Local injection of botulinum toxin type A (BTX-A) prevents scarring esophageal stricture caused by electrocautery in rabbit models
Introduction
Benign esophageal strictures are clinically common and can be caused by a variety of disorders including chronic acid reflux, chemical burns from corrosive substances, radiation injury, and post-surgical and post-endoscopic complications. These injuries can cause local submucosal fibrous connective tissue proliferation and collagen deposition, thus leading to stricture formation (1,2). In the 1990s, the most common type of esophageal stricture was cicatricial stricture resulting from long-standing gastroesophageal reflux disease (GERD), accounting for approximately 60–75% of all benign esophageal stricture cases (3,4). With the advances in gastrointestinal endoscopy, endoscopic radiofrequency ablation, endoscopic mucosal resection (EMR), endoscopic submucosal dissection (ESD), and other techniques are widely used for the treatment of precancerous esophageal lesions (e.g., Barrett’s esophagus) and early esophageal cancer, with a consequent increase in benign esophageal strictures after endoscopic treatment, especially after ESD of large esophageal lesions. Previous studies showed that the probabilities of esophageal stricture after EMR and ESD were 6% and 11–20%, respectively (5-7). Circumferential mucosal defects of more than three-fourths of the esophageal circumference are an independent predictor of post-ESD strictures of the esophagus (5,6). The incidence of esophageal strictures after ESD in these patients has been reported to be 66–100% (8,9). Dysphagia is the main symptom of benign esophageal stricture and can be accompanied by varying degrees of vomiting, aspiration, weight loss, and even cachexia, which can seriously affect patients' health and even threaten their lives. After the occurrence of esophageal stricture, multiple sessions of endoscopic dilatation treatment will be needed, increasing the risk of bleeding, perforation, and other complications, which increases the socioeconomic burden and undermines the patient’s quality of life (10,11). Currently, the common strategy for preventing esophageal stricture after endoscopic treatment is oral administration or local injection of glucocorticoids. Yamaguchi et al. (12,13) showed that oral prednisolone was effective in preventing esophageal stricture after ESD, while systemic glucocorticoids were associated with an increased risk of a variety of adverse effects including infections due to immunosuppression, osteoporosis, elevated blood glucose, visual impairment, psychiatric disorders, and peptic ulcer. Endoscopic esophageal metal stent placement is helpful in preventing esophageal stricture after endoscopic treatment. In a study performed by Wen et al. (14), patients with mucosal defects that exceeded 75% of the circumference of the esophagus after ESD treatment underwent fully covered esophageal stent placement post ESD (group A) or no stent placement (group B). In group A, the esophageal stents were removed 8 weeks post ESD. The proportion of patients who developed a stricture was significantly lower in group A (18.2%, 2/11) than in group B (72.7%, 8/11) (P<0.05). In contrast, Holt et al. (15) found that the incidence of esophageal stricture still reached 57.1% (8/14) after prophylactic esophageal stent insertion following ESD for circumferential esophageal lesions. Thus, the effectiveness of endoscopic placement of fully covered metal stents for preventing post-endoscopic esophageal stricture needs to be further investigated, and patients still face risks of stent migration, granulation tissue growth, chest pain, bleeding, and restenosis. Furthermore, the treatment costs are high, and there is a lack of an economical, effective, and low-risk method to prevent benign strictures following endoscopic treatment of the esophagus in clinical practice.
Botulinum toxin type A (BTX-A), a neurotoxin produced by Clostridium botulinum, has been successfully used in many clinical fields since 1973, including plastic surgery, strabismus, and cardial incontinence (16,17). Recent studies have shown that BTX-A can inhibit the formation of fibrous connective tissues and reduce collagen deposition and scar formation during skin wound healing (18-20), which has inspired investigations on the prevention of scarring esophageal stricture using BTX-A during esophageal injury repair. Wen et al. (21) showed that the incidence of post-ESD esophageal stricture was significantly decreased after local BTX-A injection (11.4%, 4/35 in the BTX-A group vs. 37.8%, 14/37 in the control group). Similarly, Zhou et al. (22) demonstrated that endoscopic local injection of BTX-A effectively lowered the incidence of esophageal strictures in patients undergoing ESD for circumferential esophageal mucosal defects. However, all these clinical studies were preliminary studies based on small sample sizes, and few studies have explored the mechanism of scar formation inhibition by BTX-A during the repair of esophageal mucosal injury. This study is the first investigation on the preventive effect and possible mechanisms of endoscopic injection of BTX-A on scarring esophageal stricture caused by electrocautery in rabbit models. We conducted the study with an attempt to provide a theoretical basis for future clinical studies on the prevention of benign esophageal stricture by BTX-A after esophageal injury. We present the following article in accordance with the ARRIVE reporting checklist (available at https://jtd.amegroups.com/article/view/10.21037/jtd-22-202/rc).
Methods
Experimental animals
Forty male New Zealand Large White rabbits, aged 4–6 months and weighing 2.5±0.2 kg, were purchased from the Experimental Animal Center of the Chinese PLA General Hospital. They were kept in a specific pathogen-free (SPF) animal facility at 20–22 ℃ with food and water ad libitum. The study was approved by the ethics committee of Chinese PLA General Hospital (approval No. 2011-X4-27). The use of rabbits followed the Guide for the Care and Use of Laboratory Animals released by the Ministry of Science and Technology of China in 2006 (23). A protocol was prepared before the study without registration.
Study design
Forty rabbits were randomly divided into 4 groups: cautery group, saline group, BTX-A I group, and BTX-A II group, with 10 rabbits in each group. The benign esophageal stricture models were established using electrocautery in all groups. After 12 hours of fasting, the rabbits were anesthetized with 3% sodium pentobarbital at a dose of 1 mL/kg injected intravenously into the ear vein. The metal end of high-frequency (HF) hemostatic forceps (FD-410LR, Olympus, Japan) was placed in the lower segment of the rabbit esophagus (ended approximately 16–18 cm from the incisors) via an ultra-fine gastroscope (GIF-XP260N, Olympus, Japan). The entire circumference of the esophagus was cauterized using HF hemostatic forceps with a mixed electrocoagulation power of 30 W. The cautery length was approximately 1 cm, and the duration was 4 seconds. Except for the modeling procedure, no other intervention was applied in the cautery group. In the saline group, 2 mL of saline was injected into the esophageal muscle layer at the site of electrocautery in 4 spots (1 spot in each quadrant, 0.5 mL per spot) under direct endoscopic vision immediately after the modeling. In the BTX-A I group, 10 IU of BTX-A (dissolved in 2 mL of saline) was injected into the esophageal muscle layer at the site of electrocautery in 4 spots (1 spot in each quadrant, 0.5 mL per spot) under direct endoscopic vision immediately after the modeling. In the BTX-A II group, 20 IU of BTX-A (dissolved in 2 mL of saline) was injected into the esophageal muscle layer at the site of electrocautery in 4 spots (1 spot in each quadrant, 0.5 mL per spot) under direct endoscopic vision immediately after the modeling. Each group was confirmed to have no bleeding or perforation under endoscopic vision before endoscope withdrawn. Rabbits were fed a normal diet postoperatively. Body weight and esophagographic findings were recorded before surgery and at the 1st, 2nd, and 4th postoperative weeks in all groups. The rabbits were sacrificed by an ear intravenous injection of euthanasia solution after 4 weeks. The esophagus were surgically separated by thoracotomy. Tissues in the cauterized section were harvested for histopathological analysis, and the type I collagen content, type III collagen content, and TGF-β1 mRNA expression in the esophagus tissues were determined.
Esophagography
Esophagography was performed in each group before and at the 1st, 2nd, and 4th postoperative weeks to observe the esophageal strictures. The rabbits were anesthetized with 3% pentobarbital sodium and then fixed on an angiography unit table in a lateral position with head down. An angiographic catheter was placed into the upper segment of the esophagus (about 10 cm from the incisors). The inner diameter of the narrowest part of the electrocauterized segment of the lower esophagus was measured by slowly injecting the compound meglumine diatrizoate through the gastric tube, which was followed by acquisition of radiographs. The preoperative inner diameter values of the esophagus in each group were obtained as the average measurements of the inner diameter of 3 spots in the lower esophagus.
Histopathological examinations
Hematoxylin and eosin (HE) staining and Masson staining were performed on cross sections (4–5 µm) of the retained esophageal tissue. According to literature (24,25), the histopathological changes in the esophagus were analyzed semi-quantitatively in terms of collagen deposition in the submucosal layer, damage to the mucosal muscle layer, damage to the intrinsic muscle layer, and collagen deposition.
Determination of type I collagen content, type III collagen content, and TGF-β1 mRNA expression
Type I collagen and type III collagen contents were measured in the esophageal tissues of the cauterized segments by enzyme-linked immunosorbent assay (ELISA) (Rabbit Tissue Type I Collagen and Type III Collagen Kit, Jiancheng Bioengineering Institute, Nanjing, China).
The mRNA expression of TGF-β1 in cauterized esophageal tissues was detected by reverse transcription-polymerase chain reaction (RT-PCR). The total RNA in the cauterized esophageal tissues was analyzed according to the instructions of the RNA extraction kit (Tiangen Biotech, Beijing, China). Glyceraldehyde phosphate dehydrogenase (GAPDH) was selected as an internal reference. Details of the primer sequences are shown in Table 1. The reverse transcription reaction and PCR were performed according to the instructions of the RT-PCR kit (TaKaRa, Dalian, China), and the PCR procedure was as follows: pre-denaturation at 95 ℃ for 2 min, followed by 45 cycles of 95 ℃ for 20 s, 59 ℃ for 25 s, and 72 ℃ for 30 s. Subsequently, a mixture of 5 µL of PCR products and 1 µL of loading buffer was loaded on 1.5% agarose gel for electrophoresis. As a standard molecular weight reference, PCR Marker was also applied for electrophoresis. GAPDH was used as a positive control. The results were observed under UV light, and the gel images were scanned and recorded. The Gel-Pro Analyzer software was used for grayscaling the bands. The ratio between the optical density (OD) value of the target bands and the OD value of the GAPDH bands was taken as the relative OD, and the results were recorded.
Table 1
Gene | Forward primer | Reverse primer | Product length (bp) |
---|---|---|---|
TGF-β1 | 5'-CCCAAGTGATGATGAGGTGC-3 | 5'-CAATCCAGGTGACGGTAAATG-3' | 428 |
GAPDH | 5'-CCCCAACGTGTCGGTCGTGG -3' | 5'-GGCTCCCTAAGCCCCTCCCC-3' | 462 |
PCR, polymerase chain reaction; TGF-β1, transforming growth factor β1; GAPDH, glyceraldehyde phosphate dehydrogenase.
Statistical analysis
Statistical analyses were performed using SPSS 22.0 statistical software (International Business Machines Corporation, Armonk, New York, USA). All measurement data are presented as mean ± standard deviation (). Comparisons of variables between groups were performed using the paired samples t-test, and comparisons of variables among multiple groups were performed using univariate analysis of variance (ANOVA). Data with uneven variances were analyzed using the Kruskal-Wallis test, and pairwise comparisons of multiple means among groups with differences were performed using the LSD t-test. A P value of <0.05 was considered statistically significant.
Results
Four rabbits died early during the experiment: 2 (1 each in the BTX-A I group and BTX-A II group) died due to anesthesia accident. Another rabbit in the BTX-A I group died due to mediastinal inflammation caused by esophageal perforation during the insertion of HF hemostatic forceps into the esophagus. One rabbit in the cautery group died of respiratory failure due to inadvertent aspiration of contrast agent into the respiratory tract during esophagography.
Diets and body weight of experimental animals
Rabbits in all groups showed varying degrees of reduced food intake and depression during the 1st postoperative week. Rabbits in the cautery group and saline group showed no improvement in these conditions and experienced progressive weight loss 1 week after surgery. In the BTX-A I and BTX-A II groups, however, the mental status and food intake gradually returned to normal 2 weeks after surgery. The body weights were not significantly different among the 4 groups before surgery (P=0.688), though they decreased significantly in the 1st postoperative week, showing no significant difference among the 4 groups (P=0.966). At the 2nd and 4th postoperative weeks, the body weights of the cautery and saline groups continued to decrease, while the body weights of the BTX-A I and BTX-A II groups gradually stabilized and became significantly higher than those of the cautery and saline groups (P<0.01). At the 2nd and 4th postoperative weeks, the body weights showed no significant differences between the cautery and saline groups and between the BTX-A I and BTX-A II groups (both P>0.05) (Figure 1).
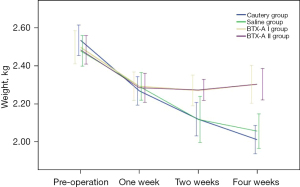
Esophagographic findings
Esophagography showed no significant difference in the internal diameter of the esophagus among the 4 groups before surgery (P=0.849). At the 1st postoperative week, the internal diameter significantly decreased in all groups, and there was no significant difference among these groups (P=0.882). At postoperative weeks 2 and 4, the inner diameter was significantly smaller in both the cautery and saline groups than in the BTX-A I and BTX-A II groups (all P<0.01). However, there was no significant difference between the cautery and saline groups (P=0.999 at week 2 and P=0.551 at week 4) or between the BTX-A I and BTX-A II groups (P=0.994 at week 2 and P=0.463 at week 4) (Figures 2,3).
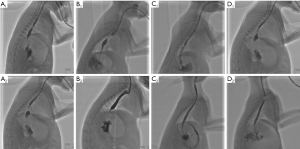
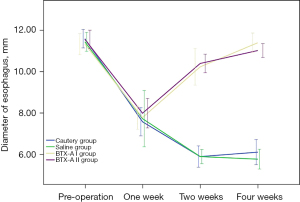
Histopathological examinations
Microstructural changes of esophageal tissues
In the cautery and saline groups, the epithelial layer of the esophageal mucosa was thinned and structurally disorganized, along with loss of papilla-like structures, cell necrosis, disorganized and absent structures of the intrinsic mucosal layer and myxomucosal layer, thickening of the submucosal layer, and reduction or disappearance of normal glands. In addition, a large number of disorganized collagen fibers and edema of the intrinsic muscle layer were visible. Masson staining showed that the structure of the mucosal muscle layer was unclear or missing, partially replaced by blue collagen fibers. A large number of blue collagen fibers were deposited in the submucosal layer, accompanied by inflammatory cell infiltration. Blue collagen fibers were also deposited in the intrinsic muscle layer, and some smooth muscle fibers were replaced by collagen fibers. In the BTX-A I and BTX-A II groups, a flat continuous double epithelial layer with uneven epithelial layer thickness was seen in the esophageal mucosa. A small number of collagen fibers were visible in the submucosa. After Masson staining, it was observed in the mucosal muscle layer that a small number of blue collagen fibers replaced the smooth muscle fibers. Some inflammatory cell infiltration was seen in the submucosal layer. In some tissues, slight blue collagen deposition was observed in the submucosal layer after Masson staining. The intrinsic muscle layer and outer membrane were intact, and no obvious abnormalities were seen (Figure 4).
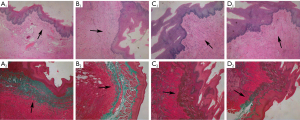
Histopathological scoring of the esophagus
The pathological scores in the cautery and saline groups were significantly higher than those in the BTX-A I and BTX-A II groups (all P<0.01). However, there was no significant difference in pathological scores between the cautery and saline groups (P=0.23) or between the BTX-A I and BTX-A II groups (P=0.91) (Table 2).
Table 2
Group | n | Histopathological score | Type I collagen (ng/mL) | Type III collagen (ng/mL) | TGF-β1/GAPDH |
---|---|---|---|---|---|
Cautery group | 9 | 3.000±0.707* | 75.756±4.513* | 14.059±0.707* | 0.691±0.046* |
Saline group | 10 | 2.600±0.843 | 71.008±6.722 | 13.531±1.447 | 0.667±0.066 |
BTX-A I group | 8 | 1.375±0.518# | 57.760±10.529# | 10.140± 1.201∆ | 0.581±0.767∆ |
BTX-A II group | 9 | 1.333±0.707 | 55.387±5.996 | 8.637±0.960 | 0.497±0.113 |
*, P>0.05, compared with the saline group; P<0.01, compared with the BTX-A I and BTX-A II groups. #, P>0.05, compared with the BTX-A II group; ∆, P<0.05, compared with the BTX-A II group. TGF-β1, transforming growth factor β1; BTX-A, botulinum toxin type A.
Type I collagen and type III collagen content in esophageal tissues
The content of type I collagen and type III collagen in esophageal tissues was measured by ELISA, and it was found that the content of type I collagen and type III collagen in the cautery and saline groups was significantly higher than that in the BTX-A I and BTX-A II groups (all P values <0.01), while the difference between the cautery and saline groups was not statistically significant (P>0.05). The type I collagen content in the BTX-A I group was slightly higher than that in the BTX-A II group, but the difference was not statistically significant (P=0.50), while the type III collagen content was significantly higher in the BTX-A I group than that in the BTX-A II group (P=0.01) (Table 2).
TGF-β1 mRNA expression in esophageal tissues
The mRNA expression of TGF-β1 in the cautery and saline groups was significantly higher than that in the BTX-A I and BTX-A II groups (all P<0.01), while there was no significant difference between the cautery and saline groups (P=0.53). The mRNA expression of TGF-β1 was significantly lower in the BTX-A II group compared with the BTX-A I group (P=0.04) (Figure 5, Table 2).
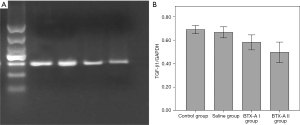
Discussion
BTX-A is a neurotoxin produced by Clostridium botulinum. Since it has a highly specific effect on cholinergic nerve endings with few adverse effects, BTX-A is widely used in plastic surgery and the treatment of diseases associated with dystonia, abnormal muscle contraction, autonomic instability, and other relevant disorders (e.g., strabismus, blepharospasm, facial muscle spasm, spastic bladder, achalasia, and hyperhidrosis). Further studies have revealed that topical application of BTX-A can inhibit collagen deposition and fibrous connective tissue formation during the repair of injuries in different tissues and organs such as skin, urethra, and joints, thereby reducing scar formation and local stenosis (26-30). Two clinical studies with small sample sizes have also explored the role of BTX-A in preventing post-ESD esophageal strictures and found it was effective in preventing scarring stricture (21,22). The current study is the first investigation on the preventive effect of endoscopic injection of BTX-A on scarring esophageal stricture caused by cauterization in rabbit models and explored its possible mechanisms. Referring to previous study (31), we used 10 IU BTX-A, a safe and effective dose, as the therapeutic dose. We tried 20 IU BTX-A as a high dose group to investigate whether increasing the dose of BTX-A can improve the prevention of esophageal stricture. BTX-A was injected into the four quadrants of the esophagus at four points in order to evenly distribute BTX-A at the electrocautery site of the esophagus. Both the cautery and saline groups showed significant feeding difficulties and weight loss after esophageal cauterization, and esophagography revealed obvious esophageal stricture formation. In contrast, the 2 groups given local injection of BTX-A showed less weight loss than the cautery and saline groups, and no significant esophageal strictures were found on esophagograms. Histopathological examinations showed that the submucosal layer of the esophagus was significantly thickened 4 weeks after cauterization, along with the deposition of a large number of collagen fibers, destroyed structure of the mucosal muscle layer, and partial replacement by collagen fibers. In addition, swelling and collagen deposition were also found in the intrinsic muscle layer. However, the histopathological changes were milder in the 2 groups that received BTX-A injection. Additionally, in these groups, the mucosal muscular layer was intact, no massive collagen fiber deposition was seen in the submucosal and muscular layers, and the histopathological scores were significantly lower than those in the cautery and saline groups. Therefore, endoscopic local injection of BTX-A relieves esophageal strictures caused by cautery both symptomatically and histopathologically. It can reduce collagen deposition in local esophageal tissues and thus has a preventive effect on the formation of scarring esophageal strictures caused by cautery in rabbits.
In the process of tissue repairing, repeated microtrauma caused by muscle contraction around the damaged tissue induces a prolonged and strong inflammatory response, then stimulates collagen and mucopolysaccharide deposition, resulting in excessive scar formation (18). BTX-A acts at the neuromuscular junction by blocking vesicle transport of acetylcholine and inhibits muscle contraction. It is indicated that BTX-A may reduce esophageal scarring formation by decreasing the muscle contraction and microtrauma during the process of esophageal tissue repairing. Scarring stricture after esophageal burns is mainly due to the massive secretion of extracellular matrix molecules (ECMs, which mainly include substances such as type I and III collagen) by fibroblasts during the repair of the burned tissue. These ECMs are deposited in large quantities during tissue repair and cannot be fully absorbed and remodeled by the organism at later stages, which eventually leads to proliferative scar formation and esophageal stenosis. TGF-β1 is the main regulator of post-traumatic tissue repair and scar formation. It can act directly or indirectly, alone or synergistically, on inflammatory and repair cells through paracrine and autocrine actions, thus regulating cell growth, differentiation, adhesion, and apoptosis. With a potent chemotactic effect on fibroblasts, TGF-β1 can promote the transformation of fibroblasts into myofibroblasts, increase the synthesis and secretion of ECMs, inhibit the activity of proteases and matrix enzymes, and reduce the degradation of collagen (32). High TGF-β1 expression in tissues plays a key role not only in the formation of proliferative scars in skin but also in the fibrosis of other tissues and organs such as the esophagus, liver, kidneys, and lungs (33-35). Previous studies have shown that BTX-A can inhibit the expression of TGF-β1 during the repair of animal body surface wounds, induce apoptosis in in vitro cultured fibroblasts, inhibit the secretion of TGF-β1 by fibroblasts, and reduce the synthesis and secretion of collagen (18,19). In our current study, RT-PCR revealed that the TGF-β1 mRNA levels in esophageal tissues were lower in the BTX-A I and II groups compared with those in the cautery and saline groups. According to previous literature, the low TGF-β1 mRNA expression in the esophageal tissues of the 2 BTX-A groups may be due to the induction of apoptosis of local esophageal tissue fibroblasts after BTX-A injection, which reduced the synthesis and secretion of TGF-β1 by fibroblasts. Also, in our present study, the levels of type I and type III collagen in esophageal tissues were significantly lower in the BTX-A I and II groups than in the cautery and saline groups. Since TGF-β1 plays an important regulatory role in the synthesis, secretion, and degradation of type I and type III collagen during tissue repair, high TGF-β1 expression promotes the deposition of type I and type III collagen. Thus, the low levels of type I and type III collagen in esophageal tissues in the BTX-A I and BTX-A II groups were associated with their low levels of TGF-β1 expression. Thus, we assumed that the mechanism of local injection of BTX-A to prevent scarring stricture after esophageal burns in rabbits was that BTX-A down-regulated the expression of TGF-β1 in the esophageal tissue at the burn site, which inhibited the deposition of type I and type III collagen and ultimately alleviated post-burn scarring esophageal stricture.
In the present study, although the TGF-β1 mRNA expression level was significantly lower in the BTX-A II group than in the BTX-A I group, these 2 groups showed no significant differences in body weight changes, internal diameter of the esophagus, and histopathological scores. Thus, we speculate that further increasing the dose of BTX-A after reaching a certain effective dose may have a limited effect on alleviating post-cautery scarring esophageal stricture.
Although several small sample clinical trials have shown that BTX-A has preventive effect on benign esophageal strictures, our study is the first to explore the mechanism of BTX-A in preventing scarring esophageal stricture in animal models. Limitations of this study include its animal model of esophageal stricture. In our study, only one type of benign esophageal stricture model (stricture caused by electrocautery) has been investigated, lacking of esophageal stricture model caused by ESD. Esophageal stricture after ESD is more common in clinic setting. The preventive effect and mechanism of BTX-A on different types of benign esophageal stricture, such as strictures caused by ESD and gastroesophageal reflux disease, could be explored in future studies.
Conclusions
Local injection of BTX-A can reduce collagen deposition in local esophageal tissues after cautery in rabbits and effectively prevent the formation of scarring esophageal stricture. The possible mechanism may be that BTX-A down-regulates the expression of TGF-β1 in the esophageal tissue at the burn site.
Acknowledgments
Funding: None.
Footnote
Reporting Checklist: The authors have completed the ARRIVE reporting checklist. Available at https://jtd.amegroups.com/article/view/10.21037/jtd-22-202/rc
Data Sharing Statement: Available at https://jtd.amegroups.com/article/view/10.21037/jtd-22-202/dss
Conflicts of Interest: All authors have completed the ICMJE uniform disclosure form (available at https://jtd.amegroups.com/article/view/10.21037/jtd-22-202/coif). The authors have no conflicts of interest to declare.
Ethical Statement: The authors are accountable for all aspects of the work in ensuring that questions related to the accuracy or integrity of any part of the work are appropriately investigated and resolved. The study was approved by the ethics committee of PLA General Hospital (approval No. 2011-X4-27). The use of rabbits followed the Guide for the Care and Use of Laboratory Animals released by the Ministry of Science and Technology of China in 2006.
Open Access Statement: This is an Open Access article distributed in accordance with the Creative Commons Attribution-NonCommercial-NoDerivs 4.0 International License (CC BY-NC-ND 4.0), which permits the non-commercial replication and distribution of the article with the strict proviso that no changes or edits are made and the original work is properly cited (including links to both the formal publication through the relevant DOI and the license). See: https://creativecommons.org/licenses/by-nc-nd/4.0/.
References
- Repici A, Conio M, De Angelis C, et al. Temporary placement of an expandable polyester silicone-covered stent for treatment of refractory benign esophageal strictures. Gastrointest Endosc 2004;60:513-9. [Crossref] [PubMed]
- Patterson DJ, Graham DY, Smith JL, et al. Natural history of benign esophageal stricture treated by dilatation. Gastroenterology 1983;85:346-50. [Crossref] [PubMed]
- Marks RD, Richter JE. Peptic strictures of the esophagus. Am J Gastroenterol 1993;88:1160-73. [PubMed]
- Spechler SJ. AGA technical review on treatment of patients with dysphagia caused by benign disorders of the distal esophagus. Gastroenterology 1999;117:233-54. [Crossref] [PubMed]
- Katada C, Muto M, Manabe T, et al. Esophageal stenosis after endoscopic mucosal resection of superficial esophageal lesions. Gastrointest Endosc 2003;57:165-9. [Crossref] [PubMed]
- Ono S, Fujishiro M, Niimi K, et al. Predictors of postoperative stricture after esophageal endoscopic submucosal dissection for superficial squamous cell neoplasms. Endoscopy 2009;41:661-5. [Crossref] [PubMed]
- Shi Q, Ju H, Yao LQ, et al. Risk factors for postoperative stricture after endoscopic submucosal dissection for superficial esophageal carcinoma. Endoscopy 2014;46:640-4. [Crossref] [PubMed]
- Hanaoka N, Ishihara R, Takeuchi Y, et al. Intralesional steroid injection to prevent stricture after endoscopic submucosal dissection for esophageal cancer: a controlled prospective study. Endoscopy 2012;44:1007-11. [Crossref] [PubMed]
- Hashimoto S, Kobayashi M, Takeuchi M, et al. The efficacy of endoscopic triamcinolone injection for the prevention of esophageal stricture after endoscopic submucosal dissection. Gastrointest Endosc 2011;74:1389-93. [Crossref] [PubMed]
- Siersema PD. Treatment options for esophageal strictures. Nat Clin Pract Gastroenterol Hepatol 2008;5:142-52. [Crossref] [PubMed]
- Yu JP, Liu YJ, Tao YL, et al. Prevention of Esophageal Stricture After Endoscopic Submucosal Dissection: A Systematic Review. World J Surg 2015;39:2955-64. [Crossref] [PubMed]
- Yamaguchi N, Isomoto H, Nakayama T, et al. Usefulness of oral prednisolone in the treatment of esophageal stricture after endoscopic submucosal dissection for superficial esophageal squamous cell carcinoma. Gastrointest Endosc 2011;73:1115-21. [Crossref] [PubMed]
- Yamaguchi N, Isomoto H, Shikuwa S, et al. Effect of oral prednisolone on esophageal stricture after complete circular endoscopic submucosal dissection for superficial esophageal squamous cell carcinoma: a case report. Digestion 2011;83:291-5. [Crossref] [PubMed]
- Wen J, Lu Z, Yang Y, et al. Preventing stricture formation by covered esophageal stent placement after endoscopic submucosal dissection for early esophageal cancer. Dig Dis Sci 2014;59:658-63. [Crossref] [PubMed]
- Holt BA, Jayasekeran V, Williams SJ, et al. Early metal stent insertion fails to prevent stricturing after single-stage complete Barrett's excision for high-grade dysplasia and early cancer. Gastrointest Endosc 2015;81:857-64. [Crossref] [PubMed]
- Jankovic J, Brin MF. Therapeutic uses of botulinum toxin. N Engl J Med 1991;324:1186-94. [Crossref] [PubMed]
- de Maio M. Therapeutic uses of botulinum toxin: from facial palsy to autonomic disorders. Expert Opin Biol Ther 2008;8:791-8. [Crossref] [PubMed]
- Lee BJ, Jeong JH, Wang SG, et al. Effect of botulinum toxin type a on a rat surgical wound model. Clin Exp Otorhinolaryngol 2009;2:20-7. [Crossref] [PubMed]
- Xiao Z, Zhang F, Lin W, et al. Effect of botulinum toxin type A on transforming growth factor beta1 in fibroblasts derived from hypertrophic scar: a preliminary report. Aesthetic Plast Surg 2010;34:424-7. [Crossref] [PubMed]
- Kasyanju Carrero LM, Ma WW, Liu HF, et al. Botulinum toxin type A for the treatment and prevention of hypertrophic scars and keloids: Updated review. J Cosmet Dermatol 2019;18:10-5. [Crossref] [PubMed]
- Wen J, Lu Z, Linghu E, et al. Prevention of esophageal strictures after endoscopic submucosal dissection with the injection of botulinum toxin type A. Gastrointest Endosc 2016;84:606-13. [Crossref] [PubMed]
- Zhou X, Chen H, Chen M, et al. Comparison of endoscopic injection of botulinum toxin and steroids immediately after endoscopic submucosal dissection to prevent esophageal stricture: a prospective cohort study. J Cancer 2021;12:5789-96. [Crossref] [PubMed]
- On the release of the "Guide for the Care and Use of Laboratory Animals" notice. Chinese Journal of Animal Husbandry and Veterinary Medicine 2007;4:35-36.
- Berthet B, di Costanzo J, Arnaud C, et al. Influence of epidermal growth factor and interferon gamma on healing of oesophageal corrosive burns in the rat. Br J Surg 1994;81:395-8. [Crossref] [PubMed]
- Guven A, Demirbag S, Uysal B, et al. Effect of 3-amino benzamide, a poly(adenosine diphosphate-ribose) polymerase inhibitor, in experimental caustic esophageal burn. J Pediatr Surg 2008;43:1474-9. [Crossref] [PubMed]
- Zhibo X, Miaobo Z. Intralesional botulinum toxin type A injection as a new treatment measure for keloids. Plast Reconstr Surg 2009;124:275e-7e. [Crossref] [PubMed]
- Xiao Z, Zhang F, Cui Z. Treatment of hypertrophic scars with intralesional botulinum toxin type A injections: a preliminary report. Aesthetic Plast Surg 2009;33:409-12. [Crossref] [PubMed]
- Khera M, Boone TB, Smith CP. Botulinum toxin type A: a novel approach to the treatment of recurrent urethral strictures. J Urol 2004;172:574-5. [Crossref] [PubMed]
- Namazi H, Torabi S. Novel use of botulinum toxin to ameliorate arthrofibrosis: an experimental study in rabbits. Toxicol Pathol 2007;35:715-8. [Crossref] [PubMed]
- Sahinkanat T, Ozkan KU, Ciralik H, et al. Botulinum toxin-A to improve urethral wound healing: an experimental study in a rat model. Urology 2009;73:405-9. [Crossref] [PubMed]
- Kim HS, Hwang JH, Jeong ST, et al. Effect of muscle activity and botulinum toxin dilution volume on muscle paralysis. Dev Med Child Neurol 2003;45:200-6. [Crossref] [PubMed]
- Verrecchia F, Mauviel A. Transforming growth factor-beta signaling through the Smad pathway: role in extracellular matrix gene expression and regulation. J Invest Dermatol 2002;118:211-5. [Crossref] [PubMed]
- Cutroneo KR. TGF-beta-induced fibrosis and SMAD signaling: oligo decoys as natural therapeutics for inhibition of tissue fibrosis and scarring. Wound Repair Regen 2007;15:S54-60. [Crossref] [PubMed]
- Lan HY. Diverse roles of TGF-beta/Smads in renal fibrosis and inflammation. Int J Biol Sci 2011;7:1056-67. [Crossref] [PubMed]
- Zhou JH, Jiang YG, Wang RW, et al. Prevention of stricture development after corrosive esophageal burn with a modified esophageal stent in dogs. J Thorac Cardiovasc Surg 2008;136:1336-42, 1342.e1-7.
(English Language Editor: C. Betlazar-Maseh)