical therapy with eptifibatide to treat suspected pump obstruction outweighed the benefit and device exchange should be the treatment of choice (37).
Direct thrombin inhibitors
There is limited data for the use of direct thrombin inhibitors such as argatroban and bivalirudin asides from retrospective case series. Unique pharmacologic profile to interact with both free and clot-bound thrombin, argatroban reversibly inhibits thrombin by inhibiting thrombin-and activation of coagulation factors V, VIII and XIII and protein C (38). It has a short half-life; however, in the event of bleeding there is no reversal agent and when using in conjunction with warfarin there is wide variation in INR (38). Badiye et al. (39) reported on a small case series where three out of four patients were treated medically with argatroban for hemolysis and after follow up of 13 months three patients were free of hemolysis. In comparison with bivalirudin, argatroban is hepatically cleared, and higher concentration of bivalirudin is required to inhibit clot-bound thrombin in contrast to free thrombin, hence requiring greater doses with greater risk of bleeding (39). Dabigatran and rivaroxaban are both orally administered. Currently we do not have any data in mechanical circulatory support and their use is currently not routinely advocated.
Thrombolytics
The decision to use thrombolytics in the management of device thrombosis is device specific and needs to be critically assessed on a case to case basis. Schlendorf et al. (40) described a series of eight HMII patients who presented with intravascular hemolysis secondary to pump thrombosis and were treated with intraventricular thrombolytic therapy. Three patients had complete and lasting resolution of hemolysis, but in the remaining five patients, thrombolytic therapy ultimately failed to halt or reverse pump thrombosis and hemolysis. Prasad et al. (41) performed pathologic analysis on six explanted HMII devices examining the elbow, inlet bearings, inlet stator, blood tube/rotor inlet, outlet elbow, and outlet stator. Rotor assembly clot was found to be concentric chronic layering as was found in the stator, suggesting rotor assembly clot may be an extension of the process on the stator bearing rather than acute thrombus. An older more and more organized thrombus becomes less likely to dissolve with tPA.
Treatment of thrombus events with tPA in the HeartWare BTT and subsequent CAP trials reported an overall success rate with tPA of 63%. Eight patients received triple therapy consisting of tPA, heparin, and eptifibatide; one died, therapy failed in four (required device exchange) and the thrombus event resolved in three (3). When tPA was used alone or with dual therapy (heparin or eptifibatide) it successfully resolved the thrombus in 9 of 11 patients (3).
Patients not candidates for device exchange with refractory hemolysis may be considered for tPA when no other option is available understanding the high risk for hemorrhagic complications. Future studies to characterize who would benefit from a trial of medical therapy first, which agent, preferred anticoagulation monitoring protocol and duration vs. those who should proceed directly to exchange are needed to recommend one strategy over another.
Special situations
Erythropoiesis-stimulating agents
Nassif et al. (42) retrospectively reviewed clinical outcomes with use of erythropoiesis -stimulating agents (ESA) in patients with HMII LVADs. Anemic LVAD patients have low circulating erythropoietin levels, and treatment of LVAD patients with ESA has been proposed (42,43). ESA use had higher rates of suspected pump thrombosis, with a hazard ratio of 2.35. For every 100-unit increase in cumulative ESA dosage, the hazard of suspected pump thrombosis increased by 10%. Furthermore, ESA use was associated with a significantly higher rate of all-cause mortality. This study was limited by its retrospective nature and need for inverse probability-weighted analysis to mitigate selection bias. However, the association of ESA use and thrombosis has been observed in other populations and given this data ESA should be used with caution.
Acute/subacute heparin induced thrombocytopenia (HIT) before mechanical circulatory support
There is little data regarding the management of acute/subacute HIT prior to mechanical circulatory support, with no generally accepted guidelines. Cardiopulmonary bypass requires full anticoagulation, and the use of heparin in the setting of acute HIT (thrombocytopenia and positive Hep/PF4 antibody by enzyme-linked immunosorbent assay) is concerning because of the risk of thrombosis due to HIT (T), a prothrombotic state caused by antibodies against heparin/platelet factor 4 complex. According to published guidelines of the American College of Physicians (ACP), heparin remains the recommended anticoagulant during cardiopulmonary bypass, even in patients with history of HIT if antibodies are no longer detectable, a brief re-challenge with heparin may be tolerable in conjunction with strict avoidance of heparin in the postoperative setting (44). However, the choice of anticoagulant during cardiopulmonary bypass in patients with acute HIT (thrombocytopenia with positive HIT antibody) is not as well defined. Several strategies may be considered: delaying surgery until HIT antibodies are negative; intra-operative anticoagulation with direct thrombin inhibitor such as argatroban or bivalirudin (irreversibility makes these agents less appealing); UFH plus an intravenous anti-platelet (short-acting antiplatelet therapy potentially attenuates HIT antibody-induced platelet activation), and plasma exchange to remove the HIT antibody (45-49). More data are required in this area in order to recommend one strategy over another, and this condition remains a significant management challenge in the perioperative period.
Conclusions
In patients with end stage heart failure refractory to medical therapy, CF-LVADs not only improve mortality, but also confer improvements in quality of life, functional capacity and symptoms. The mechanism for the major complications thrombosis and bleeding appears related to the effect of blood interaction with the VAD surface. Further optimizing surface coating and design may reduce these complications in newer generation devices.
Acknowledgements
None.
Footnote
Conflicts of Interest: The authors have no conflicts of interest to declare.
References
- Starling RC, Moazami N, Silvestry SC, et al. Unexpected abrupt increase in left ventricular assist device thrombosis. N Engl J Med 2014;370:33-40. [PubMed]
- Kirklin JK, Naftel DC, Kormos RL, et al. Interagency Registry for Mechanically Assisted Circulatory Support (INTERMACS) analysis of pump thrombosis in the HeartMate II left ventricular assist device. J Heart Lung Transplant 2014;33:12-22. [PubMed]
- Najjar SS, Slaughter MS, Pagani FD, et al. An analysis of pump thrombus events in patients in the HeartWare ADVANCE bridge to transplant and continued access protocol trial. J Heart Lung Transplant 2014;33:23-34. [PubMed]
- Pagani FD, Milano CA, Tatooles AJ, et al. HeartWare HVAD for the Treatment of Patients With Advanced Heart Failure Ineligible for Cardiac Transplantation: Results of the ENDURANCE Destination Therapy Trial. ISHLT 35th Annual Meeting. Nice, France, 2015.
- Food and Drug Administration. Serious Adverse Events with Implantable Left Ventricular Assist Devices (LVADs): FDA Safety Communication. Available online: http://www.fda.gov/MedicalDevices/Safety/AlertsandNotices/ucm457327.htm
- Görlinger K, Bergmann L, Dirkmann D. Coagulation management in patients undergoing mechanical circulatory support. Best Pract Res Clin Anaesthesiol 2012;26:179-98. [PubMed]
- Eckman PM, John R. Bleeding and thrombosis in patients with continuous-flow ventricular assist devices. Circulation 2012;125:3038-47. [PubMed]
- Klovaite J, Gustafsson F, Mortensen SA, et al. Severely impaired von Willebrand factor-dependent platelet aggregation in patients with a continuous-flow left ventricular assist device (HeartMate II). J Am Coll Cardiol 2009;53:2162-7. [PubMed]
- Uriel N, Pak SW, Jorde UP, et al. Acquired von Willebrand syndrome after continuous-flow mechanical device support contributes to a high prevalence of bleeding during long-term support and at the time of transplantation. J Am Coll Cardiol 2010;56:1207-13. [PubMed]
- Heyde EC. Gastrointestinal bleeding in aortic stenosis. N Engl J Med 1958;259:196.
- Warkentin TE, Moore JC, Morgan DG. Aortic stenosis and bleeding gastrointestinal angiodysplasia: is acquired von Willebrand's disease the link? Lancet 1992;340:35-7. [PubMed]
- Vincentelli A, Susen S, Le Tourneau T, et al. Acquired von Willebrand syndrome in aortic stenosis. N Engl J Med 2003;349:343-9. [PubMed]
- Crow S, Chen D, Milano C, et al. Acquired von Willebrand syndrome in continuous-flow ventricular assist device recipients. Ann Thorac Surg 2010;90:1263-9; discussion 1269. [PubMed]
- Crow S, John R, Boyle A, et al. Gastrointestinal bleeding rates in recipients of nonpulsatile and pulsatile left ventricular assist devices. J Thorac Cardiovasc Surg 2009;137:208-15. [PubMed]
- Meyer AL, Malehsa D, Budde U, et al. Acquired von Willebrand syndrome in patients with a centrifugal or axial continuous flow left ventricular assist device. JACC Heart Fail 2014;2:141-5. [PubMed]
- Moake JL, Turner NA, Stathopoulos NA, et al. Involvement of large plasma von Willebrand factor (vWF) multimers and unusually large vWF forms derived from endothelial cells in shear stress-induced platelet aggregation. J Clin Invest 1986;78:1456-61. [PubMed]
- Geisen U, Heilmann C, Beyersdorf F, et al. Non-surgical bleeding in patients with ventricular assist devices could be explained by acquired von Willebrand disease. Eur J Cardiothorac Surg 2008;33:679-84. [PubMed]
- Bartoli CR, Restle DJ, Zhang DM, et al. Pathologic von Willebrand factor degradation with a left ventricular assist device occurs via two distinct mechanisms: mechanical demolition and enzymatic cleavage. J Thorac Cardiovasc Surg 2015;149:281-9. [PubMed]
- Cappell MS, Lebwohl O. Cessation of recurrent bleeding from gastrointestinal angiodysplasias after aortic valve replacement. Ann Intern Med 1986;105:54-7. [PubMed]
- Letsou GV, Shah N, Gregoric ID, et al. Gastrointestinal bleeding from arteriovenous malformations in patients supported by the Jarvik 2000 axial-flow left ventricular assist device. J Heart Lung Transplant 2005;24:105-9. [PubMed]
- Slaughter MS. Hematologic effects of continuous flow left ventricular assist devices. J Cardiovasc Transl Res 2010;3:618-24. [PubMed]
- Wever-Pinzon O, Selzman CH, Drakos SG, et al. Pulsatility and the risk of nonsurgical bleeding in patients supported with the continuous-flow left ventricular assist device HeartMate II. Circ Heart Fail 2013;6:517-26. [PubMed]
- Zhang DM, Kang J, Restle DJ, et al. LVAD-Associated von Willebrand Factor Degradation Alters Angiogenesis: A Mechanistic Link Between LVAD Support, Gastrointestinal Angiodysplasia, and Bleeding? The Journal of Heart and Lung Transplantation 2015;34:S13.
- Mehra MR, Stewart GC, Uber PA. The vexing problem of thrombosis in long-term mechanical circulatory support. J Heart Lung Transplant 2014;33:1-11. [PubMed]
- Boyle AJ, Jorde UP, Sun B, et al. Pre-operative risk factors of bleeding and stroke during left ventricular assist device support: an analysis of more than 900 HeartMate II outpatients. J Am Coll Cardiol 2014;63:880-8. [PubMed]
- Goldstein DJ, John R, Salerno C, et al. Algorithm for the diagnosis and management of suspected pump thrombus. J Heart Lung Transplant 2013;32:667-70. [PubMed]
- Ballew CC, Benton EM, Groves DS, et al. Comparing Survival of HMII Patients with Elevated LDH: Implications for Medical and Surgical Management. The Journal of Heart and Lung Transplantation 2013;32:S38.
- Koene RJ, Win S, Naksuk N, et al. HAS-BLED and CHA2DS2-VASc scores as predictors of bleeding and thrombotic risk after continuous-flow ventricular assist device implantation. J Card Fail 2014;20:800-7. [PubMed]
- Blitz A. Pump thrombosis-A riddle wrapped in a mystery inside an enigma. Ann Cardiothorac Surg 2014;3:450-71. [PubMed]
- Katz JN, Adamson RM, John R, et al. Safety of reduced anti-thrombotic strategies in HeartMate II patients: A one-year analysis of the US-TRACE Study. J Heart Lung Transplant 2015;34:1542-8. [PubMed]
- Adatya S, Uriel N, Yarmohammadi H, et al. Anti-factor Xa and activated partial thromboplastin time measurements for heparin monitoring in mechanical circulatory support. JACC Heart Fail 2015;3:314-22. [PubMed]
- Adatya S, John R, Eckman PM. Treatment of hemolysis with intravenous unfractionated heparin using an antifactor Xa-based monitoring protocol. J Thorac Cardiovasc Surg 2015;149:1465-6. [PubMed]
- Sandner SE, Riebandt J, Haberl T, et al. Low-molecular-weight heparin for anti-coagulation after left ventricular assist device implantation. J Heart Lung Transplant 2014;33:88-93. [PubMed]
- Feldman D, Pamboukian SV, Teuteberg JJ, et al. The 2013 International Society for Heart and Lung Transplantation Guidelines for mechanical circulatory support: executive summary. J Heart Lung Transplant 2013;32:157-87. [PubMed]
- Blais DM, Sun B, Vesco P, et al. Profound thrombocytopenia with glycoprotein IIb/IIIa inhibitors plus heparin for pump thrombus. J Heart Lung Transplant 2008;27:1361-2. [PubMed]
- Al-Quthami AH, Jumean M, Kociol R, et al. Eptifibatide for the treatment of HeartMate II left ventricular assist device thrombosis. Circ Heart Fail 2012;5:e68-70. [PubMed]
- Tellor BR, Smith JR, Prasad SM, et al. The use of eptifibatide for suspected pump thrombus or thrombosis in patients with left ventricular assist devices. J Heart Lung Transplant 2014;33:94-101. [PubMed]
- Swan SK, Hursting MJ. The pharmacokinetics and pharmacodynamics of argatroban: effects of age, gender, and hepatic or renal dysfunction. Pharmacotherapy 2000;20:318-29. [PubMed]
- Badiye A, Hernandez GA, Chaparro S. Argatroban as novel therapy for suspected thrombosis in patients with continuous-flow left ventricle assist device and hemolysis. ASAIO J 2014;60:361-5. [PubMed]
- Schlendorf K, Patel CB, Gehrig T, et al. Thrombolytic therapy for thrombosis of continuous flow ventricular assist devices. J Card Fail 2014;20:91-7. [PubMed]
- Prasad SM, Robertson JO, Itoh A, et al. Histologic analysis of clots in explanted axial continuous-flow left ventricular assist devices. J Heart Lung Transplant 2015;34:616-8. [PubMed]
- Nassif ME, Patel JS, Shuster JE, et al. Clinical outcomes with use of erythropoiesis stimulating agents in patients with the HeartMate II left ventricular assist device. JACC Heart Fail 2015;3:146-53. [PubMed]
- Vrtovec B, Radovancevic R, Delgado RM, et al. Significance of anaemia in patients with advanced heart failure receiving long-term mechanical circulatory support. Eur J Heart Fail 2009;11:1000-4. [PubMed]
- Warkentin TE, Greinacher A, Koster A, et al. Treatment and prevention of heparin-induced thrombocytopenia: American College of Chest Physicians Evidence-Based Clinical Practice Guidelines (8th Edition). Chest 2008;133:340S-380S.
- Zucker MJ, Sabnani I, Baran DA, et al. Cardiac transplantation and/or mechanical circulatory support device placement using heparin anti-coagulation in the presence of acute heparin-induced thrombocytopenia. J Heart Lung Transplant 2010;29:53-60. [PubMed]
- Pötzsch B, Klövekorn WP, Madlener K. Use of heparin during cardiopulmonary bypass in patients with a history of heparin-induced thrombocytopenia. N Engl J Med 2000;343:515. [PubMed]
- Koster A, Loebe M, Mertzlufft F, et al. Cardiopulmonary bypass in a patient with heparin-induced thrombocytopenia II and impaired renal function using heparin and the platelet GP IIb/IIIa inhibitor tirofiban as anticoagulant. Ann Thorac Surg 2000;70:2160-1. [PubMed]
- Dyke CM, Smedira NG, Koster A, et al. A comparison of bivalirudin to heparin with protamine reversal in patients undergoing cardiac surgery with cardiopulmonary bypass: the EVOLUTION-ON study. J Thorac Cardiovasc Surg 2006;131:533-9. [PubMed]
- Welsby IJ, Um J, Milano CA, et al. Plasmapheresis and heparin reexposure as a management strategy for cardiac surgical patients with heparin-induced thrombocytopenia. Anesth Analg 2010;110:30-5. [PubMed]