Bone metastases in non-small cell lung cancer: a narrative review
Introduction
Approximately 20–30% of patients with non-small cell lung cancer (NSCLC) present with bone metastases at diagnosis, and 35–60% will develop them during their disease course (1-4). Symptoms related to bone metastases, called skeletal related events (SREs), are common and the majority of patients with bone metastases will experience significant pain during their course. While considerable progress has been made in the overall management of metastatic NSCLC, bone metastases remain a common source of morbidity, mortality, and diminished quality of life (5). Additional challenges include difficulty determining response to therapy due to lack of adequate imaging criteria in patients with bone metastases and poor outcomes in response to systemic therapies in patients with bone metastases compared to those without (6). Understanding the molecular pathogenesis of bone metastases is critical to make progress in patients with metastatic lung cancer with bone metastases. The objective of our review is to provide a comprehensive review of the pathophysiology, clinical presentation, management, and factors predicting the development and prognosis of patients with NSCLC with bone metastases. We present the following article in accordance with the Narrative Review reporting checklist (available at https://jtd.amegroups.com/article/view/10.21037/jtd-21-1502/rc).
Methods
Google Scholar and PubMed databases were searched between June 2020 and September 2021 with the following search terms to identify relevant papers for this review (Table 1): “bone metastases AND lung cancer AND pathophysiology”, “bone metastases AND non-small cell lung cancer”, “skeletal related events AND non-small cell lung cancer”, “bone metastases AND lung cancer AND outcomes”, “bone metastases AND lung cancer AND response to therapy”, “bone metastases AND lung cancer AND immunotherapy”, and “bone metastases AND lung cancer AND predictive factors”. Bibliographies of identified papers were reviewed for additional articles of interest (Table S1). Published observational cohort, retrospective studies, randomized controlled trials (RCTs), meta-analysis, and review articles published from 1990–2021 were examined for this review. Manuscripts in non-English languages were not evaluated.
Table 1
Items | Specification |
---|---|
Date of search | 6/1/2020–9/1/2021 |
Databases and other sources searched | Google Scholar, PubMed |
Search terms used | Search terms included “bone metastases AND lung cancer AND pathophysiology”, “bone metastases AND non-small cell lung cancer”, “skeletal related events AND non-small cell lung cancer”, “bone metastases AND lung cancer AND outcomes”, “bone metastases AND lung cancer AND response to therapy”, “bone metastases AND lung cancer AND immunotherapy”, and “bone metastases AND lung cancer AND predictive factors”. Bibliographies of identified papers were reviewed for additional articles of interest |
Timeframe | 1990–2021 |
Inclusion and exclusion criteria | Observational cohort, retrospective studies, RCTs, meta-analysis, and review articles published from 1990–2021 were examined for this review. Manuscripts in non-English languages were excluded |
Selection process | Brendan Knapp independently selected and reviewed all initial articles, with additional review by Siddhartha Devarakonda. Ultimate final article inclusion was determined by all authors |
RCTs, randomized controlled trials.
Discussion
Pathophysiology and molecular biology
The initial steps through which bone metastases are established are likely similar to that of metastatic colonization of other distant sites. First, there is tumor invasion of the surrounding normal tissue and new vessel formation, followed by tumor invasion into the blood vessel. Reduction of E-cadherin, normally involved in cell-to-cell adhesion, allows metastases to develop from the primary tumor (7,8). Matrix metalloproteinases (MMPs) cause the breakdown of the extracellular matrix, increasing invasion into the blood vessel (9). Once in the blood vessel, tumor cells can travel to distant sites (10,11). Chemokines, especially C-X-C motif chemokine 12 (CXCL12) and its receptor C-X-C chemokine receptor 4 (CXCR4) serve a vital role in homing of tumor cells from circulation to bone (Figure 1). Tumor cells express CXCR4 and undergo chemotaxis in response to CXCL12 expressed in the bone marrow and stroma (12,13).
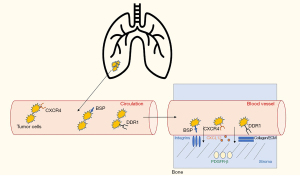
From the blood vessel, tumor cells enter the sinusoids and then the bone marrow stroma. Here, the tumor cells must generate their own blood supply before becoming enriched in the endosteal surface of the bone. Bone sialoprotein (BSP) plays a crucial role in normal bone metabolism. BSP is expressed on NSCLC tumor cells and interacts with integrins (preferentially αVβ3 and αVβ5) (14-17) in the stroma and bone marrow. BSP expression is associated with increased invasiveness, tumor cell growth, attachment, and migration. Platelet derived growth factor receptor beta (PDGFR-β), expressed in the stroma, has also been implicated in tumor growth and invasion (18). In a lung cancer mouse model, PDGFR-β receptor inhibition with sunitinib caused growth inhibition and impaired bone metastases development (19). Discoidin domain receptor-1 (DDR1) is expressed on cancer cells and interacts with collagen in the stroma and bone marrow matrix (20). Inhibition of DDR1, both in vitro and in a lung cancer bone metastases model, was associated with decreased metastatic activity, cell homing, and colonization in a study by Valencia and colleagues (21). Once enriched into the bone, tumor cells can begin to stimulate osteoclast and osteoblast activity through a variety of mechanisms.
Normal bone undergoes constant remodeling involving resorption of bone via osteoclasts and formation of new bone via osteoblasts (Figure 2). Bone metastases are characterized by dysregulation of the normal bone remodeling process and can be radiographically described as osteoblastic, osteolytic, or mixed. Osteolytic metastases are characterized by destruction of normal bone and are common in NSCLC. Osteoblastic metastases are characterized by new bone deposition and are classically found in small cell lung cancer. Most bone metastases have both resorptive and osteoblastic components. Cytokines and other factors more closely associated with osteolytic metastases include parathyroid hormone-related peptide (PTHrP), interleukins (ILs), and perhaps most importantly, the receptor activator of nuclear factor kappa-beta (RANK) and RANK-ligand (RANK-L) (11,22,23).
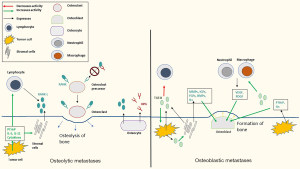
The RANK and RANK-L interaction is a major regulator of both normal and pathologic bone remodeling. RANK is a tumor necrosis factor receptor and is expressed on the surface of osteoclasts and osteoclast precursors. RANK-L is expressed by bone marrow stromal cells, osteoblasts, and osteocytes, and is secreted by activated T lymphocytes. Binding of RANK to RANK-L stimulates osteoclast differentiation, survival, and activity. Many cytokines, chemokines, and hormones induce osteolysis by increasing RANKL expression, including PTHrP, IL-5, and IL-11 produced by tumor cells (11,23,24). Osteoprotegerin (OPG), produced by osteoblasts and osteocytes, is a soluble decoy receptor of RANK-L and prevents binding of RANK and RANK-L. The ratio of RANK-L to OPG is critical for regulating osteoclast activity. Upregulated RANK-L, RANK, and OPG has been found in NSCLC cell lines and tumor tissues with bone metastases, with an increased ratio of RANK-L:OPG found in tumor tissues with bone metastases relative to those without (26). Additionally, invasion of tumor cells has been shown to be significantly enhanced by the addition of recombinant human RANK-L and transfection of RANK-L complementary DNA (cDNA). Moreover, invasion was impaired when OPG was added to these cells (26). Similarly, in a mouse model of NSCLC, human OPG-Fc reduced development of osteolytic metastases (27). Tumor cells in the bone may cause increased RANK-L expression, and RANK-L may stimulate bone metastases via binding RANK-expressing cancer cells, leading to increased invasion and migration (25).
Factors implicated in primarily osteoblastic metastases include transforming growth factor-beta (TGF-β), bone morphogenic proteins (BMPs), insulin like-growth factors (IGFs), fibroblast growth factors (FGFs), and PDGFs (23,28,37,39-42). TGF-β is a multifunctional cytokine expressed by cancer and stromal cells (28). It controls expression of multiple genes and factors promoting bone metastases, including CXCR4, MMPs (9,29,30), IL-11, vascular endothelial growth factor (VEGF), and PTHrP (29,31-33). TGF-β has also been implicated in inhibiting immune cell infiltration, allowing tumor growth (34,43). TGF-β induces production of VEGF and PDGF from immune cells, such as neutrophils and macrophages, which increase bone metastases (29). Lung cancer cells also express PTHrP, which increases both osteoblastic and osteolytic metastases (35,36). TGF-β stimulates cancer cells to produce PTHrP and stimulates stromal cells to release other bone activating cytokines (such as MMPs, IGFs, FGFs, and BMPs), leading to a vicious cycle of bone osteolysis and formation (28,33,37,38). It is likely that based on tumor specific molecular alterations, bone metastases can either be predominantly lytic with activation of RANK and RANKL pathway, or predominantly osteoblastic due to activation of TGF-β pathway.
Clinical presentation
Symptoms related to bone metastases are described as SREs. Definitions for SREs vary among studies, but include severe bone pain, pathologic fractures, spinal cord and nerve compression syndromes, bone instability, hypercalcemia, and pain or instability requiring radiation therapy (RT) or orthopedic surgery. The most common SRE is severe pain and occurs in 80% of patients with bone metastases (1,44,45). After pain, the next most commonly reported SREs are necessity for radiotherapy (50–70% of patients), pathologic fractures (7–35%), hypercalcemia (1–20%), spinal cord compression (1–15%), and necessity for surgery (0–9%) (46-48).
Multiple metastatic lesions are more common than single sites, with multiple lesions occurring in approximately 80% of patients with bone metastases (1,44,48,49). Common sites of bone metastases include the spine (40–50%), ribs (20–27%), and pelvis (17–22%) (46-48,50). Fractures are common in the proximal portion of long bones, such as the femur or rib, as well as in the vertebrae. Sudden onset back pain and neurologic symptoms should trigger concern for cord compression in patients with vertebral metastases, of which immediate neurosurgical consultation is indicated given the poor prognosis if not addressed quickly. Cord compression has been reported to occur in 15% of patients with lung cancer and bone metastases (39,51).
Hypercalcemia is the most common metabolic complication of malignancy. In lung cancer, it generally occurs from osteolysis directly induced by tumor cells or from secreted PTHrP (52). Hypercalcemia portends a poor prognosis with a median overall survival (OS) of 10–12 weeks after its development (51,53).
SREs are associated with increased morbidity and mortality and decreased quality of life (5). They occur early in the clinical course and are often present at diagnosis. Tsuya and colleagues reported that of a cohort of 259 patients with NSCLC, 70 patients (30.4%) were found to have skeletal metastases during their course, with 35 patients having SREs. Eleven of 35 patients (31%) had SREs at the initial diagnosis of NSCLC (46). Other studies have found median time to first SRE from diagnosis of bone metastasis to be between 6 and 9.5 months (mo) (1,4,47).
Imaging and diagnosis
Bone metastases may be detected on routine imaging or with directed imaging when patients present with suspicious symptoms. Radiographs have poor sensitivity for screening at 40–50% (54), and considerable bone destruction (30–75% decrease in bone density) must be present before being evident (55). Magnetic resonance imaging (MRI) has a very high sensitivity for detection and diagnosis at approximately 95% (56,57) and is the imaging modality of choice if there is concern for cord compression (58). Computed tomography (CT) may be more powerful than MRI for treatment planning and is especially useful for evaluating structural integrity. CT can detect osteolytic and osteoblastic metastases within the bone marrow before destruction is sufficient to be evident on radiograph. Bone scans are especially adept in patients with primarily osteoblastic metastases (56).
Once there is concern for bone metastases or at initial diagnosis of NSCLC, comprehensive skeletal evaluation is typically performed with fluorodeoxyglucose-positron emission tomography-CT (FDG-PET-CT). FDG-PET-CT is extremely sensitive (~98%) in the evaluation of bone metastases (56), and society guidelines recommend FDG-PET-CT to evaluate distant metastases of all sites in newly diagnosed patients with lung cancer (59,60). A CT of the chest and abdomen with contrast is recommended if FDG-PET is not available, and bone scans may be used as an alternative imaging modality to evaluate for bone metastases, although their sensitivity and specificity is lower compared to PET imaging (59-61). A definitive diagnosis is made by biopsy of the bone lesion. In many cases, however, biopsies are not obtained, especially if there is a known primary tumor with a characteristic skeletal lesion, and particularly if there are multiple lesions or if obtaining a biopsy would impose substantial risk to the patient. However, if there is any doubt for the diagnosis, biopsy should be considered. Indeed, in a study of 482 patients with a primary malignancy who underwent a biopsy of a suspicious bone lesion, 21% of the lesions were benign and 3% were due to a second malignancy (62).
Factors predicting development of bone metastases
Predicting the development and progression of bone metastases remains difficult, but accurate prediction could allow earlier intervention to prevent development of SRE. Predictive factors that have been evaluated include patient/tumor related factors, molecular alterations, and serum biomarkers.
Clinical characteristics
Stage at diagnosis, histologic subtype, and age have been shown to be predictive of bone metastases development in several studies. In a retrospective Italian cohort study of 661 deceased NSCLC patients with bone metastases, factors associated with a faster median time to development of bone metastases included age >65 (5 vs. 7 mo, P=0.046), Eastern Cooperative Oncology Group (ECOG) >2 (P=0.012), and stage IV disease at diagnosis (P=0.001) (1). On multivariate analysis of a prospective cohort study of 274 Japanese patients with stage IIIB/IV lung cancer, factors predicting bone metastases development included stage IV, ECOG >1, and increased bone alkaline phosphatase (ALP) (4). Patients with adenocarcinoma have been found to have higher risk of bone metastases relative to other histologic subtypes (63,64).
Tumor mutations
The effect of tumor mutation status on bone metastases has also been evaluated. A retrospective case-control study evaluated 189 metastatic NSCLC patients with EGFR (exon 19 and 21) mutations, KRAS mutations, and wild type EGFR/KRAS. There was no difference in incidence of bone metastases (60% vs. 52% vs. 50%), mean time to diagnosis of bone metastases in patients without bone metastases at diagnosis, or time to first SRE among the three groups of patients. As anticipated, median OS was longer in EGFR mutated patients than KRAS mutated or wild type patients (26.7 vs. 11 vs. 11.5 mo, P<0.0001) (65). Similarly, in a study of 209 patients at Colorado University with NSCLC with EGFR, KRAS, ALK, or no mutations at diagnosis, no molecular cohort was predisposed to develop to bone, brain, or lung metastases (66). However, a 2020 retrospective analysis of 570 patients with NSCLC found an increased risk of bone and lung metastases in patients with EGFR and HER2 mutations compared to patients with gene fusions, RAS mutations, or mutations without an identified oncogene driver (P<0.001) (67). In a 2014 study of 277 patients with metastatic lung adenocarcinoma at a single Japanese institution, patients with EGFR mutations had a significantly greater number of lung, brain, and bone metastases (median 3 vs. 2, P=0.035) than wild type patients. Patients with EGFR mutations had improved OS relative to wild type patients (median 28.1 vs. 14.9 mo) (68). In summary, results have been conflicting, but patients with EGFR and HER2 mutations may be at increased risk of bone metastases, albeit with improved OS in patients with EGFR mutations, likely due to the availability of effective targeted therapies for the treatment of EGFR mutated NSCLC.
Circulating tumor cells (CTCs) and DNA
CTCs and circulating tumor DNA (ctDNA) have been shown to have prognostic implications at diagnosis and evaluating treatment response in several solid tumors, including lung (69). Higher ctDNA and CTC quantities have been found in patients with bone metastases compared to those without. This likely represents increased overall tumor burden irrespective of the bone metastases themselves (70-72), but some studies have found this to be specific to bone. For example, a trial of 57 patients with EGFR mutated NSCLC found a significant correlation between bone metastases and ctDNA detection [odds ratio (OR): 3.99; 95% confidence interval (CI): 1.027–15.45; P=0.046], but not between other locations of metastases (73). Additionally, in a study of 67 lung cancer patients with bone metastases compared to 30 patients without, CTCs were more likely to be detected in the bone metastases group than in patients without (94% vs. 71%). Increased CTCs were not associated with intrapulmonary or lymph node metastasis. Of patients with CTCs detected, patients with bone metastases had a significantly higher number of CTCs than bone metastases negative patients (P=0.0045) (74). To summarize, patients with bone metastases may have increased levels of ctDNA and CTCs relative to patients without, although it is more likely related to overall tumor burden rather than the bone itself.
Serum biomarkers
Several biomarkers have also been evaluated in predicting development of bone metastases. In a case-control study of 30 patients with resected NSCLC who subsequently developed bone metastases vs. 30 patients without any metastases vs. 26 patients with non-bone metastases, 10 markers were evaluated via immunohistochemistry including MMP, VEGF, and BSP. BSP was expressed in 80% of patients with bone metastases vs. 20% of patients without metastases and in 31% of patients with non-bone metastases. Detection of BSP was strongly associated with bone dissemination (P<0.001) and independently with worse OS in all patients (14). Zhang and colleagues also found expression of BSP to be associated with bone metastases in 180 patients with completely resected NSCLC, 40 of whom later developed bone metastases (47.5% vs. 22.9%, P=0.007). Two-year bone metastases free survival was increased in patients with low BSP expression (85% vs. 69%, P=0.01) (15). Tang et al. found levels of bone ALP, Tartrate-resistant acid phosphatase 5b (TRAPC5b), and type 1 collagen carboxyterminal telopeptide to be higher in 130 lung cancer patients with bone metastases vs. 135 patients without (75). Finally, a 2017 retrospective study of 2021 patients with lung cancer at a single institution found risk factors predictive of development of bone metastases to include elevated cancer antigen-125 (CA-125) and elevated ALP (64).
Treatment of bone metastases
In general, the treatment of bone metastases is not curative; goals of management include maximizing symptom control, preserving function, minimizing SREs, and enhancing local tumor control. In addition to pain management, therapies include osteoclast inhibitors and other bone specific therapy, systemic anti-cancer therapy, radiation, surgery, and interventional techniques. A multi-disciplinary approach is required. A majority of patients will have significant pain during their course, and most will ultimately require opioids for analgesia. Other pharmacologic approaches for pain management include acetaminophen, NSAIDs, steroids, antidepressants, and gabapentin (45,76).
Bisphosphonates
Bisphosphonates are standard of care in patients with bone metastases. Bisphosphonates attach to hydroxyapatite on exposed bone around resorbing osteoclasts and are internalized by osteoclasts, causing disruption of bone resorption and possible osteoclast apoptosis (51). They are effective for both osteolytic and sclerotic lesions. In addition, they may have a direct effect on tumor cells via GTPase modulation leading to apoptosis (77) and may stimulate anti-tumor immune mechanisms (78). Bisphosphonates as a class are well-tolerated. Common side effects include flu-like symptoms and hypocalcemia; rare jaw osteonecrosis may occur. Close monitoring must be taken in patients with renal insufficiency.
The most well studied of the bisphosphonates in malignancy is zoledronic acid (ZA). There have been several studies regarding its use in bone metastases broadly and a few specific to lung cancer. A large prospective RCT by Rosen et al. in 2003 evaluated 773 patients with metastatic bone disease from solid tumors (excluding breast and prostate), of whom 378 had lung cancer. ZA delayed time to first SRE (230 vs. 163 days, P=0.023) and reduced the risk of developing SREs in all tumor types [hazard ratio (HR): 0.73; P=0.017] (79). While the data from smaller prospective studies provides conflicting results with regard to the efficacy of ZA on pain control, SRE, progression-free survival (PFS) and OS (80,81), a 2012 systematic review and meta-analysis of 12 trials of bisphosphonates in lung cancer patients with bone metastases found that patients treated with ZA and chemotherapy had significantly fewer SREs than those treated with chemotherapy alone. Patients treated with bisphosphonates in addition to another treatment modality (chemotherapy or RT) had improved pain control (82). ZA is typically dosed every 4 weeks, although longer intervals may be efficacious (83). Despite data supporting their use, bisphosphonates may not be as widely prescribed in lung cancer as other cancers, with percentages among both prospective and retrospective studies ranging from 6–60% (1,44,46,49,84). In summary, use of bisphosphonates in patients with known bone metastases may confer a modest benefit in terms of pain control, decreasing SRE, and perhaps improvement in PFS.
Denosumab
Denosumab is a monoclonal antibody against RANK-L, blocking binding to RANK and reducing formation, function, and survival of osteoclasts, leading to decreased bone resorption. It is considered safe in patients with impaired renal function, unlike bisphosphonates (85). Otherwise, its side effect profile is similar to bisphosphonates and is usually administered every 4 weeks. Denosumab was compared to ZA in a large phase three RCT of patients with solid tumors (excluding breast and prostate). Denosumab was shown to be non-inferior to ZA in delaying time to first-on-study SRE (primary endpoint). The OS and PFS were similar in both groups (86). An exploratory subgroup analysis of 811 lung cancer patients with bone metastases from this study was performed, with 411 patients receiving denosumab and 400 receiving ZA. Denosumab was associated with improved median OS in all lung cancer patients (8.9 vs. 7.7 mo in ZA; HR: 0.80; 95% CI: 0.67–0.95) and in 702 patients with NSCLC (9.5 vs. 8 mo; HR 0.78) (P=0.01 for each comparison) (87). The mechanism for potential improved OS in patients with bone metastases in this exploratory study is unclear. Denosumab inhibition of RANK-L may have a direct anti-neoplastic effect via apoptosis or anti-migration of tumor cells (88,89). Denosumab may also have additional immunomodulatory effects via disrupting the interaction between tumor cells and the bone microenvironment (87,90). Importantly, data is conflicting, with a recent open label RCT of 514 patients with metastatic NSCLC (275 with bone metastases) receiving chemotherapy plus denosumab vs. placebo showing no difference in OS in patients with or without bone metastases (91).
Radiation therapy
RT is a standard approach for symptomatic bone metastases. Short, fractionated schedules have equal effectiveness as protracted schedules (92), and pain relief typically occurs within 1–2 weeks in 50% of patients (93). A comprehensive review of radiation treatment and fractionation schedules, as well as outcomes and adverse events associated with radiotherapy, is beyond the scope of this review, and has been covered elsewhere (94-100).
Surgery and interventional techniques
Surgery is usually reserved for lesions with a complete or impending fracture, or in spinal metastases with mechanical instability or cord compression. Surgery can alleviate pain and preserve function in impending fractures in non-vertebral bones (101). However, the need for surgery portends a poor prognosis, with median OS after surgery reported to be 3 and 5.4 mo in two small retrospective analyses of NSCLC patients with operatively managed bone metastases (102,103). Presence of a fracture portended worse prognosis (3 vs. 6.3 mo median OS) (103). Most studies specific to non-spine metastases include patients with several types of malignancy, not limited to NSCLC. Two retrospective studies of patients with non-spine metastases found most patients had multiple bone metastases (vs. single metastases), with the most common location being the femur. Complete fracture was associated with worsened survival relative to those who underwent surgery for impending fracture or pain in both studies (104,105).
Surgery is indicated in patients with spinal cord compression and may be associated with improved ambulatory status compared to radiotherapy alone, as shown by a seminal 2005 study by Patchell and colleagues. In this study, 101 patients with spinal metastases (26 with lung cancer) were randomized to RT plus surgery vs. RT alone. Patients receiving surgery showed improved ambulatory rate (84% vs. 57%), maintenance of continence, functional ability, and survival (126 vs. 100 days) (106). Most studies specific to spinal metastases from NSCLC are observational. A recently published systematic review and meta-analysis of outcomes of surgery in patients with cord compression secondary to metastatic NSCLC included 11 observational cohort studies without controls. One mo mortality following surgery ranged from 1.4–10%, with reported median OS following surgery ranging from 2.1–12 mo. Improved ambulatory status following surgery ranged from 37% to 92% with a mean value of 59.5%. Improved OS and improved post-operative ambulatory status were noted in patients with fewer (1–2 vs. >2) vertebral metastases (R=0.74 for and 0.88, respectively), and fewer metastases (0 vs. unremovable) to major internal organs (R=0.82 and 0.81, respectively) (107).
Finally, numerous interventional techniques are available, including nerve blocks, spinal injections, ablation (cryo-, thermo-, or radiofrequency), and vertebroplasties (108), and several studies have shown good efficacy in pain control with these techniques (108-113). To summarize, a multidisciplinary approach must be taken for the management of bone metastases given the breadth of treatment options, and treatment should be individualized to each patient.
Predicting outcomes in patients with bone metastases
Several factors have been evaluated in prognosticating outcomes in patients with bone metastases from NSCLC. More aggressive interventions could be pursued in patients with poor prognostic factors.
Clinical characteristics
The presence of bone metastases is associated with worse survival and patients with SREs tend to do poorly. The median survival from diagnosis of bone metastases has been reported to be 6–7 mo in patients with lung cancer (51,53). In a Denmark cohort study, 1-year survival of lung cancer patients without bone metastases was 37% vs. 12.1% with bone metastases and 5.1% in patients with bone metastases and SRE (114). The presence of multiple bone metastases may portend a worse prognosis than single metastases. In a retrospective study by Sugiura et al. published in 2008, presence of multiple bone metastases was associated with significantly shorter OS than patients with single metastases (8.9 vs. 14 mo, P=0.02). Presence of fractures was also associated with worse OS compared to no fractures (6.4 vs. 10.2 mo, P=0.04) (48). In a retrospective cohort study of 661 deceased NSCLC patients with bone metastases, median OS after diagnosis of bone metastases was 9.5 and 7 mo after first SRE. Presence of more than 2 of 4 factors (age >65, non-adenocarcinoma histology, EGOG >2, presence of visceral and bone metastases at diagnosis) was associated with worse OS from diagnosis of bone metastases (5 vs. 8 mo, P<0.001). Lack of bisphosphonate use was also associated with shorter OS (1). Studies comparing survival between patients with metastatic NSCLC involving different organs have found mixed results. However, in general, these studies suggest a relatively worse prognosis in patients with liver metastases (115-117), followed by bone and brain metastases (118-120).
Tumor mutations
In general, NSCLC patients with driver mutations are known to have improved outcomes compared to those without (121), and this has been found in patients with bone metastases (65,122,123). For example, a Japanese study of 125 patients with NSCLC with bone metastases showed patients with EGFR mutations had significantly lower incidence of SRE (21% vs. 38%, P<0.05) and longer time to first SRE (13 vs. 6 mo, P<0.05) when treated with a tyrosine kinase inhibitor compared to EGFR mutation negative patients, treated mostly with chemotherapy (123). Presence of bone metastases was a significant predictor of OS in EGFR mutated (HR: 2.04; 95% CI: 1.17–3.64; P=0.011) and wild type (HR: 2.15; 95% CI: 1.40–3.29; P<0.001) patients, in a study of 277 Japanese patients with metastatic lung adenocarcinoma. On subgroup analysis of 116 patients with bone metastases however, the authors found no significant difference in OS in patients with vs. without SRE in both wild type and EGFR mutated patients (68).
Serum biomarkers
N-telopeptide of type 1 collagen (NTX) has been identified as a marker of bone metastases progression. In a retrospective analysis, high vs. normal urinary NTX correlated with a greater than two-fold increased risk of bone lesion progression and death in the placebo group of a phase three prospective RCT comparing ZA to placebo in NSCLC patients with bone metastases. Additionally, ZA reduced the relative risk of death by 35% in patients with higher baseline NTX levels compared to those with high levels receiving placebo (124). TRAPC5b is another marker of bone resorption. In a study of 141 newly diagnosed patients with stage III or IV NSCLC (72 with bone metastases), TRAPC5b activity was higher in patients with bone metastases than without (3.50±2.23 U/L, 2.09±0.72 U/L, P<0.001). A decline in TRACP5b was associated with response to treatment in patients with bone metastases (P=0.047), whereas an increase was associated with new development of bone metastases (P=0.05) (125). In a study of urinary n-telopeptide and bone ALP in 441 patients with several types of cancer (115 with NSCLC) who had bone metastases, high levels of N-telopeptide (>100 nmol/mmol creatinine) was associated with increased relative risk of death compared to patients with NSCLC who had low levels of N-telopeptide [risk ratio (RR): 3.51; 95% CI: 2.13–5.79; P<0.001] (126).
Response to chemotherapy and targeted therapy
Predicting response to therapy is critical in metastatic NSCLC. Bone metastases have been considered “unmeasurable” by the Response Evaluation Criteria in Solid Tumors (RECIST) criteria, secondary to difficulty determining response to treatment (127,128). In 2004, Hamaoka et al. established the “MD Anderson criteria” (Table 2) for bone metastases response to therapy, but this is not widely used in clinical practice (129,130). Osteosclerotic changes, considered to be a repair process of bone, appear as changes in attenuation on CT and have also been evaluated in determining response (131).
Table 2
Response type | Definition |
---|---|
Complete response | Complete fill-in or sclerosis of lytic lesion on CT or XR |
Disappearance of tumor signal on bone scan, CT, or MRI | |
Normalization of osteoblastic lesion on CT or XR | |
Partial response | Sclerotic rim formation around initially lytic lesion on CT |
Sclerosis of lesions previously undetected on CT or XR | |
Partial fill-in or sclerosis of lytic lesion on CT or XR | |
Regression of measurable lesion on CT, MRI, or XR | |
Regression of lesion on bone scan | |
Decrease in blastic lesion on CT or XR | |
Stable disease | No change in measurable lesion on CT, MRI, or XR |
No change in blastic or lytic lesion or CT, MRI, or XR | |
No new lesion on CT, MRI, or XR | |
Progressive disease | Increase in size of existing measurable lesion on CT, MRI, or XR |
New lesion on CT, MRI, bone scan, or XR | |
Increase in lytic or blastic lesion on CT or XR |
Ct, computed tomography; xr, plain radiography; Mri, magnetic resonance imaging. Adopted with publisher permission from (129).
Few trials have specifically evaluated presence of bone metastases in response to therapy, and most have been of small sample sizes. One retrospective study of 25 patients with NSCLC with bone metastases treated with first line platinum-based chemotherapy with or without bevacizumab found improved bone specific response (23 vs. 0%, P=0.038), disease control, and median time to bone progression (13.7 vs. 4.3 mo, P=0.06) in patients treated with bevacizumab vs. without. OS was similar in both groups (132). A retrospective study of 52 NSCLC patients with bone metastases receiving platinum-based combination chemotherapy found patients with osteosclerotic changes on CT had significantly higher disease control rate (100% vs. 64.7% at 3 mo, P<0.001) and improved 1-year PFS than patients without osteosclerotic changes (1-year PFS: 74.9% vs. 30.2%, P<0.001) (133). A similarly improved response and OS in 41 lung adenocarcinoma patients treated with gefitinib was found in patients with osteosclerotic lesions vs. those without in a study by Yamashita et al. (134).
Response to immune checkpoint inhibitors
The bone is a hematopoietic organ, plays an active role in regulating the immune system, and may influence response to immunotherapy (135). However, there are relatively few trials dedicated to evaluating immunotherapy in bone metastases in NSCLC. An Italian cohort study by Landi et al. (6) of previously treated NSCLC patients receiving nivolumab evaluated the role of bone metastases in immunotherapy. Cohort A included 1,588 patients with non-squamous disease (39% with bone metastases) and cohort B included 371 patients with squamous cell disease (32% with bone metastases). In both cohorts, patients with bone metastases had a lower overall response rate based on RECIST (A: 12% with bone metastases vs. 23% without, P<0.0001; B: 13% vs. 22%, P<0.04), shorter PFS (A: 3 vs. 4 mo, P<0.0001; B: 2.7 vs. 5.2 mo, P<0.0001), and shorter OS (A: 7.4 vs. 15.3 mo, P<0.0001; B: 5.0 vs. 10.9 mo, P<0.0001) compared to those without bone metastases treated with nivolumab. Presence of bone metastases negatively affected outcome regardless of performance status or presence of liver metastases and was independently associated with risk of death on multivariate analysis (A: HR: 1.5; B: HR: 1.78). In both groups, there was an increased risk of death and progression within 3 mo in patients with bone metastases. Importantly, there was no info on programmed death ligand-1 (PD-L1) status in this trial, nor a control arm without immunotherapy (6).
CheckMate 227 was a RCT that evaluated nivolumab with ipilimumab vs. nivolumab with chemotherapy vs. chemotherapy alone in metastatic NSCLC patients who had not received prior chemotherapy. Regardless of whether patients received ipilimumab and nivolumab or chemotherapy, presence of bone metastases was associated with worse OS (12.2 vs. 19.2 mo in ipilimumab/nivolumab group; 8.8 vs. 16.0 mo in chemotherapy group). There was improved OS in patients with bone metastases treated with ipilimumab/nivolumab vs. chemo in patients of all PD-L1 status [12.2 vs. 8.8 mo; HR: 0.68 (0.53–0.88)] and in those with PD-L1 expression <1% [9.5 vs. 7.6 mo; HR: 0.58 (0.37–0.89)], but not with PD-L1 expression >1% [13.4 vs. 10.0 mo; HR: 0.75 (0.55–1.03)] (136). Conversely, survival analysis of the CheckMate 057 study of nivolumab vs. docetaxel as second line therapy in NSCLC evaluated 161 patients with bone metastases, 86 receiving nivolumab and 75 docetaxel. At 3 mo, 26 of 86 patients in the nivolumab had died vs. 11 of 75 patients receiving docetaxel (P=0.019), suggesting immunotherapy may not overcome the poor overall prognosis of skeletal metastasis (137). Additionally, a retrospective study of 330 patients with metastatic NSCLC who received immune checkpoint inhibitors found significantly worse OS in patients with bone metastases than those without (5.9 vs. 13.4 mo, P<0.001). Patients with baseline bone metastases had a higher HR of death than those without when controlling for performance status, histology, line of therapy, and burden of disease (HR: 1.57; 95% CI: 1.19–2.08; P=0.001) (138). Conversely, a retrospective review of 201 patients with metastatic NSCLC receiving nivolumab at three Japanese centers found liver and lung metastases, but not bone, to be predictive of shorter median PFS (139). Finally, a small retrospective review of 15 NSCLC patients with bone metastases who received single agent nivolumab evaluated response of bone metastases using the MD Anderson response criteria to predict overall outcomes. Non-responders based on the MD Anderson criteria had earlier disease progression based on RECIST criteria compared to responders. Additionally, in responders according to both RECIST and MD Anderson criteria, time to response was earlier with the MD Anderson criteria (1.4–2 mo) than the RECIST criteria (2.8–3 mo) (140), suggesting the MD Anderson criteria could be used in future larger studies to evaluate the response of bone metastases.
Conclusions
Bone metastases in lung cancer patients remain a common occurrence, impacting morbidity, mortality, and quality of life. Patients with SREs have worse prognosis. There is data supporting use of bisphosphonates and/or denosumab, and these should be considered in all patients with bone metastases. Molecular profiling should be evaluated as a potential biomarker for risk of development of bone metastases and response to treatment. Additionally, next generation sequencing has revealed a high degree of heterogeneity among all tumors (141). Multiple subclones derived from the primary tumor may behave in different phenotypes and determining the role of driver mutations driving skeletal metastases could help identify patients at risk of developing bone metastases (142). Novel studies comparing the genomic alterations of skeletal metastases and primary tumors are needed. As therapy for patients with advanced disease evolves, more studies are needed to evaluate the interplay between immunotherapy and bone metastases, and determining the response to treatment in bone. One such trial, DENIVOS (NCT03669523), evaluating combination therapy with denosumab and nivolumab as a second-line treatment in patients with NSCLC and bone metastases, is currently ongoing. Future RCTs of immunotherapy should include data on presence of bone metastases, and dedicated trials regarding bone metastases and immunotherapy should be performed.
Acknowledgments
Funding: None.
Footnote
Reporting Checklist: The authors have completed the Narrative Review reporting checklist. Available at https://jtd.amegroups.com/article/view/10.21037/jtd-21-1502/rc
Peer Review File: Available at https://jtd.amegroups.com/article/view/10.21037/jtd-21-1502/prf
Conflicts of Interest: All authors have completed the ICMJE uniform disclosure form (available at https://jtd.amegroups.com/article/view/10.21037/jtd-21-1502/coif). The authors have no conflicts of interest to declare.
Ethical Statement: The authors are accountable for all aspects of the work in ensuring that questions related to the accuracy or integrity of any part of the work are appropriately investigated and resolved.
Open Access Statement: This is an Open Access article distributed in accordance with the Creative Commons Attribution-NonCommercial-NoDerivs 4.0 International License (CC BY-NC-ND 4.0), which permits the non-commercial replication and distribution of the article with the strict proviso that no changes or edits are made and the original work is properly cited (including links to both the formal publication through the relevant DOI and the license). See: https://creativecommons.org/licenses/by-nc-nd/4.0/.
References
- Santini D, Barni S, Intagliata S, et al. Natural History of Non-Small-Cell Lung Cancer with Bone Metastases. Sci Rep 2015;5:18670. [Crossref] [PubMed]
- Toloza EM, Harpole L, McCrory DC. Noninvasive staging of non-small cell lung cancer: a review of the current evidence. Chest 2003;123:137S-46S. [Crossref] [PubMed]
- Riihimäki M, Hemminki A, Fallah M, et al. Metastatic sites and survival in lung cancer. Lung Cancer 2014;86:78-84. [Crossref] [PubMed]
- Katakami N, Kunikane H, Takeda K, et al. Prospective study on the incidence of bone metastasis (BM) and skeletal-related events (SREs) in patients (pts) with stage IIIB and IV lung cancer-CSP-HOR 13. J Thorac Oncol 2014;9:231-8. [Crossref] [PubMed]
- Saad F, Lipton A, Cook R, et al. Pathologic fractures correlate with reduced survival in patients with malignant bone disease. Cancer 2007;110:1860-7. [Crossref] [PubMed]
- Landi L, D'Incà F, Gelibter A, et al. Bone metastases and immunotherapy in patients with advanced non-small-cell lung cancer. J Immunother Cancer 2019;7:316. [Crossref] [PubMed]
- Sulzer MA, Leers MP, van Noord JA, et al. Reduced E-cadherin expression is associated with increased lymph node metastasis and unfavorable prognosis in non-small cell lung cancer. Am J Respir Crit Care Med 1998;157:1319-23. [Crossref] [PubMed]
- Onder TT, Gupta PB, Mani SA, et al. Loss of E-cadherin promotes metastasis via multiple downstream transcriptional pathways. Cancer Res 2008;68:3645-54. [Crossref] [PubMed]
- Hofmann HS, Hansen G, Richter G, et al. Matrix metalloproteinase-12 expression correlates with local recurrence and metastatic disease in non-small cell lung cancer patients. Clin Cancer Res 2005;11:1086-92. [PubMed]
- Liotta LA, Kohn E. Cancer invasion and metastases. JAMA 1990;263:1123-6. [Crossref] [PubMed]
- Mundy GR. Metastasis to bone: causes, consequences and therapeutic opportunities. Nat Rev Cancer 2002;2:584-93. [Crossref] [PubMed]
- Phillips RJ, Burdick MD, Lutz M, et al. The stromal derived factor-1/CXCL12-CXC chemokine receptor 4 biological axis in non-small cell lung cancer metastases. Am J Respir Crit Care Med 2003;167:1676-86. [Crossref] [PubMed]
- Müller A, Homey B, Soto H, et al. Involvement of chemokine receptors in breast cancer metastasis. Nature 2001;410:50-6. [Crossref] [PubMed]
- Papotti M, Kalebic T, Volante M, et al. Bone sialoprotein is predictive of bone metastases in resectable non-small-cell lung cancer: a retrospective case-control study. J Clin Oncol 2006;24:4818-24. [Crossref] [PubMed]
- Zhang L, Hou X, Lu S, et al. Predictive significance of bone sialoprotein and osteopontin for bone metastases in resected Chinese non-small-cell lung cancer patients: a large cohort retrospective study. Lung Cancer 2010;67:114-9. [Crossref] [PubMed]
- Yoneda T, Hiraga T. Crosstalk between cancer cells and bone microenvironment in bone metastasis. Biochem Biophys Res Commun 2005;328:679-87. [Crossref] [PubMed]
- Sung V, Stubbs JT 3rd, Fisher L, et al. Bone sialoprotein supports breast cancer cell adhesion proliferation and migration through differential usage of the alpha(v)beta3 and alpha(v)beta5 integrins. J Cell Physiol 1998;176:482-94. [Crossref] [PubMed]
- Kilvaer TK, Rakaee M, Hellevik T, et al. Differential prognostic impact of platelet-derived growth factor receptor expression in NSCLC. Sci Rep 2019;9:10163. [Crossref] [PubMed]
- Catena R, Luis-Ravelo D, Antón I, et al. PDGFR signaling blockade in marrow stroma impairs lung cancer bone metastasis. Cancer Res 2011;71:164-74. [Crossref] [PubMed]
- Gadiya M, Chakraborty G. Signaling by discoidin domain receptor 1 in cancer metastasis. Cell Adh Migr 2018;12:315-23. [Crossref] [PubMed]
- Valencia K, Ormazábal C, Zandueta C, et al. Inhibition of collagen receptor discoidin domain receptor-1 (DDR1) reduces cell survival, homing, and colonization in lung cancer bone metastasis. Clin Cancer Res 2012;18:969-80. [Crossref] [PubMed]
- Southby J, Kissin MW, Danks JA, et al. Immunohistochemical localization of parathyroid hormone-related protein in human breast cancer. Cancer Res 1990;50:7710-6. [PubMed]
- Roodman GD. Mechanisms of bone metastasis. Discov Med 2004;4:144-8. [PubMed]
- Park JH, Lee NK, Lee SY. Current Understanding of RANK Signaling in Osteoclast Differentiation and Maturation. Mol Cells 2017;40:706-13. [PubMed]
- Dougall WC, Holen I, González Suárez E. Targeting RANKL in metastasis. Bonekey Rep 2014;3:519. [Crossref] [PubMed]
- Peng X, Guo W, Ren T, et al. Differential expression of the RANKL/RANK/OPG system is associated with bone metastasis in human non-small cell lung cancer. PLoS One 2013;8:e58361. [Crossref] [PubMed]
- Miller RE, Jones JC, Tometsko M, et al. RANKL inhibition blocks osteolytic lesions and reduces skeletal tumor burden in models of non-small-cell lung cancer bone metastases. J Thorac Oncol 2014;9:345-54. [Crossref] [PubMed]
- Rieunier G, Wu X, Macaulay VM, et al. Bad to the Bone: The Role of the Insulin-Like Growth Factor Axis in Osseous Metastasis. Clin Cancer Res 2019;25:3479-85. [Crossref] [PubMed]
- Krstic J, Santibanez JF. Transforming growth factor-beta and matrix metalloproteinases: functional interactions in tumor stroma-infiltrating myeloid cells. ScientificWorldJournal 2014;2014:521754. [Crossref] [PubMed]
- Moore-Smith LD, Isayeva T, Lee JH, et al. Silencing of TGF-β1 in tumor cells impacts MMP-9 in tumor microenvironment. Sci Rep 2017;7:8678. [Crossref] [PubMed]
- Pickup M, Novitskiy S, Moses HL. The roles of TGFβ in the tumour microenvironment. Nat Rev Cancer 2013;13:788-99. [Crossref] [PubMed]
- Dunn LK, Mohammad KS, Fournier PG, et al. Hypoxia and TGF-beta drive breast cancer bone metastases through parallel signaling pathways in tumor cells and the bone microenvironment. PLoS One 2009;4:e6896. [Crossref] [PubMed]
- Vicent S, Luis-Ravelo D, Antón I, et al. A novel lung cancer signature mediates metastatic bone colonization by a dual mechanism. Cancer Res 2008;68:2275-85. [Crossref] [PubMed]
- Liu Q, Zhang C, Sun A, et al. Tumor-educated CD11bhighIalow regulatory dendritic cells suppress T cell response through arginase I. J Immunol 2009;182:6207-16. [Crossref] [PubMed]
- Liao J, McCauley LK. Skeletal metastasis: Established and emerging roles of parathyroid hormone related protein (PTHrP). Cancer Metastasis Rev 2006;25:559-71. [Crossref] [PubMed]
- Soki FN, Park SI, McCauley LK. The multifaceted actions of PTHrP in skeletal metastasis. Future Oncol 2012;8:803-17. [Crossref] [PubMed]
- Weilbaecher KN, Guise TA, McCauley LK. Cancer to bone: a fatal attraction. Nat Rev Cancer 2011;11:411-25. [Crossref] [PubMed]
- Padua D, Massagué J. Roles of TGFbeta in metastasis. Cell Res 2009;19:89-102. [Crossref] [PubMed]
- Coleman RE. Metastatic bone disease: clinical features, pathophysiology and treatment strategies. Cancer Treat Rev 2001;27:165-76. [Crossref] [PubMed]
- Nelson JB, Hedican SP, George DJ, et al. Identification of endothelin-1 in the pathophysiology of metastatic adenocarcinoma of the prostate. Nat Med 1995;1:944-9. [Crossref] [PubMed]
- Labanca E, Vazquez ES, Corn PG, et al. Fibroblast growth factors signaling in bone metastasis. Endocr Relat Cancer 2020;27:R255-65. [Crossref] [PubMed]
- Han Y, You X, Xing W, et al. Paracrine and endocrine actions of bone-the functions of secretory proteins from osteoblasts, osteocytes, and osteoclasts. Bone Res 2018;6:16. [Crossref] [PubMed]
- Popper HH. Progression and metastasis of lung cancer. Cancer Metastasis Rev 2016;35:75-91. [Crossref] [PubMed]
- Decroisette C, Monnet I, Berard H, et al. Epidemiology and treatment costs of bone metastases from lung cancer: a French prospective, observational, multicenter study (GFPC 0601). J Thorac Oncol 2011;6:576-82. [Crossref] [PubMed]
- Urch C. The pathophysiology of cancer-induced bone pain: current understanding. Palliat Med 2004;18:267-74. [Crossref] [PubMed]
- Tsuya A, Kurata T, Tamura K, et al. Skeletal metastases in non-small cell lung cancer: a retrospective study. Lung Cancer 2007;57:229-32. [Crossref] [PubMed]
- Sun JM, Ahn JS, Lee S, et al. Predictors of skeletal-related events in non-small cell lung cancer patients with bone metastases. Lung Cancer 2011;71:89-93. [Crossref] [PubMed]
- Sugiura H, Yamada K, Sugiura T, et al. Predictors of survival in patients with bone metastasis of lung cancer. Clin Orthop Relat Res 2008;466:729-36. [Crossref] [PubMed]
- Kuchuk M, Addison CL, Clemons M, et al. Incidence and consequences of bone metastases in lung cancer patients. J Bone Oncol 2013;2:22-9. [Crossref] [PubMed]
- Choi J, Raghavan M. Diagnostic imaging and image-guided therapy of skeletal metastases. Cancer Control 2012;19:102-12. [Crossref] [PubMed]
- Selvaggi G, Scagliotti GV. Management of bone metastases in cancer: a review. Crit Rev Oncol Hematol 2005;56:365-78. [Crossref] [PubMed]
- Maisano R, Pergolizzi S, Cascinu S. Novel therapeutic approaches to cancer patients with bone metastasis. Crit Rev Oncol Hematol 2001;40:239-50. [Crossref] [PubMed]
- Macedo F, Ladeira K, Pinho F, et al. Bone Metastases: An Overview. Oncol Rev 2017;11:321. [PubMed]
- Muindi J, Coombes RC, Golding S, et al. The role of computed tomography in the detection of bone metastases in breast cancer patients. Br J Radiol 1983;56:233-6. [Crossref] [PubMed]
- Even-Sapir E. Imaging of malignant bone involvement by morphologic, scintigraphic, and hybrid modalities. J Nucl Med 2005;46:1356-67. [PubMed]
- O'Sullivan GJ, Carty FL, Cronin CG. Imaging of bone metastasis: An update. World J Radiol 2015;7:202-11. [Crossref] [PubMed]
- Roberts CC, Daffner RH, Weissman BN, et al. ACR appropriateness criteria on metastatic bone disease. J Am Coll Radiol 2010;7:400-9. Erratum in: J Am Coll Radiol 2010;7:e1. [Crossref] [PubMed]
- Liu T, Wang S, Liu H, et al. Detection of vertebral metastases: a meta-analysis comparing MRI, CT, PET, BS and BS with SPECT. J Cancer Res Clin Oncol 2017;143:457-65. [Crossref] [PubMed]
- Ettinger DS, Wood DE, Aisner DL, et al. NCCN Guidelines Insights: Non-Small Cell Lung Cancer, Version 2.2021. J Natl Compr Canc Netw 2021;19:254-66. [Crossref] [PubMed]
- Silvestri GA, Gonzalez AV, Jantz MA, et al. Methods for staging non-small cell lung cancer: Diagnosis and management of lung cancer, 3rd ed: American College of Chest Physicians evidence-based clinical practice guidelines. Chest 2013;143:e211S-50S.
- Expert Panel on Thoracic Imaging. ACR Appropriateness Criteria® Noninvasive Clinical Staging of Primary Lung Cancer. J Am Coll Radiol 2019;16:S184-95. [Crossref] [PubMed]
- Raphael B, Hwang S, Lefkowitz RA, et al. Biopsy of suspicious bone lesions in patients with a single known malignancy: prevalence of a second malignancy. AJR Am J Roentgenol 2013;201:1309-14. [Crossref] [PubMed]
- Oliveira MB, Mello FC, Paschoal ME. The relationship between lung cancer histology and the clinicopathological characteristics of bone metastases. Lung Cancer 2016;96:19-24. [Crossref] [PubMed]
- Zhou Y, Yu QF, Peng AF, et al. The risk factors of bone metastases in patients with lung cancer. Sci Rep 2017;7:8970. [Crossref] [PubMed]
- Hendriks LE, Smit EF, Vosse BA, et al. EGFR mutated non-small cell lung cancer patients: more prone to development of bone and brain metastases? Lung Cancer 2014;84:86-91. [Crossref] [PubMed]
- Doebele RC, Lu X, Sumey C, et al. Oncogene status predicts patterns of metastatic spread in treatment-naive nonsmall cell lung cancer. Cancer 2012;118:4502-11. [Crossref] [PubMed]
- Patil T, Mushtaq R, Marsh S, et al. Clinicopathologic Characteristics, Treatment Outcomes, and Acquired Resistance Patterns of Atypical EGFR Mutations and HER2 Alterations in Stage IV Non-Small-Cell Lung Cancer. Clin Lung Cancer 2020;21:e191-204. [Crossref] [PubMed]
- Fujimoto D, Ueda H, Shimizu R, et al. Features and prognostic impact of distant metastasis in patients with stage IV lung adenocarcinoma harboring EGFR mutations: importance of bone metastasis. Clin Exp Metastasis 2014;31:543-51. [Crossref] [PubMed]
- Moding EJ, Liu Y, Nabet BY, et al. Circulating Tumor DNA Dynamics Predict Benefit from Consolidation Immunotherapy in Locally Advanced Non-Small Cell Lung Cancer. Nat Cancer 2020;1:176-83. [Crossref] [PubMed]
- Pécuchet N, Zonta E, Didelot A, et al. Base-Position Error Rate Analysis of Next-Generation Sequencing Applied to Circulating Tumor DNA in Non-Small Cell Lung Cancer: A Prospective Study. PLoS Med 2016;13:e1002199. [Crossref] [PubMed]
- Jia J, Huang B, Zhuang Z, et al. Circulating tumor DNA as prognostic markers for late stage NSCLC with bone metastasis. Int J Biol Markers 2018;33:222-30. [Crossref] [PubMed]
- Ikeda S, Schwaederle M, Mohindra M, et al. MET alterations detected in blood-derived circulating tumor DNA correlate with bone metastases and poor prognosis. J Hematol Oncol 2018;11:76. [Crossref] [PubMed]
- Lee Y, Park S, Kim WS, et al. Correlation between progression-free survival, tumor burden, and circulating tumor DNA in the initial diagnosis of advanced-stage EGFR-mutated non-small cell lung cancer. Thorac Cancer 2018;9:1104-10. [Crossref] [PubMed]
- Cheng M, Liu L, Yang HS, et al. Circulating tumor cells are associated with bone metastasis of lung cancer. Asian Pac J Cancer Prev 2014;15:6369-74. [Crossref] [PubMed]
- Tang C, Liu Y, Qin H, et al. Clinical significance of serum BAP, TRACP 5b and ICTP as bone metabolic markers for bone metastasis screening in lung cancer patients. Clin Chim Acta 2013;426:102-7. [Crossref] [PubMed]
- Buga S, Sarria JE. The management of pain in metastatic bone disease. Cancer Control 2012;19:154-66. [Crossref] [PubMed]
- Iguchi K. Effect of bisphosphonates on anticancer activity in prostate cancer cells. Yakugaku Zasshi 2012;132:1025-30. [Crossref] [PubMed]
- Aapro M, Saad F. Bone-modifying agents in the treatment of bone metastases in patients with advanced genitourinary malignancies: a focus on zoledronic acid. Ther Adv Urol 2012;4:85-101. [Crossref] [PubMed]
- Rosen LS, Gordon D, Tchekmedyian S, et al. Zoledronic acid versus placebo in the treatment of skeletal metastases in patients with lung cancer and other solid tumors: a phase III, double-blind, randomized trial--the Zoledronic Acid Lung Cancer and Other Solid Tumors Study Group. J Clin Oncol 2003;21:3150-7. [Crossref] [PubMed]
- Zarogoulidis K, Boutsikou E, Zarogoulidis P, et al. The impact of zoledronic acid therapy in survival of lung cancer patients with bone metastasis. Int J Cancer 2009;125:1705-9. [Crossref] [PubMed]
- Pandya KJ, Gajra A, Warsi GM, et al. Multicenter, randomized, phase 2 study of zoledronic acid in combination with docetaxel and carboplatin in patients with unresectable stage IIIB or stage IV non-small cell lung cancer. Lung Cancer 2010;67:330-8. [Crossref] [PubMed]
- Lopez-Olivo MA, Shah NA, Pratt G, et al. Bisphosphonates in the treatment of patients with lung cancer and metastatic bone disease: a systematic review and meta-analysis. Support Care Cancer 2012;20:2985-98. [Crossref] [PubMed]
- Tam AH, Schepers AJ, Qin A, et al. Impact of Extended-Interval Versus Standard Dosing of Zoledronic Acid on Skeletal Events in Non-Small-Cell Lung Cancer and Small-Cell Lung Cancer Patients With Bone Metastases. Ann Pharmacother 2021;55:697-704. [Crossref] [PubMed]
- Hatoum HT, Lin S, Lipton A, et al. The impact of zoledronic acid treatment on frequency of skeletal complications experienced and on followup duration post diagnosis of bone metastasis in lung cancer patients. J Clin Oncol 2008;26:abstr 8106.
- Yee AJ, Raje NS. Denosumab, a RANK ligand inhibitor, for the management of bone loss in cancer patients. Clin Interv Aging 2012;7:331-8. [PubMed]
- Henry DH, Costa L, Goldwasser F, et al. Randomized, double-blind study of denosumab versus zoledronic acid in the treatment of bone metastases in patients with advanced cancer (excluding breast and prostate cancer) or multiple myeloma. J Clin Oncol 2011;29:1125-32. [Crossref] [PubMed]
- Scagliotti GV, Hirsh V, Siena S, et al. Overall survival improvement in patients with lung cancer and bone metastases treated with denosumab versus zoledronic acid: subgroup analysis from a randomized phase 3 study. J Thorac Oncol 2012;7:1823-9. [Crossref] [PubMed]
- Jones DH, Nakashima T, Sanchez OH, et al. Regulation of cancer cell migration and bone metastasis by RANKL. Nature 2006;440:692-6. [Crossref] [PubMed]
- De Castro J, García R, Garrido P, et al. Therapeutic Potential of Denosumab in Patients With Lung Cancer: Beyond Prevention of Skeletal Complications. Clin Lung Cancer 2015;16:431-46. [Crossref] [PubMed]
- Ahern E, Smyth MJ, Dougall WC, et al. Roles of the RANKL-RANK axis in antitumour immunity - implications for therapy. Nat Rev Clin Oncol 2018;15:676-93. [Crossref] [PubMed]
- Peters S, Danson S, Hasan B, et al. A Randomized Open-Label Phase III Trial Evaluating the Addition of Denosumab to Standard First-Line Treatment in Advanced NSCLC: The European Thoracic Oncology Platform (ETOP) and European Organisation for Research and Treatment of Cancer (EORTC) SPLENDOUR Trial. J Thorac Oncol 2020;15:1647-56. [Crossref] [PubMed]
- Hartsell WF, Scott CB, Bruner DW, et al. Randomized trial of short- versus long-course radiotherapy for palliation of painful bone metastases. J Natl Cancer Inst 2005;97:798-804. [Crossref] [PubMed]
- Coleman R, Body JJ, Aapro M, et al. Bone health in cancer patients: ESMO Clinical Practice Guidelines. Ann Oncol 2014;25:iii124-37. [Crossref] [PubMed]
- Chow E, Harris K, Fan G, et al. Palliative radiotherapy trials for bone metastases: a systematic review. J Clin Oncol 2007;25:1423-36. [Crossref] [PubMed]
- Chow R, Hoskin P, Schild SE, et al. Single vs multiple fraction palliative radiation therapy for bone metastases: Cumulative meta-analysis. Radiother Oncol 2019;141:56-61. [Crossref] [PubMed]
- Lehrer EJ, Singh R, Wang M, et al. Safety and Survival Rates Associated With Ablative Stereotactic Radiotherapy for Patients With Oligometastatic Cancer: A Systematic Review and Meta-analysis. JAMA Oncol 2021;7:92-106. [Crossref] [PubMed]
- Spencer KL, van der Velden JM, Wong E, et al. Systematic Review of the Role of Stereotactic Radiotherapy for Bone Metastases. J Natl Cancer Inst 2019;111:1023-32. [Crossref] [PubMed]
- Rich SE, Chow R, Raman S, et al. Update of the systematic review of palliative radiation therapy fractionation for bone metastases. Radiother Oncol 2018;126:547-57. [Crossref] [PubMed]
- Chow R, Hoskin P, Chan S, et al. Efficacy of multiple fraction conventional radiation therapy for painful uncomplicated bone metastases: A systematic review. Radiother Oncol 2017;122:323-31. [Crossref] [PubMed]
- Lutz S, Balboni T, Jones J, et al. Palliative radiation therapy for bone metastases: Update of an ASTRO Evidence-Based Guideline. Pract Radiat Oncol 2017;7:4-12. [Crossref] [PubMed]
- Wood TJ, Racano A, Yeung H, et al. Surgical management of bone metastases: quality of evidence and systematic review. Ann Surg Oncol 2014;21:4081-9. [Crossref] [PubMed]
- Weiss RJ, Wedin R. Surgery for skeletal metastases in lung cancer. Acta Orthop 2011;82:96-101. [Crossref] [PubMed]
- Utzschneider S, Wicherek E, Weber P, et al. Surgical treatment of bone metastases in patients with lung cancer. Int Orthop 2011;35:731-6. [Crossref] [PubMed]
- Ratasvuori M, Wedin R, Keller J, et al. Insight opinion to surgically treated metastatic bone disease: Scandinavian Sarcoma Group Skeletal Metastasis Registry report of 1195 operated skeletal metastasis. Surg Oncol 2013;22:132-8. [Crossref] [PubMed]
- Bonnevialle P, Baron-Trocellier T, Niglis L, et al. Functional results and survival after surgery for peripheral skeletal metastasis: A 434-case multicenter retrospective series. Orthop Traumatol Surg Res 2020;106:997-1003. [Crossref] [PubMed]
- Patchell RA, Tibbs PA, Regine WF, et al. Direct decompressive surgical resection in the treatment of spinal cord compression caused by metastatic cancer: a randomised trial. Lancet 2005;366:643-8. [Crossref] [PubMed]
- Hsieh MK, Bowles DR, Canseco JA, et al. Is Open Surgery for Metastatic Spinal Cord Compression Secondary to Lung Cancer Really Beneficial? A Systematic Review. World Neurosurg 2020;144:e253-63. [Crossref] [PubMed]
- Hochberg U, Elgueta MF, Perez J. Interventional Analgesic Management of Lung Cancer Pain. Front Oncol 2017;7:17. [Crossref] [PubMed]
- Health Quality Ontario. Vertebral Augmentation Involving Vertebroplasty or Kyphoplasty for Cancer-Related Vertebral Compression Fractures: A Systematic Review. Ont Health Technol Assess Ser 2016;16:1-202.
- Dupuy DE, Liu D, Hartfeil D, et al. Percutaneous radiofrequency ablation of painful osseous metastases: a multicenter American College of Radiology Imaging Network trial. Cancer 2010;116:989-97. [Crossref] [PubMed]
- Goetz MP, Callstrom MR, Charboneau JW, et al. Percutaneous image-guided radiofrequency ablation of painful metastases involving bone: a multicenter study. J Clin Oncol 2004;22:300-6. [Crossref] [PubMed]
- Callstrom MR, Dupuy DE, Solomon SB, et al. Percutaneous image-guided cryoablation of painful metastases involving bone: multicenter trial. Cancer 2013;119:1033-41. [Crossref] [PubMed]
- Callstrom MR, Charboneau JW, Goetz MP, et al. Image-guided ablation of painful metastatic bone tumors: a new and effective approach to a difficult problem. Skeletal Radiol 2006;35:1-15. [Crossref] [PubMed]
- Cetin K, Christiansen CF, Jacobsen JB, et al. Bone metastasis, skeletal-related events, and mortality in lung cancer patients: a Danish population-based cohort study. Lung Cancer 2014;86:247-54. [Crossref] [PubMed]
- Ren Y, Dai C, Zheng H, et al. Prognostic effect of liver metastasis in lung cancer patients with distant metastasis. Oncotarget 2016;7:53245-53. [Crossref] [PubMed]
- Xu Z, Yang Q, Chen X, et al. Clinical associations and prognostic value of site-specific metastases in non-small cell lung cancer: A population-based study. Oncol Lett 2019;17:5590-600. [Crossref] [PubMed]
- Li J, Zhu H, Sun L, et al. Prognostic value of site-specific metastases in lung cancer: A population based study. J Cancer 2019;10:3079-86. [Crossref] [PubMed]
- Ashour Badawy A, Khedr G, Omar A, et al. Site of Metastases as Prognostic Factors in Unselected Population of Stage IV Non-Small Cell Lung Cancer Asian Pac J Cancer Prev 2018;19:1907-10. [PubMed]
- Tamura T, Kurishima K, Nakazawa K, et al. Specific organ metastases and survival in metastatic non-small-cell lung cancer. Mol Clin Oncol 2015;3:217-21. [Crossref] [PubMed]
- Gibson AJW, Li H, D'Silva A, et al. Impact of number versus location of metastases on survival in stage IV M1b non-small cell lung cancer. Med Oncol 2018;35:117. [Crossref] [PubMed]
- Kris MG, Johnson BE, Berry LD, et al. Using multiplexed assays of oncogenic drivers in lung cancers to select targeted drugs. JAMA 2014;311:1998-2006. [Crossref] [PubMed]
- Choi BD, Shankar GM, Sivaganesan A, et al. Implication of Biomarker Mutations for Predicting Survival in Patients With Metastatic Lung Cancer to the Spine. Spine (Phila Pa 1976) 2018;43:E1274-80. [Crossref] [PubMed]
- Yoshida T, Yoh K, Goto K, et al. Impact of EGFR tyrosine kinase inhibitors on skeletal-related events in EGFR mutation-positive non-small cell lung cancer patients with bone metastases. J Clin Oncol 2012;30:abstr e18054.
- Hirsh V, Major PP, Lipton A, et al. Zoledronic acid and survival in patients with metastatic bone disease from lung cancer and elevated markers of osteoclast activity. J Thorac Oncol 2008;3:228-36. [Crossref] [PubMed]
- Yao NS, Wu YY, Janckila AJ, et al. Serum tartrate-resistant acid phosphatase 5b (TRACP5b) activity as a biomarker for bone metastasis in non-small cell lung cancer patients. Clin Chim Acta 2011;412:181-5. [Crossref] [PubMed]
- Brown JE, Cook RJ, Major P, et al. Bone turnover markers as predictors of skeletal complications in prostate cancer, lung cancer, and other solid tumors. J Natl Cancer Inst 2005;97:59-69. [Crossref] [PubMed]
- Lecouvet FE, Talbot JN, Messiou C, et al. Monitoring the response of bone metastases to treatment with Magnetic Resonance Imaging and nuclear medicine techniques: a review and position statement by the European Organisation for Research and Treatment of Cancer imaging group. Eur J Cancer 2014;50:2519-31. [Crossref] [PubMed]
- Therasse P, Arbuck SG, Eisenhauer EA, et al. New guidelines to evaluate the response to treatment in solid tumors. European Organization for Research and Treatment of Cancer, National Cancer Institute of the United States, National Cancer Institute of Canada. J Natl Cancer Inst 2000;92:205-16. [Crossref] [PubMed]
- Hamaoka T, Costelloe CM, Madewell JE, et al. Tumour response interpretation with new tumour response criteria vs the World Health Organisation criteria in patients with bone-only metastatic breast cancer. Br J Cancer 2010;102:651-7. [Crossref] [PubMed]
- Hamaoka T, Madewell JE, Podoloff DA, et al. Bone imaging in metastatic breast cancer. J Clin Oncol 2004;22:2942-53. [Crossref] [PubMed]
- Kouloulias EV, Kouvaris RJ, Antypas C, et al. An intra-patient dose-escalation study of disodium pamidronate plus radiotherapy versus radiotherapy alone for the treatment of osteolytic metastases. Monitoring of recalcification using image-processing techniques. Strahlenther Onkol 2003;179:471-9. [Crossref] [PubMed]
- Tokito T, Shukuya T, Akamatsu H, et al. Efficacy of bevacizumab-containing chemotherapy for non-squamous non-small cell lung cancer with bone metastases. Cancer Chemother Pharmacol 2013;71:1493-8. [Crossref] [PubMed]
- Rong D, Mao Y, Yang Q, et al. Early osteosclerotic changes predict chemotherapy response in non-small-cell lung cancer patients with bone metastases. Eur Radiol 2018;28:4362-9. [Crossref] [PubMed]
- Yamashita Y, Aoki T, Hanagiri T, et al. Osteosclerotic lesions in patients treated with gefitinib for lung adenocarcinomas: a sign of favorable therapeutic response. Skeletal Radiol 2012;41:409-14. [Crossref] [PubMed]
- Zhao E, Xu H, Wang L, et al. Bone marrow and the control of immunity. Cell Mol Immunol 2012;9:11-9. [Crossref] [PubMed]
- Hellmann MD, Paz-Ares L, Bernabe Caro R, et al. Nivolumab plus Ipilimumab in Advanced Non-Small-Cell Lung Cancer. N Engl J Med 2019;381:2020-31. [Crossref] [PubMed]
- Peters S, Cappuzzo F, Horn L, et al. OA03. 05 Analysis of early survival in patients with advanced non-squamous NSCLC treated with nivolumab vs docetaxel in CheckMate 057. J Thorac Oncol 2017;12:S253. [Crossref]
- Qin A, Zhao S, Miah A, et al. Bone Metastases, Skeletal-Related Events, and Survival in Patients With Metastatic Non-Small Cell Lung Cancer Treated With Immune Checkpoint Inhibitors. J Natl Compr Canc Netw 2021;19:915-21. [Crossref] [PubMed]
- Tamiya M, Tamiya A, Inoue T, et al. Metastatic site as a predictor of nivolumab efficacy in patients with advanced non-small cell lung cancer: A retrospective multicenter trial. PLoS One 2018;13:e0192227. [Crossref] [PubMed]
- Nakata E, Sugihara S, Sugawara Y, et al. Early response of bone metastases can predict tumor response in patients with non-small-cell lung cancer with bone metastases in the treatment with nivolumab. Oncol Lett 2020;20:2977-86. [Crossref] [PubMed]
- Cancer Genome Atlas Research Network. Comprehensive molecular profiling of lung adenocarcinoma. Nature 2014;511:543-50. [Crossref] [PubMed]
- Govindan R. Cancer. Attack of the clones. Science 2014;346:169-70. [Crossref] [PubMed]