Navigational transbronchial needle aspiration, percutaneous needle aspiration and its future
Introduction
The increasing use of chest computerized tomography (CT) scans for respiratory disorders and for lung cancer screening in high-risk patients following the results from the National Lung Screening Trial (NLST) has resulted in the evaluation of pulmonary nodules as a common occurrence for many practitioners (1). The annual number of CT scans currently performed in the United States is approximately 85 million (2). From the NLST we know that 27% of patients had a clinically significant nodule found on low dose CT scan and that screening following their protocol led to a 20% relative risk reduction in lung cancer deaths. However, of the nodules found, more than 90% underwent a diagnostic evaluation proving that 96.4% of those positive screenings were, in actuality, false positives (1). Given the current smoking estimates of the US population and the NLST pulmonary nodule incidence, as many as 1–2 million more suspicious nodules will be discovered annually with lung cancer screening. Lung cancer screening programs are being implemented at many institutions in an attempt to identify, counsel and formulate a plan for evaluation of these nodules in identified high-risk individuals. Peripheral lung nodule evaluation represents a clinical challenge. Given that the vast majority of these nodules are not malignant the goal is to find an accurate, safe and minimally-invasive diagnostic modality. Minimally invasive diagnostic procedures include bronchoscopic interventions and non-bronchoscopic techniques such as transthoracic needle aspiration (TTNA) or surgical biopsy. Unfortunately, conventional bronchoscopic techniques provide a diagnostic yield of 18–62% when sampling peripheral pulmonary nodules (3). In recent years advances in technology have lead to the introduction of electromagnetic navigational bronchoscopy (ENB) as a tool to guide sampling of peripheral lung nodules. The same principle has also recently been expanded and applied to the transthoracic needle biopsy, referred to as electromagnetic transthoracic needle aspiration (E-TTNA). The 2013 3rd Edition ACCP Guidelines for the Diagnosis and Management of Lung Cancer state that “in patients with peripheral lung lesions difficult to reach with conventional bronchoscopy, ENB is recommended if the equipment and the expertise are available (Grade 1C)” (4). In this review, we will highlight the current state of navigational transbronchial needle aspiration (TBNA) and percutaneous needle aspiration (PCNA) and speculate on future directions.
Technology
Electromagnetic (EM) tracking for guidance in medical procedures is based on four basic principles (5):
- Creating a magnetic field around the patient;
- Utilizing a sensing device to detect location and orientation within the magnetic field;
- Reformatting data from a CT scan for 3-D reconstruction that will overlap the location and orientation within the magnetic field;
- Display of a computer interface throughout the procedure to guide the clinician.
In detail, there is a field generator to form a low-strength and varying EM field that will pass through a patient’s body. This field then generates current in sensor coils in or on instruments that will be used through the working channel of the bronchoscope in order to determine their x, y and z positions as well as their orientation and rotation around their own axis when the instrument is moved within the EM field. There are several different sensors; the two that are used in medical applications are the 5 and 6 degree-of-freedom sensors. The 5 degree-of-freedom sensor measures the x, y and z positions as well as the pointing direction. The 6 degree-of-freedom adds rotation around its own axis (roll, pitch, and yaw movements) (6). Once the capability to track positioning within the airway is achieved, a map is required to guide the proceduralist to the lesion of interest.
CT scans contain a set of 2-dimensional images formed by pixels that are various shades on a grey-scale, based on their density, and this is measured in Hounsfield Units. Based on these density differences, the various structures of the body are easily defined and delineated from one another. As pixels form images to represent a “slice” of the body with a certain thickness, the pixels contain averaged information with depth. Pixels can therefore be used together to form a 3-dimensional cube called a voxel. These voxels can then be reconstructed to highlight various body structures such as the airways, lymphnodes, lungs, vasculature or tumors that can be used to create several visualizations for display and analysis. Once a 3-dimensional CT scan image is created, it can then be segmented, meaning, certain specific structures can be extracted from the CT images. This technique is used to convert digital imaging and communications in medicine standards (DICOM) images from a CT scan into a structural map of the airways. ENB requires a high-definition of the airways and so thin-section, high-resolution CT scan images are required with a minimum slice thickness of 1.25 mm.
Putting these items together, commercial systems use a sensor probe on their instruments that picks up the EM field generated by the localization system which is comprised of a processor, amplifier and either a location board or a sensor pad and array. When the sensor is placed within the EM field, its position and orientation are displayed on a monitor with the location superimposed upon previously acquired thin-section high-resolution CT images reconstructed into a 3-dimensional airway map. This image-guided localization device has several uses including bronchoscopic guidance for transbronchial biopsies and needle aspirations, fiducial placement and marking of nodules prior to surgery as well as PCNA.
Equipment
In the United States, there are two EM navigational systems available for clinical use; superDimension (Covidien, Minneapolis, MN, USA, Figure 1) and SPiNView (Veran Medical Technologies, St. Louis, MO, USA, Figure 2). Both systems are based on the same fundamental concept. A CT scan using a dedicated protocol is obtained for planning ENB targets and to overlay the magnetic field to the CT scan and the patient anatomy. The superDimension system (Covidien, Minneapolis, MN, USA) consists of several components. The main processor unit, steerable extended working channel catheter with different tip angulations ranging from 45o to 180o, a locatable guide and the EM board which generates the EM field and lies underneath the patient throughout the procedure. During the pre-procedure planning phase, the target lesion is identified using proprietary software (Figure 3, Figure 4A).
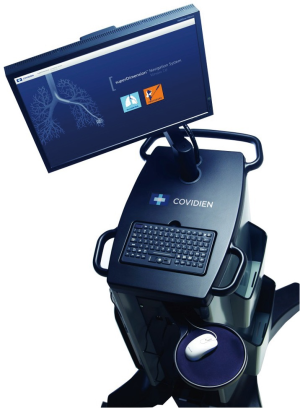
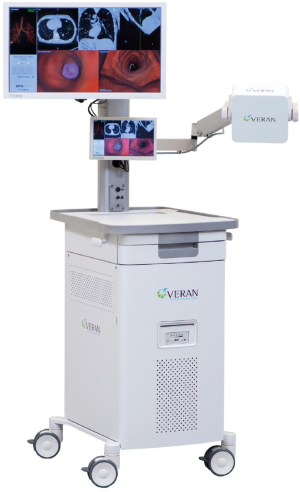
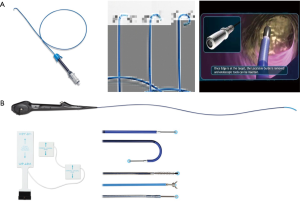
The SPiNView system (Veran Medical Technologies, St. Louis, MO, USA) uses a tracking pad that serves as a reference point, providing feedback information for the system to maintain registration despite the change in location of the target lesion due to respiratory variation. Ideally, to maximize accuracy, CT scan images are obtained during inspiration and expiration for the computer algorithm to compensate for the dynamic respiratory changes. As with the superDimension system, SpinDrive requires a pre-procedure planning phase that is performed using proprietary software loaded onto a dedicated laptop (Figure 3). The main piece of equipment is composed of a central unit with a generator that creates the EM field which projects down, over the patient. Another major difference is in the biopsy instruments. They are always-on tip trackable, eliminating the need for an extended working channel, but limiting the directionality. Because the instruments are tip-tracked, the need for fluoroscopy to ensure appropriate positioning is obviated. The biopsy forceps and brushes are 1.8 mm in outer diameter (OD) and histology and cytology needles are available in 19 and 21 gauge (G) respectively (Figure 4B).
TTNA is a non-bronchoscopic diagnostic approach to lung nodules and masses. TTNA of a peripheral lung lesion can be performed under ultrasonography, fluoroscopy or CT guidance.
Recently, the EM navigational technology was incorporated into the traditional TTNA technique creating a novel diagnostic tool and potentially a new paradigm in the evaluation of lung nodules. The SPiNPerc system (Veran Medical Technologies, St. Louis, MO, USA) consists of the same computer unit used with the SPiNView system for ENB, but instead of endobronchial navigation via a trackable bronchoscopic instrument, a 19 G (105 mm length) needle is use for navigation. The needle has two components, a stylet that works as a sensor allowing EM navigation to peripheral pulmonary nodules and the needle itself. Once within the target lesion the needle works as a working channel through which smaller FNA needles can be passed or core biopsies can be obtained (Figure 5) (7). Currently, this approach is only available on the Veran SPiNView System.
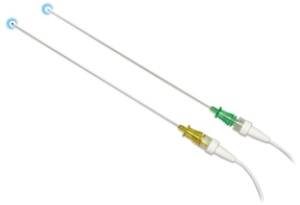
Technique/protocol
Planning
Prior to starting the procedure, the digitized information from each patient’s CT scan is imported into the EM navigation system where all views (axial, coronal and sagittal) of the chest are reconstructed using a proprietary software that is unique to each system. Virtual endoscopy images of the airway are reconstructed. Consequently, anatomic landmarks (typically major bronchial tree bifurcations) are identified as coordinates on the corresponding CT as well as on the virtual bronchoscopy image.
Registration
The same identifiable landmarks are then used during real-time bronchoscopy in order to better correlate the CT data and reconstruction to the actual anatomy. With the superDimension system, when these points in the airway are reached, either the sensor can be used to physically touch the airway landmark or a foot-pedal can be depressed to identify the areas that were used in the pre-procedural planning. With the SPiNView, the system automatically registers the patient’s anatomy without the need for any manual registration.
ENB with superDimension procedural phase starts by placing the patient on a non-metallic bed equipped with anchors for the navigational board. Once connected with the main station a rectangular EM field is displayed and three sensors are attached to the patient’s chest in a triangular distribution. Registration is confirmed and the operator navigates to the target after which the steerable and locatable guide is removed while the extended working channel is left in place to act as a guide sheath and the biopsies are taken using conventional instruments. The SPiNView system has automatic registration, but requires attaching the sensor pads before obtaining the CT images. Once in the procedural room the patient is positioned in a similar fashion used to acquire the CT scan and the bronchoscopic procedure can commence.
Bronchoscopic procedure
The bronchoscopist navigates to the target lesion steering the bronchoscope with the locatable guide/extended working channel or alternatively using tip-tracked instruments. The system will help to navigate the operator into the correct airway using virtual images and reconstructions of the CT scans. Once in proximity to the target lesion, biopsies, needle aspirates and brushings are obtained.
Percutaneous biopsy
The EM navigational needle is also tip-tracked in real time to the target lesion. Pre-procedural planning is performed as described above. The needle trajectory is guided by multiplanar CT scan images and the virtual 3D reconstruction images. Once in the lesion the needle stylet (sensor) is removed. Fine needle aspiration is performed for rapid on-site evaluation (ROSE) followed by larger biopsies. Multiple core samples are obtained using a 20 G automatic biopsy needle (Figure 6). Specimens are typically placed in formaldehyde for pathological analysis. Post procedure evaluation with chest ultrasonography or conventional chest radiography is advised in order to exclude complications such as pneumothorax.
Diagnostic yield
Multiple factors influence the diagnostic accuracy including location, nodule size, and presence in cross-section of a bronchus leading to the nodule (8). Size has the most studied influence on diagnostic yield and despite some conflicting data, the majority of papers report a sensitivity that is lower for lesions less than 2 cm in size (9-11). Another factor that was recently reported to decrease diagnostic yield is target movement during the different phases of the respiratory cycle. The dynamic nature of the procedure ideally would require a continuous and strict registration to maximize accuracy and prevent sample errors. Chen et al. reported an average motion of 17.6 mm for lung lesions when comparing scans during inspiration and expiration. Lesions found to be most susceptible were those in the lower lobes. Interestingly, size and distance from the pleura did not significantly affect respiratory-induced movement of pulmonary nodules (12). Moderate sedation vs. general anesthesia selection is an area of much controversy during bronchoscopic procedures. In a recent study no difference was found in diagnostic yield for ENB guided biopsies of peripheral lung nodules though the procedure time was less in the moderate sedation group (13).
ENB is also noted to have a steep learning curve and Lamprecht et al. noted an increased diagnostic yield after some procedural experience; with diagnostic yield of 80% and 87.5% for the first 30 and last 30 procedures, respectively (14).
Gex et al. performed a systematic review and meta-analysis on diagnostic yield and safety of ENB whereby they reported on six variables associated with higher ENB yields: nodule location in the upper or middle lobes, nodule size, lower registration error, presence of a bronchus sign on CT imaging, combined use of an ultrasonic radial probe and catheter suctioning as a sampling technique (15). Zhang et al. in a recent systematic review evaluating ENB showed a pooled sensitivity, specificity, positive likelihood ratio, negative likelihood ratio and diagnostic odds ratio of 82%, 100%, 19.36%, 0.23%, and 97.62%, respectively. This group re-confirmed the above six variables associated with improved diagnostic yield for ENB (16).
The use of TTNA is mainly based on location of the lesion, availability of less-invasive modalities and proceduralist expertise. Althogh the diagnotic yield varies depending on the technology used, Rivera et al. reported that the pooled sensitivity of TTNA for the diagnosis of peripheral bronchogenic carcinoma was 0.90 [95% confidence interval (CI), 0.88–0.91] and when separated by imaging modality, 92% (95% CI, 90–94%) and 88% (95% CI, 85–90%) for studies of CT scan-guided and fluoroscopy-guided TTNA, respectively (17). No clinical trials exist at this time to determine the diagnostic yield for E-TTNA.
Safety
One of the major advantages of bronchoscopic navigational biopsies is the safety profile. ENB complications include pneumothorax, bleeding and respiratory failure. In general ENB is a low risk diagnostic modality (16). Gex et al. reported a pneumothorax of 3.1% of whom 1.6% of patients required further management with a chest tube drainage (15).
Complications related to TTNA include pneumothorax, bleeding, hemoptysis, air embolism and infection. In a study by Wiener et al. that included 15,865 patients, pneumothorax risk after TTNA was 15% (95% CI, 14–16%) with 7% (95% CI, 6–7.2%) requiring management with a chest tube (18). Parenchymal hemorrhage and hemoptysis have an incidence of 11% and 7%, respectively. These bleeding complications tend to be self-limited and rarely require active intervention or management (19,20). E-TTNA is no different than other transthoracic biopsies with other imaging modalities and therefore the complication rate is presumed to be similar, with recent studies pending publication.
Combining modalities
ENB and EBUS
Traditional TBNA and EBUS-TBNA have both been shown to be effective in the diagnosis and staging of lung cancer (21-23). Recent technologies like ENB and radial EBUS have been successfully introduced to the diagnostic armamentarium of peripheral lung nodules. The clinical utility of the combination of different diagnostic techniques have been evaluated by different groups. Utilization of the radial EBUS in combination to ENB provides a real time verification of the target, theoretically reducing the possibility of sample error. Eberhardt et al. reported a diagnostic yield of 88% for the combined procedure versus 69% and 59% for radial EBUS and ENB alone respectively (24). Other hybrid methods include virtual bronchoscopy and radial EBUS. The clinical benefit of this combination has been documented (25). ENB was conceived with the intention to localize and sample lesions that are difficult to access by conventional bronchoscopic techniques. Chee et al demonstrated that ENB improves localization rates after unsuccessful attempts with radial EBUS (93% vs. 73%), however diagnostic yield for radial EBUS alone and radial EBUS with ENB were 43% and 50%, respectively (26). Development of instruments that allows ENB with real time EBUS and biopsy capabilities could dramatically contribute to the evaluation of peripheral pulmonary nodules.
ENB and EBUS and E-TTNA
Combination of EBUS-TBNA, ENB and E-TTNA provides a comprehensive, minimally-invasive approach to the diagnosis and staging of peripheral pulmonary lesions. This complement of a bronchoscopic with a non-bronchoscopic biopsy technique consolidates multiple procedures that can be performed in a single setting. A potential algorithm to maximize outcomes would include multiple modalities and ROSE. The procedure would start with convex-probe EBUS mediastinal staging, followed by ENB and biopsy of the peripheral pulmonary lesion which can be confirmed with radial EBUS, followed by E-TTNA of the peripheral nodule if a diagnosis is not confirmed using the bronchoscopic techniques with ROSE. The advantages of this approach may be an increase in overall diagnostic yield as well as a cost effective approach to the diagnosis of lung nodules in high risk patients for malignancy. The patient has fewer hospital encounters, more efficient time to diagnosis, less anesthesia and lower cost. Preliminary reports from a recent study by Yarmus et al. showed a diagnostic yield higher than 80% for peripheral lesions ranging in size from 12–29 mm. Before widespread use of this suggested protocol, further clinical studies are required.
Other uses
Guidance for lymph node biopsy
A less common use of EM bronchoscopy is guidance to sample mediastinal and hilar lymph nodes as an alternative to EBUS. The planning and registration is performed as described above, with one of the lymph nodes marked as a target. In this way, the location and distance to the lymph node are determined in real time using EM guidance. The site for transtracheal or transbronchial puncture is then marked by creating an indentation of the airway mucosa with the sensor probe at the desired location. Following marking, standard instruments can be utilized to perform traditional TBNA (27). In a recent study by Diken et al., they reported a diagnostic yield for ENB-TBNA of 72.8% in nodes with a mean size of 17.56 ± 6.25 mm in a cohort of 94 patients with 145 lymph nodes biopsied as compared to 42.2% using conventional TBNA (28). Another study by Gildea et al. evaluated diagnostic yield and safety with ENB to sample peripheral lesions (n=54) and lymph nodes (n=31). With respect to lymphadenopathy, their diagnostic yield was 100% for nodes with a mean size of 28.13 (range, 11–72) mm and there were no complications noted with lymph node sampling (29).
Dye marking prior to surgery
Several techniques exist to localize pulmonary nodules for operative excision and they typically require a percutaneous approach to place pleural markers, coils, hook wires or radioactive markers but these procedures, unfortunately, have a high rate of pneumothorax. The premise for use of ENB for localization is that after the lesion is located during navigation and methylene blue is injected near the lesion. The surgeon resects the marked area during the same operative and anesthesia time, and intraoperative frozen sectioning confirms successful resection. This approach greatly aids lung preservation, length of procedural, anesthesia and operating room times, limits cost and makes the work-up and management for a pulmonary nodule efficient for the patient, all while minimizing morbidity (30). Krimsky et al. have recently published an approach whereby they “tented” the sub-pleural space adjacent to the nodule with the locatable guide and extended working channel under fluoroscopy and a 25 gauge sclerotherapy needle was used to inject 0.5 mL of either indigo carmine or methylene blue dye at the site. Following marking, the patients were taken to the operating room for a minimally-invasive resection either by video-assisted thoracoscopic surgery (VATS) or robotic-assisted thoracoscopic surgery (RATS) approaches with or without an anatomic resection and lymph node dissection. They found the dye marker outlined the area of interest 81% of the time in 22 patients. One patient had diffuse dye marking of the parietal pleura and two patients the dye was not visualized. The nodules studied had a mean size of 13.4 mm (range, 7–30 mm). No patient had to be converted to an open procedure (31).
A similar technique was published the same year by Bolton et al. They evaluated 19 patients who underwent ENB localization and dye marking prior to robotic resection. Their approach differed in that they marked the lesion as well as the nearest pleural surface. Mean size of lesions was 18 mm (range, 8–40 mm and only four patients with lesions >20 mm, all of which were ground glass nodules/masses) (32). Finally, earlier this year, Anayama et al. published a technique for the use of thoracoscopy following injection of indocyanine green (ICG) that is delivered bronchoscopically via ENB guidance to a pulmonary nodule without injuring the visceral pleura. They have tested this protocol on three live pigs whereby they created pulmonary nodules with 0.3 mL of 5% agar containing iopamidol. ENB was used to then bronchoscopically inject 0.1 mL of ICG to the area of the nodule. After 6 hours, the lung was examined with a thoracoscope that has near infrared capabilities. The nodules were successfully located and were found to be within 3.3±0.95 mm of the nodule. The benefit of this approach is that ICG is not affected by color changes of the pleura that can occur with anthracosis or underlying pulmonary disease. Contrary to the use of fiducial markers, micro-coils or hook-wires this approach did not result in pneumothorax. ICG can also notably be confirmed with CT scan prior to surgery (33).
Fiducial placement
Probably the most common therapeutic rather than diagnostic use for ENB is for the placement of fiducials. Fiducial markers are radiographically visible markers that identify the position of a tumor and assists with accommodation for respiratory motion during stereotactic body radiation therapy (SBRT). Fiducials can be inserted via three methods: transthoracic, intravascular and bronchoscopic. Bronchoscopic insertion has a better safety profile over the other two insertion methods with less risk of pneumothorax, pleurisy and pulmonary infarcts (34-36). Ideally, fiducials must have a minimum spatial separation of 20 mm and an angular separation between any groupings of three fiducials of 15 degrees (37). Given this standard, it is advisable to place more than the three required fiducials in case there is migration or expectoration of the fiducial, a known complication of bronchoscopic fiducial placement that occurs 10–26% of the time (36).
Kupelian et al. placed metallic markers for radiation therapy in 23 patients for early-stage lung cancer either with CT guidance or using the ENB system transbronchially. They reported a pneumothorax rate of 8/15 (53.3%) vs. 0/8 (0%) in each group, respectively. Successful placement and migration rates were equivalent (38). Anantham et al. reported on the attempted placement of fiducial markers in nine patients. The overall success rate was 89% and of those with successful placement, 90% of the fiducials remained in appropriate position without migration 7–10 days later when they returned for SBRT planning. A mean number of 4.9 fiducial markers were placed per patient (39). Finally, Harley et al. report on 43 consecutive patients who had 2–5 fiducial markers placed in or around peripheral lung lesions using EBUS. EBUS was combined with ENB when the lesion was difficult to navigate to. In total, 12 patients required navigation with ENB. Thirty of forty-three patients had no evidence of fiducial migration following fiducial placement. Of the 13 patients who had 1 or more fiducials that had migrated, none had any clinical impact on treatment with SBRT. One patient had a small pneumothorax necessitating chest tube placement and 1-day admission and several patients had blood-streaked sputum, but otherwise there were no complications reported (40).
Foreign body removal
Although rare in adults, there is a case report of a foreign body (copper wire) that had been aspirated by a 46-year-old gentleman and had migrated to the right lower lobe causing a chronic cough. Bronchoscopic attempts were unsuccessful as the wire was not visible despite using a pediatric and ultrathin bronchoscope. ENB was then performed and forceps were extended through a guide sheath to successfully remove the wire with resolution of the cough. Utilization of ENB saved this patient from undergoing surgical intervention and can be considered in similar cases (41).
Placement of brachytherapy catheter
There is a technical note published in German describing the successful placement of a brachytherapy catheter after navigation to a peripheral, unresectable, lung cancer in the right upper lobe, confirmed with radial EBUS. This catheter remained in appropriate position for five therapeutic sessions without migration and the patient had an excellent clinical outcome. This technique may become useful in patients who are not surgical candidates, particularly with upper lobe lesions where fiducial markers are less stable (42). Future ENB therapeutic interventions like placement of ablative catheters may be a potential treatment alternative to surgery or SBRT.
Cost effectiveness
As mentioned earlier, the number of incidentally found pulmonary nodules is increasing with high-risk patients being screened for lung cancer and the use of CT scans for thoracic symptoms and disorders. The probability of malignancy, however, in an abnormal CT scan depends upon the lesion and patient characteristics. The probability of malignancy of a pulmonary nodule can be estimated using existing models (43,44). If the probability of malignancy is high the patient should go directly to resection. Equally, if the likelihood of malignancy is low, then the patient will undergo radiographic follow-up. The management of a solitary pulmonary nodule of intermediate risk for malignancy (between 5% and 65%) is unclear. In a time where healthcare is required to become more fiscally responsible, cost must be a consideration when selecting a diagnostic or therapeutic modality.
With respect to ENB with or without E-TTNA, although costs are significant, particularly if an additional high-resolution, thin-slice CT scan must be obtained, they are not prohibitive. American insurance companies do currently cover ENB. There are a couple of publications that can help to determine the most cost-effective strategy for diagnosis of a pulmonary nodule. Unfortunately, E-TTNA is a novel modality and has not yet been extensively studied and so is not included in the papers below, though may show an improvement in cost-effectiveness by increasing diagnostic yield in a minimally-invasive and time-efficient way.
The first study is a cost-effectiveness evaluation that was performed looking at initial diagnostic strategies for evaluation of a pulmonary nodule using a decision-analysis model to assess costs and outcomes of PET/CT (watchful waiting) vs. CT-guided TTNA vs. ENB vs. VATS. The base case consists of a 1.5–2 cm, upper lobe, incidentally discovered pulmonary nodule in a patient with a 15 pack-year smoking history and either a spiculated appearance or growth of 15% of this nodule on serial imaging. The above scenario with both a 50% and a 65% pretest probability of cancer were evaluated (45). Diagnostic modalities were compared based on published literature estimates of sensitivity, specificity, complication and outcome probabilities, 5-year survival rates and cost. The study found that when cancer prevalence was 65% ENB and CT-guided TTNA were the most cost-effective strategies. The decision to use one strategy over the other should be individualized based on size and location (including central vs. peripheral and proximity to airways) of the pulmonary nodule. When cancer prevalence was 50%, ENB, CT-guided TTNA and PET/CT had equivalent cost-effectiveness.
Another study by Dale et al. was a cost-consequence analysis of ENB vs. CT-guided TTNA for a solitary pulmonary nodule (46). The base case is a 65-year-old with >40 pack-year smoking history and a 2-cm solitary pulmonary nodule. The base case would have an approximate 60% chance of malignancy. They found that using CT-guided TTNA or ENB followed by VATS for a non-diagnostic biopsy was less cost-effective than performing serial biopsies. Serial biopsies meant that patients in the ENB arm who had a non-diagnostic ENB biopsy all went onto CT-guided TTNA. Similarly, patients in the CT-guided TTNA arm with a non-diagnostic CT-guided TTNA all went on to ENB biopsy. The serial biopsy approach decreased cost in both arms as the need for VATS decreased, though complications did increase. The cost savings of CT-guided TTNA are largely driven by the high sensitivity thereby leading to less need for VATS to secure a diagnosis. Although ENB is more expensive than CT-guided TTNA overall, there are cost savings with the use of ENB secondary to the minimal complication rate of ENB vs. CT-guided TTNA (pnemothorax: 1.6% vs. 15%, hemorrhage: 0.1% vs. 1% and respiratory failure: 0.1% vs. 1%, respectively).
Limitations
The main ENB limitation continues to be related to the alignment of the EM tracker to pre-procedural CT images. The current findings suggest that the diagnostic yield of ENB may be affected by CT-to-body divergence rather than the size or location of the lesion. CT-to-body divergence is unavoidable as ENB is not a real-time navigational system and this may be one of its current drawbacks. Although ENB is often referred to as the “global positioning systems (GPS) of the lungs” there is a significant difference. GPS devices receive constant positioning feedback regarding their location on a map that is continuously updated; ENB systems do not update the anatomy from the planning CT scan.
These differences could be obviated in the future with the application of CT with ultra-fast temporal resolution and/or respiratory gating.
Other technologies like co-registration and image fusion technology have been used for diagnostic and therapeutic interventions in solid organs and tumors. Application of such modalities in pulmonary procedures would be an alternative to overcome some of the ENB limitations. ENB is a multi-step process that is time consuming and requires a dedicated infrastructure but there is no doubt of the utility of ENB in the diagnosis and management of pulmonary nodules.
Future directions
ENB and E-TTNA will continue to strive towards an effective, efficient, minimally invasive and cost-conscious approach to the diagnosis of pulmonary nodules in addition to playing a role in some therapeutic interventions such as fiducial placement, pleural dye marking and foreign body removal. With ENB improving localization of pulmonary nodules hopefully some localized therapy can be delivered to the nodules (47). Tsushima et al. demonstrated the feasibility of fiberoptic bronchoscopy-guided radiofrequency-induced tissue ablation using normal sheep lungs. In this study, coagulation and necrotic tissue formed around the electrode tip created increased tissue impedance and therefore adequate necrosis was not achieved (48).
A later study by Tanabe et al. used a cooled electrode probe in humans and achieved an ablative area with destruction of the alveolar space and coagulation necrosis to a maximal area of 12×10 mm2 (49). More study needs to be performed before this should be used as a treatment option.
In order to move in a forward direction, we need to continue to improve upon the diagnostic yield as this modality offers several advantages over other techniques such as CT-guided TTNA and VATS including a better safety profile, particularly for patients who have significant respiratory compromise and cost savings.
Acknowledgements
None.
Footnote
Conflicts of Interest: No conflict of interest for Dr. Argento and Dr. Arias. Dr. Yarmus has received educational grants, research grants and consulting fees from Veran.
References
- National Lung Screening Trial Research Team, Aberle DR, Adams AM, et al. Reduced lung-cancer mortality with low-dose computed tomographic screening. N Engl J Med 2011;365:395-409. [PubMed]
- Goodman DM. Initiatives focus on limiting radiation exposure to patients during CT scans. JAMA 2013;309:647-8. [PubMed]
- Baaklini WA, Reinoso MA, Gorin AB, et al. Diagnostic yield of fiberoptic bronchoscopy in evaluating solitary pulmonary nodules. Chest 2000;117:1049-54. [PubMed]
- Rivera MP, Mehta AC, Wahidi MM. Establishing the diagnosis of lung cancer: Diagnosis and management of lung cancer, 3rd ed: American College of Chest Physicians evidence-based clinical practice guidelines. Chest 2013;143:e142S-65S.
- Gilbert C, Akulian J, Ortiz R, et al. Novel bronchoscopic strategies for the diagnosis of peripheral lung lesions: present techniques and future directions. Respirology 2014;19:636-44. [PubMed]
- Reynisson PJ, Leira HO, Hernes TN, et al. Navigated bronchoscopy: a technical review. J Bronchology Interv Pulmonol 2014;21:242-64. [PubMed]
- Arias S, Lee H, Semaan R, et al. Use of Electromagnetic Navigational Transthoracic Needle Aspiration (E-TTNA) for Sampling of Lung Nodules. J Vis Exp 2015.e52723. [PubMed]
- Seijo LM, de Torres JP, Lozano MD, et al. Diagnostic yield of electromagnetic navigation bronchoscopy is highly dependent on the presence of a Bronchus sign on CT imaging: results from a prospective study. Chest 2010;138:1316-21. [PubMed]
- Wallace JM, Deutsch AL. Flexible fiberoptic bronchoscopy and percutaneous needle lung aspiration for evaluating the solitary pulmonary nodule. Chest 1982;81:665-71. [PubMed]
- Fletcher EC, Levin DC. Flexible fiberoptic bronchoscopy and fluoroscopically guided transbronchial biopsy in the management of solitary pulmonary nodules. West J Med 1982;136:477-83. [PubMed]
- Chechani V. Bronchoscopic diagnosis of solitary pulmonary nodules and lung masses in the absence of endobronchial abnormality. Chest 1996;109:620-5. [PubMed]
- Chen A, Pastis N, Furukawa B, et al. The effect of respiratory motion on pulmonary nodule location during electromagnetic navigation bronchoscopy. Chest 2015;147:1275-81. [PubMed]
- Bowling MR, Kohan MW, Walker P, et al. The effect of general anesthesia versus intravenous sedation on diagnostic yield and success in electromagnetic navigation bronchoscopy. J Bronchology Interv Pulmonol 2015;22:5-13. [PubMed]
- Lamprecht B, Porsch P, Wegleitner B, et al. Electromagnetic navigation bronchoscopy (ENB): Increasing diagnostic yield. Respir Med 2012;106:710-5. [PubMed]
- Gex G, Pralong JA, Combescure C, et al. Diagnostic yield and safety of electromagnetic navigation bronchoscopy for lung nodules: a systematic review and meta-analysis. Respiration 2014;87:165-76. [PubMed]
- Zhang W, Chen S, Dong X, et al. Meta-analysis of the diagnostic yield and safety of electromagnetic navigation bronchoscopy for lung nodules. J Thorac Dis 2015;7:799-809. [PubMed]
- Rivera MP, Mehta AC; American College of Chest Physicians. Initial diagnosis of lung cancer: ACCP evidence-based clinical practice guidelines (2nd edition). Chest 2007;132:131S-148S.
- Wiener RS, Schwartz LM, Woloshin S, et al. Population-based risk for complications after transthoracic needle lung biopsy of a pulmonary nodule: an analysis of discharge records. Ann Intern Med 2011;155:137-44. [PubMed]
- vanSonnenberg E, Casola G, Ho M, et al. Difficult thoracic lesions: CT-guided biopsy experience in 150 cases. Radiology 1988;167:457-61. [PubMed]
- Berquist TH, Bailey PB, Cortese DA, et al. Transthoracic needle biopsy: accuracy and complications in relation to location and type of lesion. Mayo Clin Proc 1980;55:475-81. [PubMed]
- Dasgupta A, Mehta AC. Transbronchial needle aspiration. An underused diagnostic technique. Clin Chest Med 1999;20:39-51. [PubMed]
- Wang KP, Marsh BR, Summer WR, et al. Transbronchial needle aspiration for diagnosis of lung cancer. Chest 1981;80:48-50. [PubMed]
- Wang KP, Terry PB. Transbronchial needle aspiration in the diagnosis and staging of bronchogenic carcinoma. Am Rev Respir Dis 1983;127:344-7. [PubMed]
- Eberhardt R, Anantham D, Ernst A, et al. Multimodality bronchoscopic diagnosis of peripheral lung lesions: a randomized controlled trial. Am J Respir Crit Care Med 2007;176:36-41. [PubMed]
- Asahina H, Yamazaki K, Onodera Y, et al. Transbronchial biopsy using endobronchial ultrasonography with a guide sheath and virtual bronchoscopic navigation. Chest 2005;128:1761-5. [PubMed]
- Chee A, Stather DR, Maceachern P, et al. Diagnostic utility of peripheral endobronchial ultrasound with electromagnetic navigation bronchoscopy in peripheral lung nodules. Respirology 2013;18:784-9. [PubMed]
- Weiser TS, Hyman K, Yun J, et al. Electromagnetic navigational bronchoscopy: a surgeon’s perspective. Ann Thorac Surg 2008;85:S797-801. [PubMed]
- Diken ÖE, Karnak D, Çiledağ A, et al. Electromagnetic navigation-guided TBNA vs conventional TBNA in the diagnosis of mediastinal lymphadenopathy. Clin Respir J 2015;9:214-20. [PubMed]
- Gildea TR, Mazzone PJ, Karnak D, et al. Electromagnetic navigation diagnostic bronchoscopy: a prospective study. Am J Respir Crit Care Med 2006;174:982-9. [PubMed]
- Port J, Harrison S. Electromagnetic navigational bronchoscopy. Semin Intervent Radiol 2013;30:128-32. [PubMed]
- Krimsky WS, Minnich DJ, Cattaneo SM, et al. Thoracoscopic detection of occult indeterminate pulmonary nodules using bronchoscopic pleural dye marking. J Community Hosp Intern Med Perspect 2014.4. [PubMed]
- Bolton WD, Howe H 3rd, Stephenson JE. The utility of electromagnetic navigational bronchoscopy as a localization tool for robotic resection of small pulmonary nodules. Ann Thorac Surg 2014;98:471-5; discussion 475-6. [PubMed]
- Anayama T, Qiu J, Chan H, et al. Localization of pulmonary nodules using navigation bronchoscope and a near-infrared fluorescence thoracoscope. Ann Thorac Surg 2015;99:224-30. [PubMed]
- Nuyttens JJ, Prévost JB, Praag J, et al. Lung tumor tracking during stereotactic radiotherapy treatment with the CyberKnife: Marker placement and early results. Acta Oncol 2006;45:961-5. [PubMed]
- Harada T, Shirato H, Ogura S, et al. Real-time tumor-tracking radiation therapy for lung carcinoma by the aid of insertion of a gold marker using bronchofiberscopy. Cancer 2002;95:1720-7. [PubMed]
- Reichner C, Collins B, Gagnon G, et al. The Placement of Gold Fiducials for CyberKnife Stereotactic Radiosurgery Using a Modified Transbronchial Needle Aspiration Technique. J Bronchology 2005;12:193-5.
- Cyberknife System: patient preparation: user's manuel (Cyberknife G4). Sunnyvale, CA Accuracy Incorporated 2005;63:1442-7.
- Kupelian PA, Forbes A, Willoughby TR, et al. Implantation and stability of metallic fiducials within pulmonary lesions. Int J Radiat Oncol Biol Phys 2007;69:777-85. [PubMed]
- Anantham D, Feller-Kopman D, Shanmugham LN, et al. Electromagnetic navigation bronchoscopy-guided fiducial placement for robotic stereotactic radiosurgery of lung tumors: a feasibility study. Chest 2007;132:930-5. [PubMed]
- Harley DP, Krimsky WS, Sarkar S, et al. Fiducial marker placement using endobronchial ultrasound and navigational bronchoscopy for stereotactic radiosurgery: an alternative strategy. Ann Thorac Surg 2010;89:368-73; discussion 373-4. [PubMed]
- Karpman C, Midthun DE, Mullon JJ. A distal airway foreign body removed with electromagnetic navigation bronchoscopy. J Bronchology Interv Pulmonol 2014;21:170-2. [PubMed]
- Harms W, Krempien R, Grehn C, et al. Electromagnetically navigated brachytherapy as a new treatment option for peripheral pulmonary tumors. Strahlenther Onkol 2006;182:108-11. [PubMed]
- Swensen SJ, Silverstein MD, Ilstrup DM, et al. The probability of malignancy in solitary pulmonary nodules. Application to small radiologically indeterminate nodules. Arch Intern Med 1997;157:849-55. [PubMed]
- Ost DE, Gould MK. Decision making in patients with pulmonary nodules. Am J Respir Crit Care Med 2012;185:363-72. [PubMed]
- Deppen SA, Davis WT, Green EA, et al. Cost-effectiveness of initial diagnostic strategies for pulmonary nodules presenting to thoracic surgeons. Ann Thorac Surg 2014;98:1214-22. [PubMed]
- Dale CR, Madtes DK, Fan VS, et al. Navigational bronchoscopy with biopsy versus computed tomography-guided biopsy for the diagnosis of a solitary pulmonary nodule: a cost-consequences analysis. J Bronchology Interv Pulmonol 2012;19:294-303. [PubMed]
- Eberhardt R, Kahn N, Herth FJ. ‘Heat and destroy’: bronchoscopic-guided therapy of peripheral lung lesions. Respiration 2010;79:265-73. [PubMed]
- Tsushima K, Koizumi T, Tanabe T, et al. Bronchoscopy-guided radiofrequency ablation as a potential novel therapeutic tool. Eur Respir J 2007;29:1193-200. [PubMed]
- Tanabe T, Koizumi T, Tsushima K, et al. Comparative study of three different catheters for CT imaging-bronchoscopy-guided radiofrequency ablation as a potential and novel interventional therapy for lung cancer. Chest 2010;137:890-7. [PubMed]