Consistency analysis of high-sensitivity cardiac troponin I in peripheral blood and venous blood by quantum dot immunofluorescence assay and clinical application in acute myocardial infarction
Introduction
Cardiovascular disease (CVD) is the main cause of premature death and disability worldwide, with an increasing incidence (1,2). Acute myocardial infarction (AMI) is the most common clinical form of coronary syndrome, and non-diagnostic AMI may lead to increasing human mortality worldwide (3,4). Therefore, rapid monitoring and follow-up of AMI symptoms in patients is important for clinical diagnosis, which will greatly reduce the risk of further life-threatening problems (5). In general, the occurring AMI affects the increase in cardiac biomarker levels in the blood. Cardiac troponin is an outstanding biomarker for AMI, which is of great value for the monitoring and diagnosis of AMI (6). Therefore, the development of an easy-to-use quantitative troponin method is highly desirable for rapid diagnosis of AMI disease and follow-up of treatment process
Current methods of detecting cTnI include electrochemiluminescence and enzyme-linked immunofluorescence, which require to collect venous blood samples from the patient’s arm (7,8). The time it takes between a blood sample and the reporting of test findings is a significant impediment to making quick decisions. The time spent transferring blood to a central laboratory and then centrifuging it to create a plasma sample is a substantial component of the turnaround time in this procedure. Point-of-care cardiac troponin tests that use peripheral blood and have quick turnaround times may help to speed up decision making.
In recent years, peripheral blood testing has attracted more and more attention. For instance, Tomimuro et al. developed a µTADs device combined with Bret’s sensor protein to rapidly detect antibodies in human peripheral blood samples (9). Sarangadharan et al. have developed a hand-held biosensor system to rapidly screen for brain natriuretic peptide (BNP) from a single drop of whole blood (10). There are few reports on the detection of peripheral blood troponin. This study used quantum dot immunofluorescence technology combined with bedside POCT instrument to detect troponin in peripheral blood.
Quantum dots are characterized by high fluorescence efficiency, good stability, high sensitivity and rapid quantification (11,12). Quantum dots is applied in many biomedical filed for its unique optoelectronic properties as a novel option (13,14). Quantum dots are used as superior luminescence tags for their broad excitation spectrum, narrow emission spectrum and large Stokes shift (>100 nm). Such optoelectronic properties are important in multiplexed applications as unique light source, since it could excite multiple quantum dots with different sizes at the same time (15). Additionally, quantum dots demonstrate higher molar adsorption coefficients and quantum yield compared with organic fluorophore. Therefore, fluorescent NPs are almost 20-fold brighter and thousand-fold more enduring compared with traditional organic dyes (16). Such exceptional optical properties illustrate that quantum dots are one of the important photoluminescent probes which could be applied in many analytical experiments.
In this research, we evaluated a novel technique for the rapid testing of high-sensitivity cTnI (hs-cTnI) in peripheral blood by quantum dot fluorescence immunoassay and explored the clinical application of peripheral blood hs-cTnI. This technology has important value in pre-hospital emergency care, early AMI screening and the construction of national chest pain centers. We present the following article in accordance with the STARD reporting checklist (available at https://jtd.amegroups.com/article/view/10.21037/jtd-22-436/rc).
Methods
Patients
A total of 90 patients with chest pain admitted to Wuxi Second People’s Hospital of Nanjing Medical University between June 2019 and January 2021 were enrolled. Of them, 52 were diagnosed with AMI based on the 2017 ESC STEMI Guidelines (17). Laboratory evidence of AMI was defined as cTn value >99th percentile of the upper reference limit (URL) at least once. The clinical manifestations were consistent with those of myocardial ischemia. Patients with moderate to severe liver and kidney insufficiency, tumor or other chronic diseases were not included. The study was conducted in accordance with the Declaration of Helsinki (as revised in 2013). The study was approved by the Ethics Committee of Wuxi Second People’s Hospital of Nanjing Medical University (No. 2020-Y-19) and informed consent was taken from all the patients.
Materials and Instruments
The instrument is Nanjing Vazyme dry fluorescence immunoassay analyzer AFS-1000. The reagent is the matching troponin test card and the diluent used to detect peripheral blood. The venous blood is lithium heparin anticoagulated whole blood, and the peripheral blood is the patient’s fingertip blood.
Study methods
At present, venous blood troponin detection is routinely used in clinical practice. We compared the peripheral blood troponin results with the venous blood troponin results. A 30 µL peripheral blood sample was collected from each patient’s ring finger and added to 90 µL of diluent. After the sample was mixed, take 80 µL from the dilution to detect hs-cTnI using Vazyme dry fluorescence immunoassay analyzer AFS-1000. A 3–5 mL venous blood sample was collected from the patient’s vein and was placed into a lithium heparin anticoagulant tube. Taken 80 µL from lithium heparin anticoagulation tube to detect hs-cTnI using Vazyme dry fluorescence immunoassay analyzer AFS-1000. The peripheral and venous blood samples were collected at the same time and comprised the two study groups. The diagnostic criteria for AMI include a history of ischemic chest pain, myocardial ischemic necrosis ECG dynamic changes, and serum myocardial dynamic changes biochemical markers (mainly hs-cTn). The results of hs-cTn are based on venous blood quantum dot immunofluorescence detection.
Statistical analysis
GraphPad Prism8 and SPSS24 were used for statistical analysis. Measurement data were expressed as , t-test was used to compare the differences between the two groups, and linear correlation was used for correlation analysis. A quantum dot fluorescence immunoassay was used to establish the standard curves for clinical detection of hs-cTnI in peripheral and to verify the test’s precision and linear range.
We constructed a receiver operating characteristic (ROC) curve to analyze the diagnostic efficacy of peripheral blood quantum dot immunofluorescence hs-cTnI in AMI, and then compared the area under the ROC curve (AUC) with the corresponding value of venous blood hs-cTnI Compare. Calculate the sensitivity, specificity, correct rate, positive predictive value and negative predictive value of peripheral blood hs-cTn for diagnosing AMI. The value of the area under the ROC curve is between 0.5 and 1.0. When AUC is greater than 0.5, the closer the AUC is to 1.0, the better the diagnostic effect. Bland-Altman analysis use SPSS24 to assess the agreement of the two methods. All hypothesis tests were two-tailed. P<0.05 was considered statistically significant
Results
Establishment of rapid detection of hs-cTnI in peripheral blood by quantum dot fluorescence immunoassay
Establishment of test standard curve
Using 9 concentration gradients of hs-cTnI as the X-axis and the signal ratio T/C of the dry fluorescence immunoassay analyzer as the Y-axis (n=3), the standard curves of hs-cTnI detection in venous blood and peripheral blood by quantum dot fluorescence immunoassay were established (Figure 1).
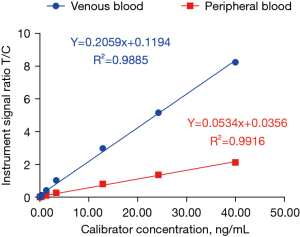
Precision verification
The levels of hs-cTnI (n=3) of fixed batches at low (0.03 ng/mL), medium (1.33 ng/mL), and high (24.26 ng/mL) concentration gradients were measured using the above established standard curves of venous blood and peripheral blood (Figure 2A-2C). The intra-batch precision of venous blood and peripheral blood was calculated (Table 1), and the coefficient of variation (CV) of peripheral blood method was <15% (Table 1).
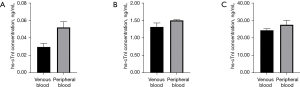
Table 1
Analytical performance | Venous blood | Peripheral blood |
---|---|---|
Linear range | 0.02–40 ng/mL | 0.05–40 ng/mL |
Limit of detection | ≈0.02 ng/mL | ≈0.05 ng/mL |
In-batch precision (low) | 13.3% | 12.7% |
In-batch precision (medium) | 8.6% | 1.3% |
In-batch precision (high) | 3.6% | 9.2% |
hs-cTnI, high-sensitivity troponin I.
Linear range verification
Nine concentration gradients of hs-cTnI were detected. Linear regression was performed using the X-axis as the standard concentration and the Y-axis as the measured concentration (n=3). The venous blood and peripheral blood methods were linear in 0.02–40 ng/mL (Figure 3, Table 1).
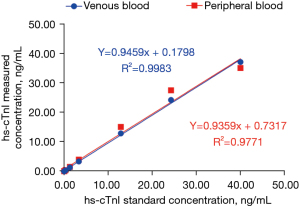
Minimum detection limit verification
hs-cTnI concentrations of 0.02, 0.03, 0.04, 0.05 and 0.06 ng/mL were detected by quantum dot fluorescence immunoassay in peripheral blood, and the lowest detection limit was about 0.05 ng/mL (Table 1).
Correlation analysis of hs-cTnI detection results by two methods
The detection result of hs-cTnI in peripheral blood was the X-axis, and the detection result of hs-cTnI in whole venous blood was the Y-axis. The regression equation between them was Y=1.026x+0.521, and the correlation coefficient was R2=0.9337 (Figure 4A). There were 39 cases with hs-cTnI <0.5 ng/mL. The regression equation was Y=0.655x+0.015, and the correlation coefficient R2=0.8011. All were linearly correlated (P<0.05) (Figure 4B). With hs-cTnI >0.5 ng/mL as the positive limit, there were 51 cases in total. The regression equation was Y=1.005x+1.249, and the correlation coefficient R2=0.9099 (Figure 4C).
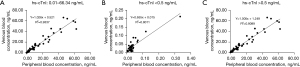
Distribution of hs-cTnI in peripheral blood and venous blood and Bland-Altman analysis
The hs-cTnI test results of the two methods ranged from 0.01 to 66.34 ng/mL, and t-test revealed P=0.7462, showing no statistically significant difference (Figure 5A). The mean value of the hs-cTnI test results of the two methods was the X-axis, and the difference was the Y-axis: 94.4% (85/90) of the data were within the consistency limit (Figure 5B).
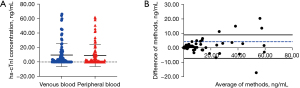
Evaluation of clinical diagnostic efficacy
Using hs-cTnI 0.5 ng/mL as the positive threshold, we predicted the diagnosis of AMI and compared the predicted results with the final clinical diagnosis. Clinical sensitivity (94.5%:87.3%), specificity (89.5%:89.5%), accuracy (90%:87.8%), positive predictive value (90.4%:91.8%), negative predictive value (87.2%:82.3%) of hs-cTnI detected in venous blood and peripheral blood are shown in Figure 6.
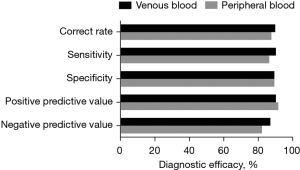
Diagnostic value in patients with AMI
The receiver operating characteristic curves of AMI diagnosis and prediction were drawn. The area under the curve for hs-cTnI was 0.9431 [95% confidence interval (CI), 0.8918 to 0.9944] for venous blood and 0.9352 (95% CI, 0.8829 to 0.9876) for peripheral blood. The cut-off value of hs-cTnI in venous blood for the diagnosis of AMI was 1.598, and the sensitivity was 82.69%. The diagnostic accuracy was high, and the difference was not statistically significant (P>0.05) (Figure 7).
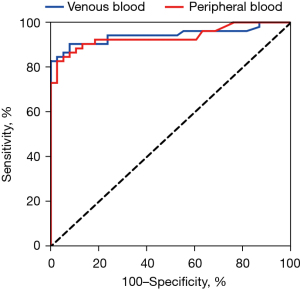
Discussion
The level of troponin in the blood has important value in predicting infarct size, evaluating the thrombolytic effect and identifying unstable angina pectoris (18-20). When cardiomyocytes undergo necrosis due to ischemia and hypoxia, as in AMI, cTnI is initially released in a free state, but with progression of the injury, cTnI in the bound state is continuously released as a result of the cellular destruction. Therefore, the blood cTnI concentration shows a bimodal change, and the diagnostic time window can be as long as several weeks, which has important clinical significance for the rapid diagnosis of AMI.
We attempted to establish a new method for the rapid detection of hs-cTnI using a quantum dot fluorescence immunoassay in peripheral blood and evaluated it as a good substitute for the detection of hs-cTnI in whole venous blood.
Quantum dots are semiconductor nanoparticles with a radius that is smaller than or close to the radius of the Bohr exciton, but generally, they are 1–10 nm. Quantum dots are used as fluorescent probes, generating fluorescence signals through excitation of the dots, which can be measured by a device as quantitative data. Their advantages include wide excitation wavelength range, narrow emission wavelength, adjustable fluorescence size, high sensitivity, good optical stability, long fluorescence life, large Stokes displacement, and high quantum fluorescence efficiency (21,22). Quantum dots overcome the disadvantages of other markers, such as short chemiluminescence, precise environmental requirements, poor reproducibility, poor stability (e.g., fluorescent dyes), inactivation of enzymes and low sensitivity (23,24). Quantum dots fluorescence immunoassay combines the advantages of immunoassay technology and chromatography, making it simple, rapid and highly specific for rapid diagnosis of AMI.
The results showed that detection of hs-cTnI in peripheral blood by the quantum dot fluorescence immunoassay met the basic requirements of clinical detection with good analytical performance. It detected hs-cTnI in peripheral blood in a certain linear concentration range, and at a low concentration. Secondly, there was a significant correlation between the hs-cTnI detection results for peripheral blood and those for venous blood, with good comparability and consistency. For the clinical diagnosis of AMI, the specificity was the same and the diagnostic accuracy was high. Therefore, quantum dot fluorescence immunoassay of peripheral blood can be used for rapid detection of hs-cTnI and thus the clinical diagnosis and treatment of AMI patients. Our study reveals the potential of a simple and miniaturized peripheral blood device for bedside analysis. The key point of this technology is the sensitivity and accuracy of peripheral blood detection. In terms of sensitivity, it can be further optimized by developing highly specific antibody raw materials and more suitable process formulations; the values of other manufacturers are compared and verified with the clinical symptoms of patients.
Further optimization of the detection of hs-cTnI by quantum dot fluorescence immunoassay in peripheral blood for clinical application is a new direction that can be realized in the future. With continuous research and development of the peripheral blood hs-cTnI detection kit, it will certainly assist in the rapid diagnosis and differential diagnosis of AMI in clinical departments and primary hospitals and meet the requirement of convenience.
Conclusions
Rapid detection of hs-cTnI in peripheral blood by quantum dot fluorescence immunoassay was successfully established as feasible. The quality indexes met the requirements of clinical detection, and the results were highly correlated and consistent with the results for venous blood, providing a good prospect for application in clinical settings.
Acknowledgments
Funding: This work was funded by the Clinical Technology Foundation of Wuxi (No. Z201804).
Footnote
Reporting Checklist: The authors have completed the STARD reporting checklist. Available at https://jtd.amegroups.com/article/view/10.21037/jtd-22-436/rc
Data Sharing Statement: Available at https://jtd.amegroups.com/article/view/10.21037/jtd-22-436/dss
Conflicts of Interest: All authors have completed the ICMJE uniform disclosure form (available at https://jtd.amegroups.com/article/view/10.21037/jtd-22-436/coif). The authors have no conflicts of interest to declare.
Ethical Statement: The authors are accountable for all aspects of the work in ensuring that questions related to the accuracy or integrity of any part of the work are appropriately investigated and resolved. The study was conducted in accordance with the Declaration of Helsinki (as revised in 2013), and was approved by the Ethics Committee of Wuxi Second People’s Hospital of Nanjing Medical University (No. 2020-Y-19) and informed consent was taken from all the patients.
Open Access Statement: This is an Open Access article distributed in accordance with the Creative Commons Attribution-NonCommercial-NoDerivs 4.0 International License (CC BY-NC-ND 4.0), which permits the non-commercial replication and distribution of the article with the strict proviso that no changes or edits are made and the original work is properly cited (including links to both the formal publication through the relevant DOI and the license). See: https://creativecommons.org/licenses/by-nc-nd/4.0/.
References
- Zhao D, Liu J, Wang M, et al. Epidemiology of cardiovascular disease in China: current features and implications. Nat Rev Cardiol 2019;16:203-12. [Crossref] [PubMed]
- Mensah GA, Roth GA, Fuster V. The Global Burden of Cardiovascular Diseases and Risk Factors: 2020 and Beyond. J Am Coll Cardiol 2019;74:2529-32. [Crossref] [PubMed]
- Yang Z, Min Zhou D. Cardiac markers and their point-of-care testing for diagnosis of acute myocardial infarction. Clin Biochem 2006;39:771-80. [Crossref] [PubMed]
- Ju H, Lai G, Yan F. Immunosensing for Detection of Protein Biomarkers. Elsevier 2017:207-37.
- Han X, Li S, Peng Z, et al. Recent Development of Cardiac Troponin I Detection. ACS Sensors 2016;1:106-14. [Crossref]
- Çimen D, Bereli N, Günaydın S, et al. Detection of cardiac troponin-I by optic biosensors with immobilized anti-cardiac troponin-I monoclonal antibody. Talanta 2020;219:121259. [Crossref] [PubMed]
- Chow SL, Maisel AS, Anand I, et al. Role of Biomarkers for the Prevention, Assessment, and Management of Heart Failure: A Scientific Statement From the American Heart Association. Circulation 2017;135:e1054-91. [Crossref] [PubMed]
- Song J, Murugiah K, Hu S, et al. Incidence, predictors, and prognostic impact of recurrent acute myocardial infarction in China. Heart 2020;107:313-8. [Crossref] [PubMed]
- Tomimuro K, Tenda K, Ni Y, et al. Thread-Based Bioluminescent Sensor for Detecting Multiple Antibodies in a Single Drop of Whole Blood. ACS Sens 2020;5:1786-94. [Crossref] [PubMed]
- Sarangadharan I, Wang SL, Tai TY, et al. Risk stratification of heart failure from one drop of blood using hand-held biosensor for BNP detection. Biosens Bioelectron 2018;107:259-65. [Crossref] [PubMed]
- Bian F, Sun L, Cai L, et al. Quantum dots from microfluidics for nanomedical application. Wiley Interdiscip Rev Nanomed Nanobiotechnol 2019;11:e1567. [Crossref] [PubMed]
- Guo Y, Li J. MoS2 quantum dots: synthesis, properties and biological applications. Mater Sci Eng C Mater Biol Appl 2020;109:110511. [Crossref] [PubMed]
- Coto-García AM, Sotelo-González E, Fernández-Argüelles MT, et al. Nanoparticles as fluorescent labels for optical imaging and sensing in genomics and proteomics. Anal Bioanal Chem 2011;399:29-42. [Crossref] [PubMed]
- Suárez PL, García-Cortés M, Fernández-Argüelles MT, et al. Functionalized phosphorescent nanoparticles in (bio)chemical sensing and imaging - A review. Anal Chim Acta 2019;1046:16-31. [Crossref] [PubMed]
- Geissler D, Charbonnière LJ, Ziessel RF, et al. Quantum dot biosensors for ultrasensitive multiplexed diagnostics. Angew Chem Int Ed Engl 2010;49:1396-401. [Crossref] [PubMed]
- Sukhanova A, Devy J, Venteo L, et al. Biocompatible fluorescent nanocrystals for immunolabeling of membrane proteins and cells. Anal Biochem 2004;324:60-7. [Crossref] [PubMed]
- Ibanez B, James S, Agewall S, et al. 2017 ESC Guidelines for the management of acute myocardial infarction in patients presenting with ST-segment elevation: The Task Force for the management of acute myocardial infarction in patients presenting with ST-segment elevation of the European Society of Cardiology (ESC). Eur Heart J 2018;39:119-77. [Crossref] [PubMed]
- Tang O, Matsushita K, Coresh J, et al. High-Sensitivity Cardiac Troponin I and T for Cardiovascular Risk Stratification in Adults With Diabetes. Diabetes Care 2020;43:e144-6. [Crossref] [PubMed]
- Corrigendum to. A Possible Mechanism behind Faster Clearance and Higher Peak Concentrations of Cardiac Troponin I Compared with Troponin T in Acute Myocardial Infarction. Clin Chem 2020;66:1573. [Crossref] [PubMed]
- Apple FS, Fantz CR, Collinson PO, et al. Implementation of High-Sensitivity and Point-of-Care Cardiac Troponin Assays into Practice: Some Different Thoughts. Clin Chem 2021;67:70-8. [Crossref] [PubMed]
- Kargozar S, Hoseini SJ, Milan PB, et al. Quantum Dots: A Review from Concept to Clinic. Biotechnol J 2020;15:e2000117. [Crossref] [PubMed]
- Wu M, Chen Z, Xie Q, et al. One-step quantification of salivary exosomes based on combined aptamer recognition and quantum dot signal amplification. Biosens Bioelectron 2021;171:112733. [Crossref] [PubMed]
- Pu L. Enantioselective Fluorescent Recognition of Free Amino Acids: Challenges and Opportunities. Angew Chem Int Ed Engl 2020;59:21814-28. [Crossref] [PubMed]
- Pohanka M. Current Biomedical and Diagnostic Applications of Gold Micro and Nanoparticles. Mini Rev Med Chem 2021;21:1085-95. [Crossref] [PubMed]
(English Language Editor: K. Brown)