Predictors associated with mortality of extracorporeal life support therapy for acute heart failure: single-center experience with 679 patients
Introduction
Extracorporeal life support (ECLS) is the ultimate treatment option in patients with acute heart or respiratory failure refractory to conventional treatment. ECLS therapy, respectively veno-arterial extracorporeal membrane oxygenation (V-A ECMO) is used increasingly (1,2). Especially post-cardiotomy extracorporeal life support (PC-ECLS) has shown a remarkable increase (3). However, there is insufficient evidence regarding the benefits or harms of ECLS therapy in different patient collectives (4-8). This lack of studies was the limiting factor in a recent Cochrane analysis on the topic (9). Despite technical improvements and increasing experience, the mortality of patients undergoing ECLS therapy remains high. Recent studies and meta-analyses report highly variable mortality ranging from 35.0% to 76% (4,6,8,10-13). Clinical reality presents a heterogeneous patient collective requiring a complex diagnostic and therapeutic pathway. An increasing number of critically ill patients with unclear prognosis is supported with ECLS therapy. Withholding an ECLS installation is an ethical dilemma (1). Consequently, it is important to have more information about the course of ECLS therapy. This study describes a multivariable prediction model to gain further evidence with this emerging therapy option. We developed a comprehensive analysis on the outcome of ECLS patients over 13 years at the University Hospital Zurich in Switzerland. We present the following article in accordance with the TRIPOD reporting checklist (available at https://jtd.amegroups.com/article/view/10.21037/jtd-21-1770/rc).
Methods
The study was conducted in accordance with the Declaration of Helsinki (as revised in 2013). The study was approved and the requirement for written informed consent was waived by the Cantonal Ethics Commission of Zurich, Switzerland (BASEC-Nr. 2019-01926).
Study design
This retrospective, observational single-center study was conducted at the University Hospital Zurich in Switzerland, a tertiary care referral hospital and designated ECLS center. We included all adult patients treated with ECLS therapy between January 2007 and December 2019. Solely cases with veno-arterial (V-A) cardiocirculatory or cardiopulmonary support were considered. Data was extracted from the clinic-specific registers and the hospital-wide clinical information system through medical controlling. Exclusion criteria were age below 18 years and documented refusal of consent (Figure 1). We grouped indications for ECLS therapy in four common categories according to current literature (1,2,4): postcardiotomy, cardiopulmonary resuscitation, refractory cardiogenic shock, and other. Included in “postcardiotomy” group are ECLS indications of intraoperative weaning failure from cardiopulmonary bypass, and postoperative refractory cardiogenic shock. Patients treated with ECLS during cardiac arrest or immediate after return of spontaneous circulation (ROSC) are assigned to the group “cardiopulmonary resuscitation”. The category “other” included ECLS indications for lung transplantation and expansive thoracic surgery.
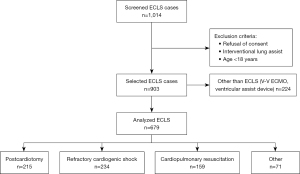
Study endpoints
This study aims to describe in-hospital mortality, to identify independent predictors associated with mortality and to analyze changes of mortality over time.
Data collection and variables
We manually reviewed the medical records of all included patients. Based on operative and intensive care unit reports, pre-defined variables were extracted of the corresponding cases (definition of variables in Table S1). Coding data and administrative data were saved and exported from SAP ERP (SAP AG, Walldorf, Germany). Business intelligent software Qlikview (QlikTech, Radnor, PA, USA) was a step-in data evaluation. The closest laboratory value before (T0) and after (T1) ECLS insertion was noted. We performed agreement analysis with Bland-Altman plots to determine the difference between T0 and T1 for each laboratory parameter. To minimize missing data, missing values of T0 were complemented with data from T1, because agreement analysis showed consistency. The variable “length of stay” was used to calculate the amount of transfusion parameters given per day. For this task, a length of stay value of 0 was replaced by 1 to avoid dividing by zero; 30-day survival was defined as 30-day survival after ECLS implantation.
ECLS insertion
At the beginning of the study period, peripheral cannulation for ECLS was performed surgically by cut-down to the common femoral artery. The arterial cannula was inserted via a graft sewed on to the artery. After percutaneous techniques became available, the standard procedure included cannulation of the common femoral artery with a perfusion limb through the superficial femoral artery. In both, surgical cut-down and percutaneous ECLS insertion, the venous cannula was placed through the common femoral vein in the right atrium, with the tip at the confluence of the superior vena cava. In central ECLS, the arterial cannula was inserted in the ascending aorta, and the venous cannula in the right atrium. The same cannulation strategy was applied for ECLS implantation in referring hospitals (14). At our institution, ECLS insertion is performed by cardiac surgeons with transesophageal echocardiographic guidance by a cardiac anesthetist.
Statistical analysis
For descriptive statistics, we show median and interquartile range (IQR) or mean and standard deviation (SD) for continuous variables. For categorical variables, we show counts and proportions. We checked if there is a relation between two categorical variables with Fisher’s exact test. Mann-Whitney test was used to compare two groups with respect to continuous data.
We used a multiple logistic regression model to assess the effect of possibly influential variables on the binary variable in-hospital mortality. The predictor variables were chosen for clinical reasons. There was no evidence of multicollinearity, as assessed by Spearman’s correlation. In order to capture potential changes over time, we included a cubic spline for the time variable in the model. The associated effect of time was shown in a graph. We calculated the within-sample receiver operating characteristic (ROC) curve to determine discrimination of our regression model. We plotted Kaplan-Meier estimates for duration of ECLS therapy per day for each indication group, where a termination of ECLS due to death was treated as a censoring event.
Hypothesis tests were 2-sided. A P value <0.05 was considered statistically significant. Analyses were done with R version 4.0.5 (15).
Results
We analysed 679 ECLS cases over 13 years. The patient’s median age was 60 years, and 27.5% were female. Most frequent comorbidities prior to ECLS insertion were coronary artery disease, congestive heart failure, and peripheral vascular disease. Specific differences in descriptive statistics between the four indications “postcardiotomy”, “refractory cardiogenic shock”, “cardiopulmonary resuscitation”, and “other” (e.g., lung transplantation and expansive thoracic surgery) for ECLS are shown in Table 1. Lung transplantations performed at the ECLS primarily represent the indication of “others” (58 out of 71 cases). The further 13 procedures are high-risk pulmonary or tracheal surgeries involving ECLS.
Table 1
ECLS therapy for | All indications (n=679) | Postcardiotomy (n=215) | Cardiopulmonary resuscitation (n=159) | Refractory cardiogenic shock (n=234) | Other indications (n=71) |
---|---|---|---|---|---|
Patient characteristics | |||||
Age (years) | 60.0 (49.0 to 69.0) | 64.0 (56.0 to 73.0) | 60.0 (48.0 to 68.0) | 56.0 (46.2 to 66.0) | 56.0 (43.5 to 61.0) |
BMI (kg/m²) | 25.7 (22.9 to 29.0) | 26.2 (23.5 to 29.8) | 26.0 (24.0 to 29.0) | 24.9 (22.8 to 28.5) | 24.1 (19.5 to 27.1) |
Sex (female) | 187 (27.5) | 56 (26.1) | 44 (27.7) | 65 (27.8) | 22 (31.0) |
SAPS II (points) (n=626) | 52.0 (40.0 to 71.0) | 53.0 (42.0 to 69.0) | 68.5 (47.0 to 82.0) | 50.5 (39.0 to 68.0) | 37.0 (29.0 to 50.8) |
Charlson comorbidity index | 3.0 (2.0 to 5.0) | 4.0 (3.0 to 5.2) | 3.0 (1.0 to 5.0) | 3.0 (1.0 to 5.0) | 2.0 (1.0 to 3.0) |
Transplantation | |||||
Heart | 21 (3.1) | 16 (7.4) | 1 (0.6) | 4 (1.7) | 0 (0.0) |
Lung | 68 (10.0) | 0 (0.0) | 1 (0.6) | 9 (3.9) | 58 (81.7) |
Baseline laboratory parameters | |||||
Lactate (mmol/L) | 6.6 (5.3) [83] | 8.9 (5.1) [27] | 8.3 (5.1) [11] | 4.8 (4.3) [42] | 1.2 (2.1) [3] |
Haemoglobin (g/L) | 102.5 (27.9) [28] | 87.8 (20.6) [2] | 110.6 (30.9) [9] | 108.0 (27.1) [17] | 112.9 (25.9) [0] |
Myoglobin (µg/L) | 2,031.6 (4,526.1) [31] | 1,968.3 (4,261.2) [9] | 3,321.8 (6,073.4) [3] | 1,550.4 (3,879.6) [10] | 733.7 (819.0) [9] |
Creatinine (µmol/L) | 133.6 (78.1) [10] | 132.5 (68.0) [5] | 135.3 (68.4) [2] | 151.9 (93.1) [2] | 73.2 (25.9) [1] |
Comorbidities | |||||
Coronary artery disease | 326 (48.0) | 112 (52.1) | 99 (62.3) | 106 (45.3) | 9 (12.7) |
Congestive heart failure | 243 (35.8) | 107 (49.8) | 41 (25.8) | 89 (38.2) | 6 (8.4) |
Peripheral vascular disease | 118 (17.4) | 3 (4.2) | 73 (34.0) | 20 (12.6) | 22 (9.4) |
Obstructive pulmonary disease | 55 (8.1) | 17 (7.9) | 7 (4.4) | 15 (6.4) | 16 (22.5) |
Diabetes mellitus | 97 (14.3) | 30 (13.9) | 26 (16.4) | 32 (13.7) | 9 (12.7) |
Chronic kidney disease | 53 (7.8) | 25 (11.6) | 9 (5.7) | 15 (6.4) | 4 (5.6) |
ECLS | |||||
External insertion† | 62 (9.1) | 2 (0.9) | 21 (13.2) | 39 (16.7) | 0 (0.0) |
Insertion technique | |||||
Seldinger peripheral | 445 (65.5) | 81 (37.7) | 135 (84.9) | 203 (86.8) | 26 (36.6) |
Surgical peripheral | 96 (14.1) | 41 (19.1) | 15 (9.4) | 31 (13.2) | 9 (12.7) |
Surgical central | 138 (20.3) | 93 (43.3) | 9 (5.7) | 0 (0.0) | 36 (50.7) |
Further cardiac support system | 83 (12.2) | 37 (17.2) | 22 (13.8) | 24 (10.3) | 0 (0.0) |
Intra-aortic balloon pump | 66 (9.7) | 34 (15.8) | 17 (10.7) | 15 (6.4) | 0 (0.0) |
Impella® | 17 (2.5) | 3 (1.4) | 5 (3.1) | 9 (3.9) | 0 (0.0) |
Successful weaning | 306 (45.1) | 76 (35.4) | 56 (35.2) | 107 (45.7) | 67 (94.4) |
Bridge to assist device | 53 (7.8) | 10 (4.7) | 8 (5.0) | 35 (15.0) | 0 (0.0) |
Bridge to transplantation | 7 (1.0) | 0 (0.0) | 1 (0.6) | 6 (2.6) | 0 (0.0) |
Mortality | |||||
In-hospital mortality | 377 (55.5) | 152 (70.7) | 108 (67.9) | 110 (47.0) | 7 (9.9) |
Death during ECLS therapy | 313 (46.1) | 129 (60.0) | 94 (59.1) | 86 (36.8) | 4 (5.6) |
In-hospital death after ECLS weaning | 64 (9.4) | 23 (10.7) | 14 (8.8) | 24 (10.2) | 3 (4.3) |
30-day survival | 316 (46.8) [4] | 71 (33.0) | 47 (29.7) [1] | 133 (57.6) [3] | 65 (91.5) |
Complications during ECLS therapy | |||||
Transfusions | |||||
Red blood cells (units) | 3.0 (1.0 to 5.0) | 3.0 (1.0 to 5.0) | 2.0 (1.0 to 4.0) | 4.0 (2.0 to 7.0) | 2.0 (1.0 to 5.0) |
4.3 (5.2) | 4.6 (6.6) | 2.8 (3.7) | 5.0 (4.6) | 4.1 (4.8) | |
Fresh frozen plasma (units) | 1.0 (0.0 to 1.0) | 1.0 (1.0 to 1.0) | 0.0 (0.0 to 1.0) | 0.0 (0.0 to 1.0) | 0.0 (0.0 to 0.0) |
0.8 (1.0) | 1.2 (1.0) | 0.6 (0.8) | 0.6 (1.0) | 0.3 (0.7) | |
Platelet concentrate (units) | 1.0 (0.0 to 2.0) | 2.0 (1.0 to 3.0) | 0.0 (0.0 to 2.0) | 1.0 (0.0 to 2.0) | 0.0 (0.0 to 0.0) |
1.5 (2.2) | 2.1 (2.2) | 1.2 (1.9) | 1.5 (2.3) | 0.5 (1.5) | |
Major bleeding | 171 (25.2) | 72 (33.5) | 33 (20.8) | 55 (23.5) | 11 (15.5) |
Sepsis | 99 (14.6) | 24 (11.2) | 16 (10.1) | 53 (22.6) | 6 (8.4) |
Intra-cranial bleeding | 25 (3.7) | 5 (2.3) | 6 (3.8) | 13 (5.6) | 1 (1.4) |
Stroke | 36 (5.3) | 16 (7.4) | 9 (5.7) | 11 (4.7) | 0 (0.0) |
Liver failure | 101 (14.9) | 48 (22.3) | 21 (13.2) | 31 (13.2) | 1 (1.4) |
Renal replacement therapy | 308 (45.4) | 114 (53.0) | 72 (45.6) | 108 (46.1) | 14 (19.7) |
Ischemia | |||||
Extremities | 91 (13.4) | 19 (8.8) | 25 (15.7) | 41 (17.5) | 6 (8.4) |
Intestinal | 48 (7.1) | 22 (10.3) | 9 (5.7) | 15 (6.4) | 2 (2.8) |
Laparotomy (bleeding, intestinal ischemia) | 51 (7.5) | 19 (8.8) | 11 (6.9) | 14 (6.0) | 7 (9.9) |
Open chest therapy | 119 (17.5) | 90 (41.9) | 12 (7.5) | 16 (6.8) | 1 (1.4) |
Duration | |||||
Length ECLS (days) | 5.0 (1.0 to 9.0) | 5.0 (1.0 to 8.0) | 4.0 (1.0 to 8.0) | 7.5 (4.0 to 13.0) | 0.0 (0.0 to 2.0) |
Length ICU (days) | 11.0 (3.0 to 23.0) | 10.0 (2.0 to 20.0) | 7.0 (2.0 to 16.0) | 15.0 (8.0 to 29.0) | 8.5 (3.0 to 30.0) |
Length of hospital stay (days) | 17.0 (6.0 to 37.0) | 14.0 (4.5 to 29.0) | 10.0 (3.0 to 22.5) | 22.0 (12.0 to 45.0) | 39.0 (31.0 to 61.5) |
Data presents as median and IQR or mean and SD. Categorical variables as number and percentage (%). If necessary, the deviating number indicates in parentheses [n]. †, ECLS insertion by University Hospital Zurich outreach team in another hospital before transfer to University Hospital Zurich for definitive care. ECLS, extracorporeal life support; BMI, body mass index; SAPS II, simplified acute physiology score II; ICU, intensive care unit; IQR, interquartile range; SD, standard deviation.
The incidences of out-of-hospital and in-hospital resuscitation was 40 respectively 119 out of total 159 cases in the group of “cardiopulmonary resuscitation”. Mean down time was 2 minutes (SD of 6.6 minutes, 12 missing cases). ROSC was observed in 108 cases after mean 39.7 minutes (SD of 33.6 minutes, 41 missing cases).
Type of cardiac surgery in the “postcardiotomy” group was coronary artery bypass grafting (CABG) (n=42; 19.5%), valve repair/replacement (n=28; 13.0%), combined (n=59; 27.4%), heart transplantation (n=17; 7.9%), aortic dissection (n=37; 17.2%), and others (n=32; 14.9%).
Major bleeding events occurred mostly in the postcardiotomy subgroup (33.5%), whereas the bleeding events in subgroups cardiopulmonary resuscitation and refractory cardiogenic shock were comparable (20.8% and 23.5%, respectively).
Median duration of ECLS support was 5 (IQR, 1.0–9.0) days. ECLS therapy was thereby shortest in the “other” group with 0 (0–2) days and longest for “refractory cardiogenic shock” with 7.5 (4–13) days. The Kaplan-Meier estimates of duration of ECLS therapy are shown in Figure 2.
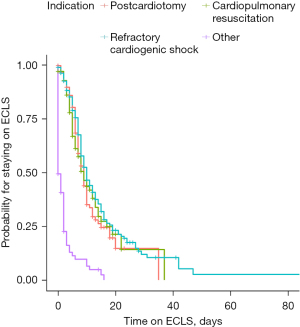
Successful weaning was achieved in 45.1% (306 out of 679 patients). 53 (7.8%) patients were bridged to an assist device and 7 (1.0%) directly to heart transplantation. Median length of hospital stay was 17 (IQR, 6.0–37.0) days.
Overall, in-hospital mortality of ECLS therapy was 55.5% (377 out of 679 patients). In-hospital mortality varied significantly between ECLS indications: 70.7% (152/215) for postcardiotomy, 67.9% (108/159) for cardiopulmonary resuscitation, 47.0% (110/234) for refractory cardiogenic shock, and 9.9% (7/71) for lung transplantation and expansive thoracic surgery (P<0.001) (Figure 3). Comparing in-hospital survival with 30-day survival shows a similar profile within the indications (Table 1).
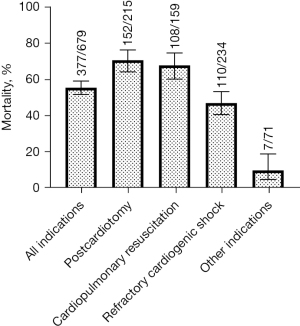
Regarding the cannulation strategy, considered over all cases, the distribution of survivors and non-survivors is comparable. Considering the specific subgroups, the more invasive the cannulation, the higher the mortality, especially for postcardiotomy (Table S2).
Figure 4 shows the predicted mortality stratified by the year of implantation. The results are based of the logistic regression model with the cubic spline. No evidence for a trend over time could be detected.
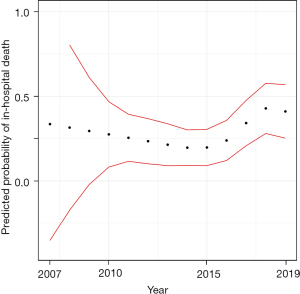
We entered variables of clinical interest into a multiple logistic regression model to identify associated predictors for in-hospital mortality (Table 2). Compared to ECLS for “refractory cardiogenic shock” (reference category), ECLS after “cardiopulmonary resuscitation” was associated with significantly higher mortality [odds ratio (OR) =2.25; 95% confidence interval (CI): 1.20–4.27; P=0.01]. Age and simplified acute physiology score (SAPS) II were also significant predictors. Notably, we found very strong evidence that each unit of any allogenic blood transfusions given per day was associated with a significantly higher mortality. Red blood cell transfusion with an OR of 1.68 (95% CI: 1.33–2.15; P<0.001), fresh frozen plasma transfusion with an OR of 2.37 (95% CI: 1.34–4.64; P=0.007), and platelet concentrate transfusion with an OR of 1.49 (95% CI: 1.15–2.02; P=0.005). Correspondingly, 52 out of 302 survivors (17.2%) and 119 out of 377 non-survivors (31.6%) experienced the adverse event “major bleeding”. Further, we determined new onset of liver failure to be associated with in-hospital mortality with an OR of 3.12 (95% CI: 1.50–6.78; P=0.003). The within-sample ROC curve for the logistic regression model showed excellent discrimination with an area under the curve (AUC) of 0.89 (95% CI: 0.87–0.92), (Figure S1). In a sub analysis we performed the logistic regression models for each indication separately (Tables S3-S5).
Table 2
Predictor variables | OR | 95% CI | P value |
---|---|---|---|
Intercept | 0.01 | 0.00 to 0.47 | 0.02 |
Age (per year) | 1.03 | 1.01 to 1.05 | 0.004 |
BMI (per kg/m²) | 0.99 | 0.95 to 1.03 | 0.68 |
SAPS II (per point) | 1.02 | 1.00 to 1.03 | 0.02 |
Baseline laboratory values | |||
Lactate (per mmol/L) | 1.02 | 0.97 to 1.09 | 0.43 |
Haemoglobin (per g/L) | 1.00 | 0.99 to 1.01 | 0.92 |
Myoglobin (per µg/L) | 1.00 | 1.00 to 1.00 | 0.06 |
Creatinine (per µmol/L) | 1.00 | 1.00 to 1.00 | 0.70 |
Indication | |||
Refractory cardiogenic shock | Reference | Reference | Reference |
Cardiopulmonary resuscitation | 2.25 | 1.20 to 4.27 | 0.01 |
Postcardiotomy | 1.12 | 0.56 to 2.19 | 0.75 |
Other | 0.45 | 0.10 to 1.53 | 0.24 |
Transfusions | |||
Red blood cells (unit/day) | 1.68 | 1.33 to 2.15 | <0.001 |
Fresh frozen plasma (unit/day) | 2.37 | 1.34 to 4.64 | 0.007 |
Platelet concentrate (unit/day) | 1.49 | 1.15 to 2.02 | 0.005 |
Liver failure | 3.12 | 1.50 to 6.78 | 0.003 |
Length ECLS (per day) | 1.02 | 0.99 to 1.06 | 0.23 |
ECLS, extracorporeal life support; OR, odds ratio; CI, confidence interval; BMI, body mass index; SAPS II, simplified acute physiology score II.
Discussion
In this retrospective study, we analyzed 679 ECLS cases over a 13-year period in a single tertiary care referring ECLS center. To the best of our knowledge, this is one of the largest single-center ECLS analysis reported so far.
We found a high in-hospital mortality in ECLS patients: 55.5% of all patients died. A German nationwide analysis of 22’687 V-A ECMO runs described a similar but slightly higher hospital mortality of 65.6% (11). One reason for the difference may be the use of ECLS for lung transplantation and expansive thoracic surgery in our cohort. These surgeries are summarized in our “other” indications group, which showed the lowest mortality (9.9%). The distinct risk profile of patients in the “other” group may also explain the shorter duration of ECLS therapy, as compared to the remaining three groups in the Kaplan-Meier estimates.
In-hospital mortality during or after ECLS therapy differed significantly between the reported indications. This knowledge will help as a rationale for decision making in future ECLS cases. High mortality was observed for postcardiotomy (70.7%) and related to cardiopulmonary resuscitation (67.9%). Whereby the subgroup “out-of hospital” cardiopulmonary resuscitation shows the distinct highest mortality rate (85%). These results are in line with a recent meta-analysis (4). Here, highest mortality was observed on post cardiac arrest ECLS treatment (64–76%, depending on out-of or in-hospital cardiac arrest) as well as postcardiotomy (60%). There are some difficulties in comparing mortality rates for different ECLS indications between studies. Notably, there is no consensus on predefined indication groups (16). In our study, we decided on four groups. Three of them are widely used in current literature [postcardiotomy (3,10,17,18), cardiopulmonary resuscitation (19,20) and refractory cardiogenic shock (12,21)]. We added the fourth group (“other”) to account for specific indications for ECLS use that cannot be integrated into the three main groups considering the whole ECLS cohort at our institution between 2007 and 2019.
Interestingly, there was no significant change of in-hospital mortality during our observation period. To account for a possible non-linear change, we used cubic spline interpolation in the logistic regression model, as shown in Figure 4. A slight increase of in-hospital mortality in recent years can be seen, however, there are not enough values in the earlier years to be able to show a difference, as can be seen by the broader CIs in the years before 2010. We can see from the plot that there is no evidence for an effect of time on mortality. We would have expected a mortality reduction over the years, due to the gained knowledge and improvement in the ECLS treatment. The inclusion of higher risk patients in the recent years may have contributed to the lack of reduction in mortality. This observation is in line with comprehensive analyses of post-cardiotomy ECLS (3,13). Further sub-group analysis for primary graft dysfunction after heart transplantation (22) or distinct focus on ECLS after CABG shows a high mortality, too (23).
We identified specific risk predictors for in-hospital mortality in the multiple logistic regression model (Table 2). Increasing age and SAPS II were associated with higher mortality. Advanced age is a well-known risk factor (10,18,21), also revealed in a prospective cohort analysis (24). In particular, patients older than 70 years are at highest risk of not surviving to discharge (13). The SAPS II is a widely used ICU risk predictor and has been shown to be also associated with ECLS mortality, although with poor discrimination (25,26). ECLS after “cardiopulmonary resuscitation” was associated with significant higher mortality compared to ECLS for “refractory cardiogenic shock”. The sub-group analysis of Alba et al. (4) for ECMO etiology showed significantly different short-term mortality across groups (e.g., out-of-hospital cardiac arrest, heart failure, postcardiotomy), also by multivariable metaregression after adjusting for age, sex, and recruitment time frame.
We also found a new manifestation of liver failure during ECLS therapy to be associated with higher in-hospital mortality. Liver failure as an independent risk factor for mortality has previously not been described in larger studies and is supported by only limited literature regarding this topic. Altered liver parameters during ECLS therapy are known (27). The MELD-XI score is predictive of mortality in ECLS (28,29). In case of liver failure, molecular adsorbent recirculating system (MARS) therapy has been described in patients on ECLS for safe and accelerated recovery of liver function and improved survival to wean from ECLS (30).
In our regression analysis, the number of transfused allogenic blood products is considerably associated with mortality. This finding is in line with the association of transfusion of allogeneic blood products and increased morbidity and mortality (31). The univariate analysis of Guimbretière et al. (32) reported a prognostic impact of blood product transfusion for ECLS therapy. A dose-dependent relationship between the amount of transfused red blood cells (33) respectively the total of red blood cell units received during ECLS (34) was associated with in-hospital mortality and adverse outcomes (35). A recent systematic review suggested that a restrictive transfusion threshold in ECLS management provides favorable outcomes in single-center cohorts (36). Allogeneic blood transfusion and bleeding complications may have some co-linearity. In an early analysis of our study, we interestingly found that bleeding complication during ECLS therapy was not an independent mortality predictor. In contrast, allogeneic blood transfusions were independently associated with in-hospital mortality in our multivariate model. This indicates that there may be additional factors regarding allogenic blood transfusions apart from the bleeding complication, that are worsening patient’s outcome.
We chose the predictor variables in our regression model as variables of interest for clinical reasons. Within-sample ROC analysis validates our choice by showing excellent discrimination of our regression model (AUC 0.89, Figure S1). This corresponds to discrimination of risk scores designed specifically for predicting ECLS mortality (6,26). As our study was primarily an exploratory study, we did not split the data set in a training and a test set for the calculation of the ROC and the AUC. In future studies, these discriminatory abilities should be validated using a new data set that was not used to train the model.
This study complements the existing heterogeneous data situation. We confirmed previously described predictors such as age and SAPS II score. Moreover, we found additional parameters with impact on in-hospital mortality, such as liver failure and transfusion of allogenic blood products. Our results emphasize the importance of risk stratification and ongoing evaluation of organ function during ECLS therapy. These results offer new therapeutic approaches for improving outcomes in ECLS therapy. Further, the left ventricular distension under ECLS therapy must be considered and direct venting is a favorable strategy (37). While the prevention of ICU associated liver failure may be difficult, alternatives to blood transfusions exist. Patient Blood Management optimizes the patient’s own blood volume, implements blood loss sparing surgery techniques and advocates factor-based coagulation management (31,38). These strategies have been shown to be beneficial in many aspects of perioperative medicine, as well as intensive care medicine (39).
Retrospective studies provide evidence of possible causal relationships, in some cases compelling, but they cannot provide definitive proof. Randomized studies are challenging and currently ongoing. A consensus on predefined indication groups as well as outcomes would greatly benefit further studies in the field. The study was conducted over 13 years, along with technological and process improvements over this period, which causes a considerable bias. However, risk factors that are significantly associated with mortality over this long period appear to have a substantial influence. In our multivariate analysis we focused on variables which are not already known as independent mortality predictors of ECLS therapy. In the future, it would be very valuable to conduct a prospective study to further develop and especially validate a prediction model for (non-) favorable outcomes.
Conclusions
In-hospital mortality of patients treated with ECLS therapy is high which has not changed in the observed period. However, there are strong predictors associated with in-hospital mortality at the time of ECLS insertion but also during ECLS therapy. Knowledge of these predictors may affect future decisions about ECLS indications and the respective management to use this elaborate therapy more effectively with favorable outcomes.
Acknowledgments
Funding: None.
Footnote
Reporting Checklist: The authors have completed the TRIPOD reporting checklist. Available at https://jtd.amegroups.com/article/view/10.21037/jtd-21-1770/rc
Data Sharing Statement: Available at https://jtd.amegroups.com/article/view/10.21037/jtd-21-1770/dss
Conflicts of Interest: All authors have completed the ICMJE uniform disclosure form (available at https://jtd.amegroups.com/article/view/10.21037/jtd-21-1770/coif). AK received honoraria for lecturing from Bayer AG Switzerland. DRS’s academic department is receiving grant support from the Swiss National Science Foundation, Berne, Switzerland, the Swiss Society of Anesthesiology and Reanimation (SGAR), Berne, Switzerland, the Swiss Foundation for Anesthesia Research, Zurich, Switzerland, Vifor SA, Villars-sur-Glâne, Switzerland and Vifor (International) AG, St. Gallen, Switzerland. DRS is Co-Chair of the ABC-Trauma Faculty, sponsored by unrestricted educational grants from Novo Nordisk Health Care AG, Zurich, Switzerland, CSL Behring GmbH, Marburg, Germany, LFB Biomédicaments, Courtaboeuf Cedex, France and Octapharma AG, Lachen, Switzerland. DRS received honoraria/travel support for consulting or lecturing from: Danube University of Krems, Austria, US Department of Defense, Washington, USA, European Society of Anesthesiology, Brussels, BE, Korean Society for Patient Blood Management, Seoul, Korea, Korean Society of Anesthesiologists, Seoul, Korea, Network for the Advancement of Patient Blood Management, Haemostasis and Thrombosis, Paris, France, Alexion Pharmaceuticals Inc., Boston, MA, Baxalta Switzerland AG, Volketswil, Switzerland, Bayer AG, Zürich, Switzerland, B. Braun Melsungen AG, Melsungen, Germany, Boehringer Ingelheim GmbH, Basel, Switzerland, Bristol-Myers-Squibb, Rueil-Malmaison Cedex, France and Baar, Switzerland, CSL Behring GmbH, Hattersheim am Main, Germany and Berne, Switzerland, Celgene International II Sàrl, Couvet, Switzerland, Daiichi Sankyo AG, Thalwil, Switzerland, Haemonetics, Braintree, MA, USA, Instrumentation Laboratory (Werfen), Bedford, MA, USA, LFB Biomédicaments, Courtaboeuf Cedex, France, Merck Sharp & Dohme, Kenilworth, New Jersey, USA, Novo Nordisk Health Care AG, Zurich, Switzerland, PAION Deutschland GmbH, Aachen, Germany, Pharmacosmos A/S, Holbaek, Denmark, Pfizer AG, Zürich, Switzerland, Pierre Fabre Pharma, Alschwil, Switzerland, Portola Schweiz GmbH, Aarau, Switzerland, Roche Diagnostics International Ltd, Reinach, Switzerland, Sarstedt AG & Co., Sevelen, Switzerland and Nümbrecht, Germany, Shire Switzerland GmbH, Zug, Switzerland, Tem International GmbH, Munich, Germany, Vifor Pharma, Munich, Germany, Neuilly sur Seine, France and Villars-sur-Glâne, Switzerland, Vifor (International) AG, St. Gallen, Switzerland, Zuellig Pharma Holdings, Singapore, Singapore. FM reports grant and/or research institutional support from Abbott, Medtronic, Edwards Lifesciences, Biotronik, Boston Scientific Corporation, NVT and Terumo; consulting fees, personal and institutional honoraria from Abbott, Medtronic, Edwards Lifesciences, Xeltis, Cardiovalve, Occlufit, Simulands and Occlufit; royalty income/IP rights from Edwards Lifesciences. FM is Shareholder (including share options) of Cardiogard, Magenta, SwissVortex, Transseptalsolutions, Occlufit, 4Tech and Perifect. MJW received speaker’s honoraria and travel grants from Berlin Heart GmbH, Berlin, Germany. The other authors have no conflicts of interest to declare.
Ethical Statement: The authors are accountable for all aspects of the work in ensuring that questions related to the accuracy or integrity of any part of the work are appropriately investigated and resolved. The study was conducted in accordance with the Declaration of Helsinki (as revised in 2013). The study was approved and the requirement for written informed consent was waived by the Cantonal Ethics Commission of Zurich, Switzerland (BASEC-Nr. 2019-01926).
Open Access Statement: This is an Open Access article distributed in accordance with the Creative Commons Attribution-NonCommercial-NoDerivs 4.0 International License (CC BY-NC-ND 4.0), which permits the non-commercial replication and distribution of the article with the strict proviso that no changes or edits are made and the original work is properly cited (including links to both the formal publication through the relevant DOI and the license). See: https://creativecommons.org/licenses/by-nc-nd/4.0/.
References
- Guglin M, Zucker MJ, Bazan VM, et al. Venoarterial ECMO for Adults: JACC Scientific Expert Panel. J Am Coll Cardiol 2019;73:698-716. [Crossref] [PubMed]
- Pineton de Chambrun M, Bréchot N, Combes A. Venoarterial extracorporeal membrane oxygenation in cardiogenic shock: indications, mode of operation, and current evidence. Curr Opin Crit Care 2019;25:397-402. [Crossref] [PubMed]
- Lorusso R, Whitman G, Milojevic M, et al. 2020 EACTS/ELSO/STS/AATS Expert Consensus on Post-Cardiotomy Extracorporeal Life Support in Adult Patients. Ann Thorac Surg 2021;111:327-69. [Crossref] [PubMed]
- Alba AC, Foroutan F, Buchan TA, et al. Mortality in patients with cardiogenic shock supported with VA ECMO: A systematic review and meta-analysis evaluating the impact of etiology on 29,289 patients. J Heart Lung Transplant 2021;40:260-8. [Crossref] [PubMed]
- Kaushal M, Schwartz J, Gupta N, et al. Patient Demographics and Extracorporeal Membranous Oxygenation (ECMO)-Related Complications Associated With Survival to Discharge or 30-Day Survival in Adult Patients Receiving Venoarterial (VA) and Venovenous (VV) ECMO in a Quaternary Care Urban Center. J Cardiothorac Vasc Anesth 2019;33:910-7. [Crossref] [PubMed]
- Schmidt M, Burrell A, Roberts L, et al. Predicting survival after ECMO for refractory cardiogenic shock: the survival after veno-arterial-ECMO (SAVE)-score. Eur Heart J 2015;36:2246-56. [Crossref] [PubMed]
- Vigneshwar NG, Kohtz PD, Lucas MT, et al. Clinical predictors of in-hospital mortality in venoarterial extracorporeal membrane oxygenation. J Card Surg 2020;35:2512-21. [Crossref] [PubMed]
- Wang L, Yang F, Wang X, et al. Predicting mortality in patients undergoing VA-ECMO after coronary artery bypass grafting: the REMEMBER score. Crit Care 2019;23:11. [Crossref] [PubMed]
- Tramm R, Ilic D, Davies AR, et al. Extracorporeal membrane oxygenation for critically ill adults. Cochrane Database Syst Rev 2015;1:CD010381. [Crossref] [PubMed]
- Biancari F, Perrotti A, Dalén M, et al. Meta-Analysis of the Outcome After Postcardiotomy Venoarterial Extracorporeal Membrane Oxygenation in Adult Patients. J Cardiothorac Vasc Anesth 2018;32:1175-82. [Crossref] [PubMed]
- Friedrichson B, Mutlak H, Zacharowski K, et al. Insight into ECMO, mortality and ARDS: a nationwide analysis of 45,647 ECMO runs. Crit Care 2021;25:38. [Crossref] [PubMed]
- Wilson-Smith AR, Bogdanova Y, Roydhouse S, et al. Outcomes of venoarterial extracorporeal membrane oxygenation for refractory cardiogenic shock: systematic review and meta-analysis. Ann Cardiothorac Surg 2019;8:1-8. [Crossref] [PubMed]
- Kowalewski M, Zieliński K, Brodie D, et al. Venoarterial Extracorporeal Membrane Oxygenation for Postcardiotomy Shock-Analysis of the Extracorporeal Life Support Organization Registry. Crit Care Med 2021;49:1107-17. [Crossref] [PubMed]
- Wilhelm MJ, Inderbitzin DT, Reser D, et al. Outcome of inter-hospital transfer of patients on extracorporeal membrane oxygenation in Switzerland. Swiss Med Wkly 2019;149:w20054. [Crossref] [PubMed]
- R Core Team. R: A language and environment for statistical computing. Vienna: R Foundation for Statistical Computing, 2019. Available online: https://www.R-project.org
- Extracorporeal Life Support Organization. ELSO Guidelines for Cardiopulmonary Extracorporeal Life Support. Version 1.4. Ann Arbor, MI, USA: ELSO, 2017.
- Harahwa T, Chor CYT, Harky A. The use of extracorporeal membrane oxygenation postcardiotomy-A systematic review. J Card Surg 2020;35:1941-53. [Crossref] [PubMed]
- Wang L, Wang H, Hou X. Clinical Outcomes of Adult Patients Who Receive Extracorporeal Membrane Oxygenation for Postcardiotomy Cardiogenic Shock: A Systematic Review and Meta-Analysis. J Cardiothorac Vasc Anesth 2018;32:2087-93. [Crossref] [PubMed]
- Ouweneel DM, Schotborgh JV, Limpens J, et al. Extracorporeal life support during cardiac arrest and cardiogenic shock: a systematic review and meta-analysis. Intensive Care Med 2016;42:1922-34. [Crossref] [PubMed]
- Xie A, Phan K, Tsai YC, et al. Venoarterial extracorporeal membrane oxygenation for cardiogenic shock and cardiac arrest: a meta-analysis. J Cardiothorac Vasc Anesth 2015;29:637-45. [Crossref] [PubMed]
- Khorsandi M, Dougherty S, Bouamra O, et al. Extra-corporeal membrane oxygenation for refractory cardiogenic shock after adult cardiac surgery: a systematic review and meta-analysis. J Cardiothorac Surg 2017;12:55. [Crossref] [PubMed]
- Jacob S, Lima B, Gonzalez-Stawinski GV, et al. Extracorporeal membrane oxygenation as a salvage therapy for patients with severe primary graft dysfunction after heart transplant. Clin Transplant 2019;33:e13538. [Crossref] [PubMed]
- Shao J, Wang L, Wang H, et al. Predictors for unsuccessful weaning from venoarterial extracorporeal membrane oxygenation in patients undergoing coronary artery bypass grafting. Perfusion 2020;35:598-607. [Crossref] [PubMed]
- Bonacchi M, Cabrucci F, Bugetti M, et al. Outcomes' predictors in Post-Cardiac Surgery Extracorporeal Life Support. An observational prospective cohort study. Int J Surg 2020;82:56-63. [Crossref] [PubMed]
- Lee HS, Kim HS, Lee SH, et al. Clinical implications of the initial SAPS II in veno-arterial extracorporeal oxygenation. J Thorac Dis 2019;11:68-83. [Crossref] [PubMed]
- Muller G, Flecher E, Lebreton G, et al. The ENCOURAGE mortality risk score and analysis of long-term outcomes after VA-ECMO for acute myocardial infarction with cardiogenic shock. Intensive Care Med 2016;42:370-8. [Crossref] [PubMed]
- Masha L, Peerbhai S, Boone D, et al. Yellow Means Caution: Correlations Between Liver Injury and Mortality with the Use of VA-ECMO. ASAIO J 2019;65:812-8. [Crossref] [PubMed]
- Ayers B, Wood K, Melvin A, et al. MELD-XI is predictive of mortality in venoarterial extracorporeal membrane oxygenation. J Card Surg 2020;35:1275-82. [Crossref] [PubMed]
- Sern Lim H. Baseline MELD-XI score and outcome from veno-arterial extracorporeal membrane oxygenation support for acute decompensated heart failure. Eur Heart J Acute Cardiovasc Care 2016;5:82-8. [Crossref] [PubMed]
- Sparks BE, Cavarocchi NC, Hirose H. Extracorporeal membrane oxygenation with multiple-organ failure: Can molecular adsorbent recirculating system therapy improve survival? J Heart Lung Transplant 2017;36:71-6. [Crossref] [PubMed]
- Spahn DR, Goodnough LT. Alternatives to blood transfusion. Lancet 2013;381:1855-65. [Crossref] [PubMed]
- Guimbretière G, Anselmi A, Roisne A, et al. Prognostic impact of blood product transfusion in VA and VV ECMO. Perfusion 2019;34:246-53. [Crossref] [PubMed]
- Chen FT, Chen SW, Wu VC, et al. Impact of massive blood transfusion during adult extracorporeal membrane oxygenation support on long-term outcomes: a nationwide cohort study in Taiwan. BMJ Open 2020;10:e035486. [Crossref] [PubMed]
- Aubron C, Cheng AC, Pilcher D, et al. Factors associated with outcomes of patients on extracorporeal membrane oxygenation support: a 5-year cohort study. Crit Care 2013;17:R73. [Crossref] [PubMed]
- Qin CX, Yesantharao LV, Merkel KR, et al. Blood Utilization and Clinical Outcomes in Extracorporeal Membrane Oxygenation Patients. Anesth Analg 2020;131:901-8. [Crossref] [PubMed]
- Abbasciano RG, Yusuff H, Vlaar APJ, et al. Blood Transfusion Threshold in Patients Receiving Extracorporeal Membrane Oxygenation Support for Cardiac and Respiratory Failure-A Systematic Review and Meta-Analysis. J Cardiothorac Vasc Anesth 2021;35:1192-202. [Crossref] [PubMed]
- Cevasco M, Takayama H, Ando M, et al. Left ventricular distension and venting strategies for patients on venoarterial extracorporeal membrane oxygenation. J Thorac Dis 2019;11:1676-83. [Crossref] [PubMed]
- Spahn DR, Muñoz M, Klein AA, et al. Patient Blood Management: Effectiveness and Future Potential. Anesthesiology 2020;133:212-22. [Crossref] [PubMed]
- Shander A, Javidroozi M, Lobel G. Patient Blood Management in the Intensive Care Unit. Transfus Med Rev 2017;31:264-71. [Crossref] [PubMed]