Electromagnetic navigation transthoracic needle aspiration for the diagnosis of pulmonary nodules: a safety and feasibility pilot study
Introduction
Results of the National Lung Screening Trial (NLST) found a 20% risk in lung cancer related deaths in patients screened annually for 3 years with low dose CT. Currently, over 8 million Americans meet criteria for lung cancer screening (1) with more than 10 million chest CT scans performed annually in the United States (2,3). This number is likely to escalate in part due to the results of the NLST and subsequent Medicare coverage of lung cancer screening (4,5). However, the management of solitary pulmonary nodules (SPN) remains a diagnostic challenge. The majority of nodules will be benign, but the opportunity to diagnose an early and potentially curable lung cancer is critical (6). The benefit of an early diagnosis also needs to be weighed against the risk of procedural complications.
The three most common methods to diagnose a SPN are bronchoscopy, transthoracic needle aspiration (TTNA), and surgical biopsy (7). Despite new technologies, the diagnostic yield for bronchoscopy remains as low as 33% (8). Developing a systematic approach that combines staging of disease in combination with a high diagnostic yield in the periphery, without increased complications are needed. Recently, a novel diagnostic tool has been developed allowing for physicians to perform electromagnetic guidance transthoracic needle aspiration (ETTNA). The technology incorporates a unique electromagnetic guidance system allowing clinicians to track SPN and target them for ETTNA without utilizing real time CT in the operating room or bronchoscopy suite. Providing this capability allows the pulmonologist to perform initial lymph node staging with endobronchial ultrasound (EBUS) in the same procedural setting. This approach may provide a much needed intervention allowing physicians to utilize a multimodality approach in a single procedural setting to optimize diagnostic yield and limit complications.
We report the safety, feasibility and diagnostic yield performing a complete staging and diagnostic evaluation in patient with a SPN utilizing a novel thoracic navigation system which allows a combination of minimally invasive biopsies [EBUS, navigational bronchoscopy (NB), ETTNA] in a single procedural session.
Methods
This was a prospective single arm pilot study examining the safety, feasibility and diagnostic yield of ETTNA, NB and EBUS in a single procedural setting. Institutional Review Board approval was obtained at the Johns Hopkins Hospital (NA00086035) and the trial was registered on clinicaltrials.gov (NCT02109458). Primary outcomes were feasibility of procedural performance and adverse events from ETTNA including pneumothorax, chest tube requirement, respiratory distress, and bleeding. The secondary outcome was diagnostic yield.
Patients were enrolled after informed consent from November 2013 until August 2014. Inclusion criteria included; age >18 years, undiagnosed SPN (≥10 mm and ≤30 mm surrounded by lung parenchyma) that were accessible by an anterior or lateral chest percutaneous approach with a clinical indication to undergo bronchoscopy for diagnosis of a SPN. Subjects were excluded if they had positron emission tomography (PET) positive mediastinal lymphadenopathy and/or mediastinal lymphadenopathy ≥10 mm on CT imaging, clinical contradictions to undergo standard flexible bronchoscopy, inability to provide informed consent or age ≤18 years of age.
On the day of the procedure, all patients underwent a chest CT scan to create a virtual airway map (Veran Medical, St Louis, MO, USA) with 0.5 mm intervals and 0.67–0.75 mm thickness after placement of a navigational tracking pad (vPAD2, Veran Medical) on the patients anterior chest (Figure 1). The target nodule was identified and a virtual bronchoscopic planning route to the SPN was created (Figure 2). The chest wall entry site for ETTNA was selected by the performing bronchoscopist to avoid organ or vascular injury and a confirmed navigational pathway to the SPN (target crosshairs within the target lesion) was identified (Figure 3).
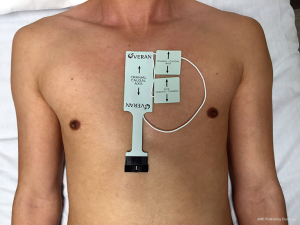
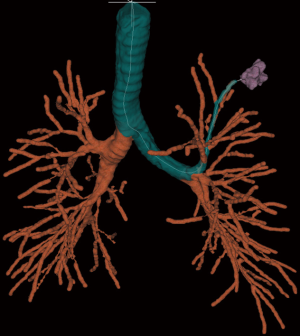
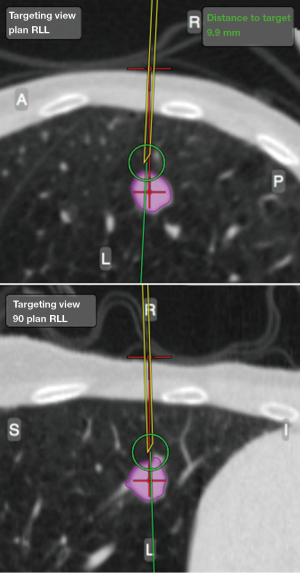
All patients were placed under deep sedation by a board certified anesthesiologist with a laryngeal mask airway in place. Patients were observed under standard bronchoscopy monitoring protocols. Procedures were performed by an Interventional Pulmonologist at the Johns Hopkins Hospital (LY, DFK, and HL). A convex EBUS (Olympus BF-UC180F) was performed for full lymph node staging. This was followed by NB (SPiNView® Thoracic Navigation System, Veran Medical) using an electromagnetic tip tracked biopsy instrument (1.8 mm OD Serrated Cup Always-On Tip Tracked Forceps, Veran Medical) via a thin bronchoscope with a 4-mm outer diameter (Olympus BF-MP160F). The biopsy instrument was navigated to the SPN if the SPN was localized by NB [target crosshairs within the target lesion with the tip tracked instrument, a transbronchial forceps (TBBX) and/or TBNA with brushings and bronchoalveolar lavage (BAL)] were performed (Figure 4). If onsite cytology evaluation of the peripheral sample yielded a positive diagnosis, additional samples were collected for mutational analysis. If NB did not yield a confirmatory onsite diagnosis, the bronchoscope was removed and ETTNA (Veran Medical, SpinPerc) was attempted under continued deep sedation. Under sterile conditions, the biopsy area was prepped and draped. One percent lidocaine was injected subcutaneously followed by percutaneous placement of an electromagnetic tip tracked biopsy needle introducer (19 gauges × 105 mm) under navigational guidance (Figure 5). The SPN was localized and a 20-gauge coaxial core biopsy device (SuperCore Argon Medical) device was used. The biopsy device was rotated 90 degrees after each biopsy and a minimum of four samples were obtained. After the ETTNA samples were obtained, the needle introducer was removed and a simple bandage was placed. Sedation was then weaned and the patient was transported to the postoperative care unit for standard monitoring.
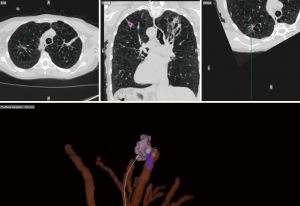
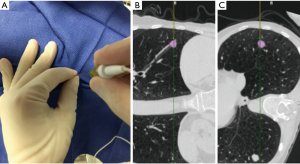
All subjects had a post procedural chest radiograph within two hours of the procedure. If a pneumothorax was present, chest tube insertion criteria was based on the British Thoracic Society guidelines and the patient was admitted for chest tube management (9). If chest tube placement was not needed, a four hour follow up chest radiograph was performed and if the pneumothorax was stable or decreasing in size the patient was discharged to home with short term interval follow up to confirm complete resolution.
Additional data collected included patient demographics, nodule size (longest axis), nodule location, presence of air bronchus sign, distance from the pleural surface to the edge of the nodule in its shortest path, complications (pneumothorax, respiratory distress, hemoptysis, bleeding graded), diagnostic results, and time for each component of the procedure (EBUS, NB, ETTNA). Bleeding complications during the procedure were recorded using a modified bleeding scale (Grade 0= none, 1= suction, 2= iced saline, 3= bronchial blocker, 4= surgical intervention).
All non-diagnostic biopsies underwent short interval follow up CT imaging which unless the CT revealed a decrease in size or complete resolution of the nodule within six months; all patients underwent a video assisted wedge resection for a definitive diagnosis by a board certified thoracic surgeon. Nodules with a non-malignant but non disease specific definitive pathologic diagnosis (i.e., fibrosis) after the procedure were followed for at least twelve months with surveillance CT to ensure stability with plans for a video assisted wedge resection if there was any observed growth.
Statistical comparisons were made using chi-square analysis where applicable. Continuous variables are described using means with standard deviations (SD) along with range and/or 95% confidence intervals based on normal theory. Dichotomous variables are summarized in simple proportions along with exact binomial 95% confidence intervals. Statistical comparisons of continuous variables were done using ANOVA, while those comparing proportions were done using the Fisher’s exact test. A P value of less than 0.05 indicated statistical significance. The statistical power analysis (80% power and a one-sided alpha of 0.05) was based on the a priori hypothesis that the combined diagnostic yield of ETTNA and NB would result in a diagnostic yield of 85% when compared to historical controls of NB alone. For the comparative cohort we assumed a bronchoscopic diagnostic yield of 61% utilizing historical control data for SPN of this size (10). The sample size for these parameters was 24 patients. All statistical analysis was performed using STATA software (version 13.1).
Results
A total of 594 patients (all patients undergoing any bronchoscopy during the enrollment period) were assessed for eligibility of which 35 met inclusion criteria during the study period. Eleven patients declined consent (Figure 6). Twenty-four subjects were recruited into the study (Table 1). Twenty-four EBUS and NB and twenty-three ETTNA cases were performed. In one subject an ETTNA was not performed due to an anatomic limitation of an intercostal rib interfering with the needle entry angle for ETTNA. The mean SPN size was 20.3 mm (range, 12–29 mm) and mean distance from the pleural surface to the edge of the nodule in its shortest path distance was 12.6 mm (range, 1.6–29.5 mm) (Figure 7). Anatomic lobar location of the nodule, measurements and radiographic specifics are presented in Table 2.
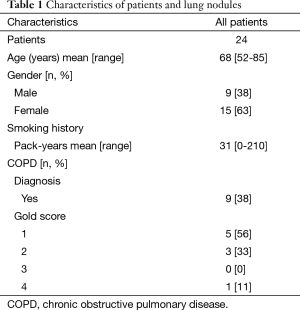
Full table
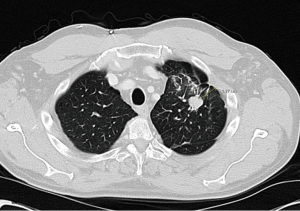
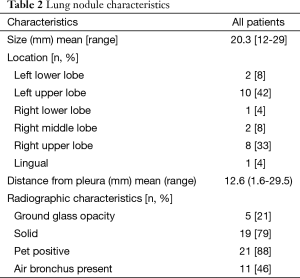
Full table
No bleeding, hemoptysis, or respiratory events were encountered. There were five (5/24, 21%) pneumothoraces of which only two (2/24, 8%) subjects required chest tube placement. None of the chest tube placements were emergent. In the five cases with a pneumothorax, 4/5 (80%) had a diagnosis from the procedure. All four of these cases were diagnostic from the ETTNA sample alone. All five samples from patients who experienced a pneumothorax yielded lung tissue on pathologic analysis.
Total procedural time as defined by the time the EBUS bronchoscope was inserted into the oropharynx until the time the ETTNA needle was removed was 72.5 minutes. Total EBUS procedural time from insertion of the EBUS scope to removal of the EBUS scope was 20.5 minutes. Total NB time as defined by the time from initial insertion of the flexible bronchoscope into the oropharynx until removal of the scope at the conclusion of the NB phase of the procedure was 22.9 minutes. Total ETTNA time as defined by the start of sterile field preparation prior to initial needle placement until needle removal was 18.3 minutes.
The diagnostic yield for ETTNA alone was 83% alone. The combined diagnostic yield of ETTNA and NB was 87% (P=0.0016; exact 95% CI: 65%, 97%). The diagnostic yield increased further when combining EBUS, NB and ETTNA in a single procedure to 92% (P=0.0001). NB was diagnostic in only 33% of all cases, but had a diagnostic yield of 73% when an air bronchus sign was present on CT scan consistent with prior navigational data for SPN bronchoscopic targets within the inclusion criteria (11).
ETTNA was the only biopsy method that was diagnostic in nine subjects, and NB was the only diagnostic biopsy method in one subject. EBUS was diagnostic in five cases with four EBUS cases revealing adenocarcinoma (N3=1, N1=3) and one case revealing granuloma. Of the four EBUS malignant cases, three were also diagnostic by NB and/or ETTNA. 13/24 (54%) of the patients enrolled had a final malignant diagnosis. One hundred percent of the patients had a definitive final diagnosis at the conclusion of this study (Table 3).
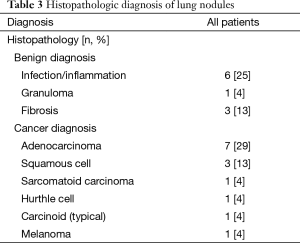
Full table
Three cases of inflammatory changes seen on biopsy were followed with short term CT surveillance which all revealed a decrease in size or resolution at which point the result was labeled as a true positive finding. Four subjects with a non-malignant diagnosis including non-necrotizing granulomas (sarcoidosis) and fibrosis were followed radiographically for 12 months with nodule stability in all cases.
Discussion
CT guided TTNA is the proven minimally invasive standard for SPN diagnostics and has a higher diagnostic yield than bronchoscopy (8,12-14). However, the rate of TTNA complications is higher with a pneumothorax rate as high as 43% vs. ≤4% with bronchoscopy (15). Although TTNA may provide access to the primary lesion, it lacks the ability to provide staging information requiring some patients to undergo an additional separate procedure prior to treatment (i.e., EBUS or mediastinoscopy). A minimally invasive alternative to TTNA is desired for diagnosing SPN, but the yields of these technologies were historically lacking.
The results of our pilot study using a novel electromagnetic guidance system to perform ETTNA resulted in a 96% feasibility rate of bronchoscopists performing ETTNA with an acceptable safety profile. Although TTNA may be a relatively underutilized procedure for pulmonologists, our pneumothorax rates were comparable to the NLST pneumothorax data in procedures performed by interventional radiologists with comparable chest tube placement rates. The additional time to perform the ETTNA was shorter than either EBUS or NB and more importantly, the additional procedure time was not associated with any anesthesia related complications. Given that ETTNA sampling is not based on real time image guidance but on a computer generated model, there was potential concern that a pneumothorax would affect overall diagnostic yield. However, despite the presence of a pneumothorax, a definitive diagnosis was still obtained in 4/5 (80%) of subjects with a pneumothorax.
The combination of performing ETTNA, NB and EBUS in a single procedural setting resulted in a 92% overall diagnostic yield. Given the pilot design of this study, the overall diagnostic yield data needs larger clinical trials to confirm, however the pilot data does support the safety and feasibility of pulmonologists to perform this procedure in a single setting. There may in fact be additional safety benefits of this combined modality by limiting separate procedures (bronchoscopy and CT guided TTNA) and the independent variable anesthesia risks associated with each procedural encounter. The potential advantage of further increases in yield and decreases in pneumothorax rates with the use of rapid on-site cytology (ROSE) in combination with EBUS + NB + ETTNA is promising. Performing procedures in a stepwise fashion with ROSE and EBUS-TBNA first followed by NB followed by ETTNA if needed may further reduce complications. A stepwise algorithm with ROSE may allow for a diagnostic procedure with an even lower pneumothorax risk by performing transbronchial biopsy or TBNA and/or TTNA in only those cases that are needed. In this new era of health care reform and patient centered safety metrics, another clear advantage may be a single health care encounter which may allow for a patient to obtain all minimally invasive biopsies and diagnosis in one single setting. This approach could also help limit the need for invasive surgical biopsies until all other minimally invasive technics have not confirmed a diagnosis.
The diagnostic yield of ETTNA and NB was 87% (P=0.0016) and results in a potential clinically significant and meaningful improved outcome over prior bronchoscopic yield. The diagnostic yield for ETTNA alone was 82%, which was significantly better than NB but lower than some prior reports of TTNA by radiologists. A potential explanation may be that our nodule characteristics were heterogeneous with a mix of solid/semi-solid nodules (20.8% semi-solid). Reports of TTNA biopsy for semisolid nodules have a markedly lower sensitivity than solid nodules (51–84%) (16-18). Evidence that may also suggest procedural differences between subspecialists do not account for differences in yield or complications as shown by the fact that there were no run in procedures performed prior to study recruitment and no difference in complication rates between providers or sequential procedures.
There are several limitations to this study. Although powered for diagnostic yield, this pilot study was performed at a single high volume center with experienced bronchoscopists with significant NB experience and thus the generalizability of the yield results of this study warrant further investigation with larger clinical trials. However, the NB yield overall was comparable to previously published data suggesting the bronchoscopic approach is generalizable to a wide array of pulmonary practices (19). In addition, none of the investigators had any significant prior experience with this NB system or any prior experience with ETTNA. In this study the overall NB was only 33%. Although this is within the reported yield based on historical data, the fact that only SPN with a mean size of 20.3 mm were included reflect the true diagnostic challenges that pulmonologists are faced with in the setting of SPN (20). It is also established that the diagnostic yield markedly decreases without the air bronchus sign as again reflected in our study and favors using NB when an air bronchus sign is present (21). This highlights the limitations of bronchoscopic biopsy methods due to airway confinement and stresses the importance of novel combined minimally invasive methods including ETTNA. To further validate the yield results and provide an algorithmic approach to EBUS, NB and ETTNA, prospective multicentered studies are needed.
Conclusions
This is the first human pilot study demonstrating an acceptable safety and feasibility profile with a novel thoracic navigation system. Multicentered prospective trials to utilize a step-wise algorithmic approach to nodule sampling are needed to confirm these findings.
Acknowledgements
Veran Medical was the sponsor and funding source and provided the SPiN® Thoracic Navigation System and SpinPerc platforms used during the trial. The company had no participation in patient selection, procedural planning, patient results, data review, analysis or writing and/or review of the manuscript.
Footnote
Conflicts of Interest: Dr. Arias, Dr. Semaan, Dr. Wang, Ms. Frimpong, Ms. Oak-Jones, Dr. Thompson, and Mr. Ortiz have no conflicts to report. Drs. Yarmus, Feller-Kopman, Chen and Lee have all received institutional educational grants for CME activities, research support and consulting fees from Veran. The tested technology was borrowed for this study by the sponsor. All authors had full control of the design of the study, methods used, outcome parameters and results, analysis of data and production of the written report. Dr. Yarmus and Dr. Lee are the guarantors of the paper, taking responsibility for the integrity of the work as a whole, from inception to published article and attest that no undisclosed authors contributed to the manuscript.
References
- Ma J, Ward EM, Smith R, et al. Annual number of lung cancer deaths potentially avertable by screening in the United States. Cancer 2013;119:1381-5. [PubMed]
- Mettler FA Jr, Thomadsen BR, Bhargavan M, et al. Medical radiation exposure in the U.S. in 2006: preliminary results. Health Phys 2008;95:502-7. [PubMed]
- Berrington de González A, Mahesh M, Kim KP, et al. Projected cancer risks from computed tomographic scans performed in the United States in 2007. Arch Intern Med 2009;169:2071-7. [PubMed]
- National Lung Screening Trial Research Team, Aberle DR, Adams AM, et al. Reduced lung-cancer mortality with low-dose computed tomographic screening. N Engl J Med 2011;365:395-409. [PubMed]
- Moyer VA; U.S. Preventive Services Task Force. Screening for lung cancer: U.S. Preventive Services Task Force recommendation statement. Ann Intern Med 2014;160:330-8. [PubMed]
- Naidich DP, Bankier AA, MacMahon H, et al. Recommendations for the management of subsolid pulmonary nodules detected at CT: a statement from the Fleischner Society. Radiology 2013;266:304-17. [PubMed]
- Krochmal R, Arias S, Yarmus L, et al. Diagnosis and management of pulmonary nodules. Expert Rev Respir Med 2014;8:677-91. [PubMed]
- Schreiber G, McCrory DC. Performance characteristics of different modalities for diagnosis of suspected lung cancer: summary of published evidence. Chest 2003;123:115S-128S. [PubMed]
- MacDuff A, Arnold A, Harvey J, et al. Management of spontaneous pneumothorax: British Thoracic Society Pleural Disease Guideline 2010. Thorax 2010;65 Suppl 2:ii18-31. [PubMed]
- Wang Memoli JS, Nietert PJ, Silvestri GA. Meta-analysis of guided bronchoscopy for the evaluation of the pulmonary nodule. Chest 2012;142:385-93. [PubMed]
- Seijo LM, de Torres JP, Lozano MD, et al. Diagnostic yield of electromagnetic navigation bronchoscopy is highly dependent on the presence of a Bronchus sign on CT imaging: results from a prospective study. Chest 2010;138:1316-21. [PubMed]
- Larscheid RC, Thorpe PE, Scott WJ. Percutaneous transthoracic needle aspiration biopsy: a comprehensive review of its current role in the diagnosis and treatment of lung tumors. Chest 1998;114:704-9. [PubMed]
- Loubeyre P, McKee TA, Copercini M, et al. Diagnostic precision of image-guided multisampling core needle biopsy of suspected lymphomas in a primary care hospital. Br J Cancer 2009;100:1771-6. [PubMed]
- Loubeyre P, Copercini M, Dietrich PY. Percutaneous CT-guided multisampling core needle biopsy of thoracic lesions. AJR Am J Roentgenol 2005;185:1294-8. [PubMed]
- Pue CA, Pacht ER. Complications of fiberoptic bronchoscopy at a university hospital. Chest 1995;107:430-2. [PubMed]
- Shimizu K, Ikeda N, Tsuboi M, et al. Percutaneous CT-guided fine needle aspiration for lung cancer smaller than 2 cm and revealed by ground-glass opacity at CT. Lung Cancer 2006;51:173-9. [PubMed]
- Infante M, Lutman RF, Imparato S, et al. Differential diagnosis and management of focal ground-glass opacities. Eur Respir J 2009;33:821-7. [PubMed]
- Hur J, Lee HJ, Nam JE, et al. Diagnostic accuracy of CT fluoroscopy-guided needle aspiration biopsy of ground-glass opacity pulmonary lesions. AJR Am J Roentgenol 2009;192:629-34. [PubMed]
- Eberhardt R, Anantham D, Herth F, et al. Electromagnetic navigation diagnostic bronchoscopy in peripheral lung lesions. Chest 2007;131:1800-5. [PubMed]
- Ost DE, Ernst A, Lei X, et al. Diagnostic Yield and Complications of Bronchoscopy for Peripheral Lung Lesions. Results of the AQuIRE Registry. Am J Respir Crit Care Med 2016;193:68-77. [PubMed]
- Gould MK, Donington J, Lynch WR, et al. Evaluation of individuals with pulmonary nodules: when is it lung cancer? Diagnosis and management of lung cancer, 3rd ed: American College of Chest Physicians evidence-based clinical practice guidelines. Chest 2013;143:e93S-120S.