MiR-223-3p affects myocardial inflammation and apoptosis following myocardial infarction via targeting FBXW7
Introduction
Myocardial infarction (MI) is a common cardiovascular disease in clinical practice which seriously endangers human health (1). The incidence and mortality rates of MI are increasing gradually. It can cause the increase of oxygen free radicals and calcium overload of cardiomyocytes, aggravate cardiomyocyte apoptosis and necrosis, and its clinical manifestations are arrhythmia, expansion of MI area, deterioration of cardiac function, and ventricular remodeling (2,3). Cardiomyocyte apoptosis, inflammation and cytokines are involved in a series of pathological changes of ventricular remodeling and heart failure after MI, which lead to disease progression and poor prognosis in patients with MI (4). MicroRNAs (miRNAs, MiRs) are tissue-specific non-coding single stranded small RNA with high conservation. They are composed of 19–25 nucleotides and regulate protein expression (5). Studies have shown that miRNAs play an important role in the pathophysiological processes of a variety of cardiovascular diseases, such as myocardial hypertrophy, arrhythmia, myocardial ischemia, and so on (6,7). The tumor suppressor gene miR-223-3p can inhibit the proliferation and migration of a variety of malignant tumors (8,9). Long et al. showed that miR-223-3p could inhibit inflammatory response (10). However, no study has yet been conducted on the regulatory mechanism of miR-223-3p in MI. The FBXW7 gene is one of the tumor suppressors that promote human tumor cell apoptosis. Study has shown that FBXW7 may regulate the occurrence and development of inflammatory response by affecting TLR4 inflammatory signaling pathway (11).
In this study, we determined that the expression of miR-223-3p decreased in an MI cell model and rat model, and may reduce myocarditis and apoptosis after MI by targeting the inhibition of FBXW7 expression. Our study suggests that miR-223-3p may become a new therapeutic target for MI patients. This study further improved the mechanism of myocarditis and apoptosis after MI, and provided a new theoretical basis for clinical treatment or auxiliary diagnosis. We present the following article in accordance with the ARRIVE reporting checklist (available at https://jtd.amegroups.com/article/view/10.21037/jtd-22-82/rc).
Methods
Cell culture and treatment
We cultured H9c2 cells (purchased from Hunan Fenghui Biotechnology Co., Ltd., Hunan, China) in an incubator containing 10% fetal bovine serum (FBS; Gibco Thermo Fisher Scientific, Waltham, MA, USA) and 1% penicillin/streptomycin (Merck, Kenilworth, NJ, USA) at 37 ℃ with Dulbecco’s modified Eagle medium (DMEM; Invitrogen, Carlsbad, CA, USA). Then, when the cell fusion rate reached about 80%, the H9c2 cells were subcultured. After the cells had been starved in serum-free medium for 12 h, they were treated with H2O2 at different concentrations of 50, 100, 200, and 400 µm for 24 h.
Construction and treatment of MI model in rats
A protocol was prepared before the study without registration. Experiments were performed under a project license (No. 2018-LHKY-019-02) granted by the Ethics Committee of Beijing Luhe Hospital, Capital Medical University, in compliance with national standard GB/T 35892-2018 laboratory animal guidelines for the care and use of animals. Adult male Sprague Dawley (SD) rats have good tolerance to the construction of MI model. In this study, a total of 40 male SD rats, 8 weeks old, nonspecific pathogen grade, weighing 200–220 g, were purchased from Southern Medical University. The rats were housed under standard conditions, with controlled 12-hour light/dark cycle, temperature and humidity, unrestricted activity, and free access to water and food. Rats were given adaptive feeding for 2 weeks before the experiment. Compared with mimic, agonist injection has a higher success rate. According to the random number table method, the rats were randomly divided into a sham group, MI group, MI + agoNC group, and MI + agomiR-223-3p group, with 10 rats in each group. All rats were anesthetized with sodium pentobarbital (30 mg/kg), fixed on the workbench in the supine position, and connected to the small animal ventilator after endotracheal intubation. The rats in MI group, MI + agoNC group, and MI + agomiR-223-3p group underwent thoracotomy and ligated the anterior descending branch of the left coronary artery. Sham group rats underwent thoracotomy without ligation of the left anterior descending branch. AgomiR-223-3p (RiboBio, Guangzhou, China) is the same double stranded RNA analog as mature miR-223-3p. The day before operation, rats were injected with agomiR-223-3p (5 mg/kg) or agoNC through the caudal vein. The expression of miR-223-3p in rat cardiac tissue was detected on the 1st, 3rd, and 7th day after operation. In the MI + agomiR-223-3p group, 2 rats without successful overexpression were excluded and 8 rats were finally included. There were 10 rats in each of the other groups. The cardiac function of rats was measured by echocardiography on the 7th day after operation.
TUNEL staining
The rats were euthanized by cutting off their tails and their hearts were subsequently removed. The heart was fixed with 10% formaldehyde and 30% sucrose. After rapid freezing, the heart tissues of rats in each group were cut into 5 µM thick slices. Thermally induced epitopes were extracted in 10 mm citrate buffer (pH 6.0), followed by 5% goat serum blocking and streptavidin/biotin blocking (Vector Laboratories, Inc. Burlingame, CA, USA). Heart sections were stained with terminal deoxynucleotidyl transferase dUTP nick end labeling (TUNEL) by DeadEnd colorimetric TUNEL system (Promega, Madison, WI, USA) at 4 ℃. Staining was analyzed by Zeiss 780 laser scanning microscope (Carl Zeiss, Thornwood, NY, USA). We detected TUNEL positive nuclei in apoptotic cardiomyocytes.
Cell grouping and transfection
Cell grouping and transfection were conducted to explore the regulatory mechanism of miR-223-3p in MI model in vitro. The fusion degree of cultured H9c2 cells was about 80%. At the same time, NC mimic, miR-223-3p mimic, NC vector, and FBXW7 vector were transfected into H9c2 cells with Lipofectamine 3000 (Invitrogen, USA). At 48 h after transfection, the cells were treated with 100 µm H2O2 for 4 h to construct an in vitro model of MI.
Luciferase activity assay
The biological prediction website TargetScan (http://www.targetscan.org/vert_80/) was used to analyze the target gene of miR-223-3p to verify whether FBXW7 is the direct target of miR-223-3p. The target gene FBXW7 double luciferase reporter gene vector and mutants mutated with miR-223-3p binding site: pglo-FBXW7 WT and pglo-FBXW7 mut were constructed respectively. The 2 reporter plasmids were co transfected with overexpression plasmid miR-223-3p mimic and negative control plasmid into fibroblast-like synovial cells respectively. After 24 h of transfection, the cells were lysed, centrifuged at 12,000 rpm for 1 min, and the supernatant was collected. Dual luciferase reporter gene detection system (Reporter assay system, e1910; Promega, USA) was used to detect luciferase activity. Then, 100 µL firefly luciferase working solution was added to each cell sample to detect firefly luciferase, and 100 µL Renilla luciferase working solution was added to detect Renilla luciferase. Firefly luciferase and Renilla luciferase were used as the relative luciferase activities.
MTT
The H9c2 cells were inoculated into 96 well plates, and the number of cells per well was 5×103. The cells were transfected after being cultured in a constant temperature incubator for 24 h. After 48 h of transfection, the cells were treated with H2O2. After further incubation for 4 h, 10 µL of 3-(4,5-dimethylthiazol-2-yl)-2,5-diphenyltetrazolium bromide (MTT) was added to each well (10 mg/mL) (Sigma Aldrich, St. Louis, MO, USA), and 96 well plates were placed in the incubator for 4 h. Then, a pipette was used to remove the liquid from all holes and add 150 µL dimethyl sulfoxide (DMSO) to each hole. Absorbance at 570 nm was measured using a microplate reader (Thermo Fisher Scientific, USA).
Flow cytometry
The apoptosis of H9c2 cells in each group was detected by annexin V-FITC apoptosis detection kit (Beyotime, Shanghai, China). First, H9c2 cells were washed twice with ice cold phosphate-buffered saline (PBS) and suspended with 100 µL binding buffer. After staining with annexin V- FITC and propidium iodide (PI), the proportion of apoptosis was detected by CytoFLEX flow cytometry (Becton, Dickinson, and Co., Franklin Lakes, NJ, USA).
ELISA
The supernatant of H9c2 cells and rat heart tissue in each group were detected for the inflammatory factors tumor necrosis factor-α (TNF-α), interleukin-6 (IL-6), and interleukin-10 (IL-10). After the samples were processed according to the operating instructions, the optical density (OD) value of each group of samples at 450 nm was detected by spectrophotometer (Thermo Fisher Scientific, USA), and the contents of TNF-α, IL-6, and IL-10 in each group of samples were obtained. The enzyme-linked immunosorbent assay (ELISA) kit for determining TNF-α content was purchased from Cell Signaling Technology (CST; Danvers, MA, USA). The kits used to determine the contents of IL-6 and IL-10 were purchased from Abcam (Cambridge, MA, USA).
qRT-PCR
Total RNA of H9c2 cells was isolated using Trizol. The RNA was reversed into complementary DNA (cDNA) using the universal reverse kit (RP1105-50T, Shanghai Acmec Biochemical Co, Ltd., Shanghai, China) and the miRNA reverse kit (MT0006, Beijing Baiao Laibo Technology Co., Ltd., Beijing, China). The miR-223-3p and U6 primers were designed and synthesized by Sangon Biotech Co., Ltd (Shanghai, China). The amplification procedure was 4 ℃ for 3 min, 94 ℃ for 30 s, 58 ℃ for 30 s, 72 ℃ for 1 min, and the number of cycles was 30. The relative expression was calculated by ΔΔCt. We used U6 as the internal reference for miR-223-3p. The primer sequence of miR-223-3p forward is: 5'– GCGTGTATTTGACAAGCTGAGTT-3', and the primer sequence of miR-223-3p reverse is: 5'– GTGTCAGTTGTCAAATACCCCA-3'. The primer sequence of U6 forward is: 5'- GCTTCGGCAGCACAATACTAAAT-3', and the primer sequence of U6 reverse is: 5'- CGCTTCACGAATTGCGTGTCAT-3'.
Western blot
The total protein was extracted from the H9c2 cells of each group and separated by 10% polyacrylamide gel electrophoresis. The protein was transferred to NC membrane (GE Healthcare, Little Chalfont, UK), which was blocked with 10% skimmed milk in Tris buffered saline with Tween-20 (TBST) solution for 1 h at room temperature, and then cultured overnight at 4 ℃. Primary anti-rabbit anti FBXW7 (ab109617, Abcam, USA), GAPDH (PA1-988, Thermo Fisher Scientific [China] Co., Ltd., Shanghai, China) were incubated. After incubation at room temperature for 2 h, TBST was used for cleaning. The secondary antibody goat anti-rabbit IgG (A21020, AmyJet Scientific Inc., Ltd., Wuhan, China) was incubated. After 2 h of incubation, the protein bands were displayed by chemiluminescence (GE Healthcare) and quantitatively analyzed by GelDoc-2000 imaging system (Bio-Rad, Hercules, CA, USA).
Statistical analysis
The data were analyzed using the software SPSS 21.0 (IBM Corp., Chicago, IL, USA) and expressed as mean ± standard deviation. Student’s t-test or one-way analysis of variance (ANOVA) were used to evaluate the differences between single groups, and two-way ANOVA was used to analyze the differences between multiple groups. A P value <0.05 indicated a statistically significant difference.
Results
The expression of miR-223-3p was down regulated in MI
We detected the expression of miR-223-3p in an MI cell model and rat model. The expression of miR-223-3p was significantly down regulated in H2O2 treated cells (Figure 1A). The expression of miR-223-3p decreased significantly in the infarcted area (Figure 1B) and marginal area (Figure 1C) of MI rats, and there was no significant change in the expression of miR-223-3p in the distal area of myocardium (Figure 1D).
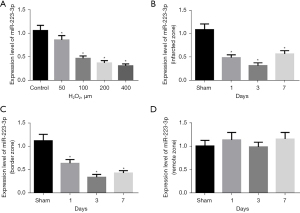
MiR-223-3p inhibits myocarditis and apoptosis after MI
To explore the effect of miR-223-3p in MI cells, we transfected miR-223-3p mimic into H9c2 cells, and the expression of miR-223-3p in miR-223-3p mimic group was significantly higher than that in NC mimic group (Figure 2A). The levels of the cytokines TNF-α, IL-6, and IL-10 were detected by ELISA. After H2O2 treatment, the levels of TNF-α, IL-6, and IL-10 increased significantly. The levels of TNF-α, IL-6, and IL-10 in miR-223-3p overexpression transfected cells decreased significantly (Figure 2B-2D). The survival of cells in each group was detected by MTT. After H2O2 treatment, the survival rate of H9c2 cells decreased significantly. Overexpression of miR-223-3p significantly improved cell survival (Figure 2E). The apoptosis of cells in each group was detected by flow cytometry. After H2O2 treatment, the apoptosis rate of H9c2 cells increased significantly, and the overexpression of miR-223-3p significantly reduced the apoptosis rate (Figure 2F,2G).
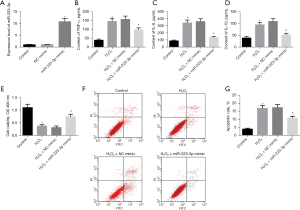
MiR-223-3p directly targeted FBXW7
TargetScan software analysis found that there was a binding site between FBXW7 and miR-223-3p (Figure 3A), which may be the target gene of miR-223-3p. Luciferase activity assay analysis showed that miR-223-3p overexpression inhibited the luciferase activity of FBXW7 WT, but did not inhibit the luciferase activity of FBXW7 mut (Figure 3B). Western blot showed that the expression of FBXW7 protein in H9c2 cells overexpressing miR-223-3p decreased significantly (Figure 3C,3D). The above results showed that FBXW7 is a target gene of miR-223-3p and that miR-223-3p inhibits the expression of FBXW7.
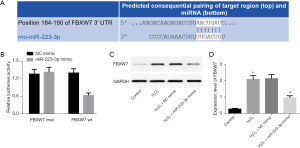
FBXW7 overexpression blocks the regulatory effect of miR-223-3p on myocarditis and apoptosis
To further explore whether FBXW7 affects myocarditis and apoptosis after MI by targeting miR-223-3p, we combined overexpression of miR-223-3p and FBXW7 in H9c2 cells. We used qRT-PCR to detect the expression of miR-223-3p and FBXW7 messenger RNA (mRNA) in cells of each group. The results showed that compared with NC mimic + NC vector group, the expression of miR-223-3p in cells of miR-223-3p mimic + NC vector group increased significantly, the expression of FBXW7 mRNA decreased significantly, and the expression of FBXW7 in cells of NC mimic + FBXW7 vector group increased significantly. Compared with miR-223-3p mimic + NC vector group, the expression of FBXW7 in miR-223-3p mimic + FBXW7 vector group was significantly increased (Figure 4A). The levels of inflammatory factors TNF-α, IL-6, and IL-10 were detected by ELISA. Overexpression of FBXW7 increased the contents of TNF-α, IL-6, and IL-10 in cardiomyocytes, and effectively blocked the inhibitory effect of miR-223-3p overexpression on inflammatory factors (Figure 4B-4D). The survival of cells in each group was detected by MTT. The cell survival rate of FBXW7 overexpression transfected cardiomyocytes was significantly reduced. Overexpression of FBXW7 blocked the promoting effect of miR-223-3p overexpression on cell survival (Figure 4E). The apoptosis of cells in each group was detected by flow cytometry. The apoptosis rate of cells treated with FBXW7 overexpression increased significantly. The apoptosis rate of cells overexpressing miR-223-3p and FBXW7 was significantly higher than that of cells overexpressing miR-223-3p alone (Figure 4F,4G). The above results show that FBXW7 overexpression can effectively block the effect of miR-223-3p on myocarditis and apoptosis.
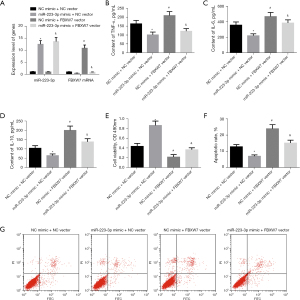
Overexpression of miR-223-3p inhibited myocarditis and apoptosis after MI in rats
Intravenous injection of agomiR-223-3p increased the expression level of miR-223-3p in rat cardiomyocytes (Figure 5A). After miR-223-3p overexpression, the cardiac function of rats was significantly improved (Figure 5B-5D). The results of ELISA showed that after overexpression of mir-223-3p, the contents of inflammatory factor indexes TNF-α, IL-6, and IL-10 in rat cardiomyocytes were significantly reduced, and myocarditis was significantly improved (Figure 5E-5H). The TUNEL staining showed that cardiomyocyte apoptosis decreased significantly after miR-223-3p overexpression (Figure 5G). Meanwhile, western blot showed that the expression level of FBXW7 protein decreased significantly in rats injected with miR-223-3p overexpression (Figure 5I). The above results suggest that miR-223-3p may inhibit myocarditis and apoptosis after MI by inhibiting the expression of FBXW7.
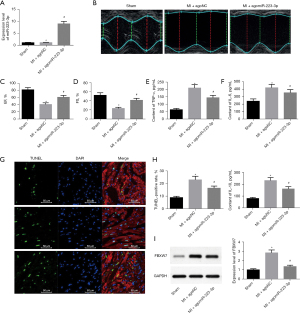
Discussion
As an ischemic heart disease, MI leads to myocardial cell injury and death due to the interruption of local blood supply to the heart. The resulting oxygen deficiency and myocardial ischemia lead to serious myocardial injury and even death (12). Its high incidence and mortality rates are major health problems worldwide.
When MI occurs, myocardial cells are irreversibly damaged and become necrotic due to hypoxia and decreased supply of adenosine triphosphate (ATP). After releasing their contents, necrotic cells activate the autoimmune system, resulting in a severe inflammatory response (12,13). Among the cytokines involved, the TNF, interleukin, and transforming growth factor families will further affect the survival of cardiomyocytes, change vascular endothelial function, and recruit inflammatory cells to the injured myocardium, so as to aggravate or improve the local injury of ischemic myocardium (14,15). The persistence of inflammation will lead to matrix degradation and cardiomyocyte apoptosis (16). Reducing myocardial inflammatory response and apoptosis and maintaining cardiac function is also an important link to delay the course of MI. Mounting experiments show that a large number of cardiomyocyte apoptosis occurs after reperfusion, which may be related to increasingly serious reactions caused by the production of oxygen free radicals (17,18). Inflammation can be the initiating factor of apoptosis, such as cytokine release, neutrophil infiltration, and so on. The occurrence of apoptosis also provides conditions for the aggravation of inflammation, such as the change of intracellular environment and the recruitment of macrophages (19,20). Although the exact mechanism between inflammation and apoptosis has not been clarified, a large number of experiments have shown that reducing cardiomyocyte apoptosis by inhibiting inflammatory response can improve cardiac function and inhibit ventricular remodeling (21,22).
An miRNA is a kind of noncoding single stranded RNA molecule with a length of about 22 nucleotides encoded by endogenous genes found in eukaryotic cells. It participates in the mediation of eukaryotic differentiation, proliferation, growth, and apoptosis by regulating the transcription of its target genes (23). In the realm of cardiovascular disease, miRNA not only plays an important role in myocardial hypertrophy, myocardial necrosis, and apoptosis through the regulation of its target genes, but also participates in every process of MI (24,25). The miRNA miR-223-3p is a tumor suppressor. Ji et al. showed that miR-223-3p can inhibit the metastasis and progression of osteosarcoma (26). Wu et al. reported that miR-223-3p can regulate the expression of inflammatory factors (27). The results of our study showed that the expression of miR-223-3p was down regulated in both an MI cell model and rat model. Overexpression of miR-223-3p can inhibit myocardial inflammatory response and apoptosis.
While exploring the possible regulatory mechanism of miR-223-3p in MI with TargetScan software, we found that there was a binding site between miR-223-3p and FBXW7. Our study confirmed that miR-223-3p could target and inhibit the expression of FBXW7. In the MI rat model, overexpression of miR-223-3p was shown to reduce the expression of FBXW7. Overexpression of FBXW7 can block the regulatory effect of miR-223-3p overexpression on myocarditis and regulation.
In conclusion, miR-223-3p may inhibit the inflammatory response and apoptosis of cardiomyocytes after MI and improve cardiac function by inhibiting the expression of FBXW7. These results suggest that miR-223-3p may be a new regulatory factor in the treatment of MI. The regulatory network of miR-223-3p in MI still needs to be further explored. Whether miR-223-3p is suitable for clinical treatment of MI still requires further research.
Acknowledgment
Funding: None.
Footnote
Reporting Checklist: The authors have completed the ARRIVE reporting checklist. Available at https://jtd.amegroups.com/article/view/10.21037/jtd-22-82/rc
Data Sharing Statement: Available at https://jtd.amegroups.com/article/view/10.21037/jtd-22-82/dss
Conflicts of Interest: All authors have completed the ICMJE uniform disclosure form (available at https://jtd.amegroups.com/article/view/10.21037/jtd-22-82/coif). The authors declare that they have no conflicts of interest.
Ethical statement: The authors are accountable for all aspects of the work in ensuring that questions related to the accuracy or integrity of any part of the work are appropriately investigated and resolved. Experiments were performed under a project license (No. 2018-LHKY-019-02) granted by the Ethics Committee of Beijing Luhe Hospital, Capital Medical University, in compliance with national standard GB/T 35892-2018 laboratory animal guidelines for the care and use of animals.
Open Access Statement: This is an Open Access article distributed in accordance with the Creative Commons Attribution-NonCommercial-NoDerivs 4.0 International License (CC BY-NC-ND 4.0), which permits the non-commercial replication and distribution of the article with the strict proviso that no changes or edits are made and the original work is properly cited (including links to both the formal publication through the relevant DOI and the license). See: https://creativecommons.org/licenses/by-nc-nd/4.0/.
References
- Koeda Y, Itoh T, Ishikawa Y, et al. A multicenter study on the clinical characteristics and risk factors of in-hospital mortality in patients with mechanical complications following acute myocardial infarction. Heart Vessels 2020;35:1060-9. [Crossref] [PubMed]
- Kim Y, Ahn Y, Cho MC, et al. Current status of acute myocardial infarction in Korea. Korean J Intern Med 2019;34:1-10. [Crossref] [PubMed]
- Toma Y. How to Bail Out Patients with Severe Acute Myocardial Infarction. Heart Fail Clin 2020;16:177-86. [Crossref] [PubMed]
- Campbell KH, Tweet MS. Coronary Disease in Pregnancy: Myocardial Infarction and Spontaneous Coronary Artery Dissection. Clin Obstet Gynecol 2020;63:852-67. [Crossref] [PubMed]
- Xiao Y, Zhao J, Tuazon JP, et al. MicroRNA-133a and Myocardial Infarction. Cell Transplant 2019;28:831-8. [Crossref] [PubMed]
- Verjans R, Peters T, Beaumont FJ, et al. MicroRNA-221/222 Family Counteracts Myocardial Fibrosis in Pressure Overload-Induced Heart Failure. Hypertension 2018;71:280-8. [Crossref] [PubMed]
- Chen Y, Zhao Y, Chen W, et al. MicroRNA-133 overexpression promotes the therapeutic efficacy of mesenchymal stem cells on acute myocardial infarction. Stem Cell Res Ther 2017;8:268. [Crossref] [PubMed]
- Li Q, Jiang B, Qi Y, et al. Long non-coding RNA SLCO4A1-AS1 drives the progression of non-small-cell lung cancer by modulating miR-223-3p/IKKα/NF-κB signaling. Cancer Biol Ther 2020;21:806-14. [Crossref] [PubMed]
- Ren N, Jiang T, Wang C, et al. LncRNA ADAMTS9-AS2 inhibits gastric cancer (GC) development and sensitizes chemoresistant GC cells to cisplatin by regulating miR-223-3p/NLRP3 axis. Aging (Albany NY) 2020;12:11025-41. [Crossref] [PubMed]
- Long FQ, Kou CX, Li K, et al. MiR-223-3p inhibits rTp17-induced inflammasome activation and pyroptosis by targeting NLRP3. J Cell Mol Med 2020;24:14405-14. [Crossref] [PubMed]
- Kuai X, Li L, Chen R, et al. SCFFBXW7/GSK3β-Mediated GFI1 Degradation Suppresses Proliferation of Gastric Cancer Cells. Cancer Res 2019;79:4387-98. [Crossref] [PubMed]
- Inami T, Tsurumi M, Seino Y, et al. Successful treatment of ball-shaped very late thrombus after myocardial infarction. BMJ Case Rep 2014;2014:bcr2014204340. [Crossref] [PubMed]
- Yoneyama K, Ishibashi Y, Koeda Y, et al. Association between acute myocardial infarction-to-cardiac rupture time and in-hospital mortality risk: a retrospective analysis of multicenter registry data from the Cardiovascular Research Consortium-8 Universities (CIRC-8U). Heart Vessels 2021;36:782-9. [Crossref] [PubMed]
- Goedemans L, Bax JJ, Delgado V. COPD and acute myocardial infarction. Eur Respir Rev 2020;29:190139. [Crossref] [PubMed]
- Khan HA, Ekhzaimy A, Khan I, et al. Potential of lipoproteins as biomarkers in acute myocardial infarction. Anatol J Cardiol 2017;18:68-74. [Crossref] [PubMed]
- Gualandro DM, Calderaro D, Yu PC, et al. Acute myocardial infarction after noncardiac surgery. Arq Bras Cardiol 2012;99:1060-7. [Crossref] [PubMed]
- Smolderen KG, Brush A, Dreyer RP. Psychosocial Factors and Recovery After Acute Myocardial Infarction in Younger Women. Curr Cardiol Rep 2019;21:50. [Crossref] [PubMed]
- Tashiro H, Tanaka A, Ishii H, et al. Reduced exercise capacity and clinical outcomes following acute myocardial infarction. Heart Vessels 2020;35:1044-50. [Crossref] [PubMed]
- Latet SC, Hoymans VY, Van Herck PL, et al. The cellular immune system in the post-myocardial infarction repair process. Int J Cardiol 2015;179:240-7. [Crossref] [PubMed]
- Jortveit J, Pripp AH, Langørgen J, et al. Incidence, risk factors and outcome of young patients with myocardial infarction. Heart 2020;106:1420-6. [Crossref] [PubMed]
- Balanescu DV, Donisan T, Deswal A, et al. Acute myocardial infarction in a high-risk cancer population: Outcomes following conservative versus invasive management. Int J Cardiol 2020;313:1-8. [Crossref] [PubMed]
- Yalta K, Yilmaz MB, Yalta T, et al. Late Versus Early Myocardial Remodeling After Acute Myocardial Infarction: A Comparative Review on Mechanistic Insights and Clinical Implications. J Cardiovasc Pharmacol Ther 2020;25:15-26. [Crossref] [PubMed]
- Wojciechowska A, Braniewska A, Kozar-Kamińska K. MicroRNA in cardiovascular biology and disease. Adv Clin Exp Med 2017;26:865-74. [Crossref] [PubMed]
- Mellis D, Caporali A. MicroRNA-based therapeutics in cardiovascular disease: screening and delivery to the target. Biochem Soc Trans 2018;46:11-21. [Crossref] [PubMed]
- Liu Y, Song JW, Lin JY, et al. Roles of MicroRNA-122 in Cardiovascular Fibrosis and Related Diseases. Cardiovasc Toxicol 2020;20:463-73. [Crossref] [PubMed]
- Ji Q, Xu X, Song Q, et al. miR-223-3p Inhibits Human Osteosarcoma Metastasis and Progression by Directly Targeting CDH6. Mol Ther 2018;26:1299-312. [Crossref] [PubMed]
- Wu J, Niu P, Zhao Y, et al. Impact of miR-223-3p and miR-2909 on inflammatory factors IL-6, IL-1ß, and TNF-α, and the TLR4/TLR2/NF-κB/STAT3 signaling pathway induced by lipopolysaccharide in human adipose stem cells. PLoS One 2019;14:e0212063. [Crossref] [PubMed]
(English Language Editor: J. Jones)