Serial evaluation of segmental esophageal reconstruction using a polyurethane scaffold in a pig model
Introduction
Esophageal defects ensuing from surgical resection of diseased tissue are rarely amenable to primary repair, and surgical reconstruction with an autologous conduit like stomach, small bowel, or colon is often required to restore gastrointestinal luminal continuity. Overall, esophagectomy is a life-threatening procedure, with a 30-day mortality rate up to 4.2% (1,2) and overall major morbidity rate of 33.1%, reflecting the highest complication rate of any commonly performed thoracic surgical procedure (3). Eliminating use of autologous conduits in favor of bioengineered constructs has potential to reduce mortality and improve surgical outcomes.
Ideally, a graft substitute would be available for use without associated concomitant morbidity risk from autologous conduit reconstruction. Though the esophagus has a relatively simplistic function, the structural anatomy and layered architecture prove inherently difficult to replicate. The use of synthetic or biologic scaffolds, sometimes in combination with numerous cell sources, has been evaluated in animal models as a full thickness, circumferential esophageal substitute (4-20). Though a number of large animal studies report esophageal regeneration with biomaterial replacement (5,6,9,10,14,16,17,19,21), no reliable product has emerged for use in lieu of current reconstructive surgical techniques. There is minimal use of biologic scaffolds for esophageal replacement in human patients, with limited case reports (22-28). Dua et al. first described the use of ECM for full circumferential esophageal defect repair in 2016 (24), however, there is still no approved product for clinical use.
In this study, the use of a synthetic electrospun polyurethane (PU) tubular scaffold for thoracic esophageal replacement is evaluated. This model has been evaluated for efficacy in our laboratory with results demonstrating that circumferential replacement of a 5 cm thoracic esophageal defect was well tolerated in pigs and esophageal healing observed (9,14). The aim of this study is to (I) investigate whether a PU matrix could augment esophageal healing over that of stent alone; (II) evaluate the healing response across a larger 10 cm defect; and (III) determine if there is restoration of esophageal function over time. We present the following article in accordance with the ARRIVE reporting checklist (available at https://jtd.amegroups.com/article/view/10.21037/jtd-21-1559/rc).
Methods
Synthetic scaffold electrospinning
To fabricate the scaffold, a 5 wt% polyurethane (DSM, Netherlands) in hexafluoroisopropanol (Oakwood Chemical, USA) solution was prepared and solution components sealed in Erlenmeyer flasks and mixed, prior to loading into a syringe pump (Harvard Apparatus, USA) at a rate of 5 mL/h. A thin layer of glycerin gel was applied to a stainless-steel cylindrical collector coated with kosher salt and mounted to a custom stage. A pulley was attached and connected to a rotating motor at ~100 rpm. The collector assembly was mounted over the center of the collector and filled with additional salt, which was deposited over the collector for the duration of spins. The loaded syringe pump(s) was placed in front of the collector and the distance from needles to collector recorded. Positive and negative high voltage power supplies (Glassman High Voltage, USA) were wired to the syringe tips and collector, respectively. After completion of electrospinning, the scaffold was removed and placed into warm water for 10 min to remove salt crystals, and repeated four times. Scaffolds were then placed in a vacuum chamber for 24 h to remove residual water, and cut to size (6.5 or 13 cm), plasma treated, and exposed to 3,500 J/cm2 of UV radiation. The prepared tubular scaffold (Figure 1A) product is a medical grade implant suitable for use in vivo.
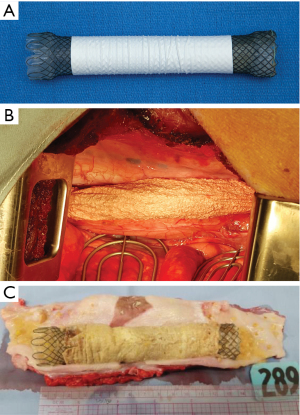
Transplantation of polyurethane matrix
This study was conducted in accordance with Mayo Clinic Institutional Animal Care and Use Committee (IACUC). Experiments were performed under protocol number A25614 and granted by Mayo Clinic IACUC in compliance with AVMA guidelines for the care and use of animals. Both male and female Yucatan (long-term) and domestic (short-term) pigs were utilized. All animals arrived at the Mayo Institute Hills Research Facility, received a veterinary examination, were administered proper vaccinations when applicable, and were quarantined/acclimatized for a minimum of 5 days. Animals were housed on Tenderfoot flooring in temperature and humidity-controlled rooms with 12-h light/dark cycles and all cages power washed daily. Animals were observed by veterinary technicians twice daily and laboratory personnel remained constant for the duration of the study. Animals were almost exclusively fed by laboratory staff, with weekly weight gain used to modify dietary intake accordingly. Animals were group housed when possible to encourage social behavior, and animals were exercised on a regular basis outside of their home kennel. All animals were provided with large safe enrichments in the form of hanging chains with plastic attachments, and free moving dumbbells and footballs. If not on liquid diet, animals were offered dietary snacks under supervision. Study groups consisted of 5 cm (n=4) or 10 cm (n=6) defects bridged with scaffold, and 5 cm stent only control pigs (n=2). Animals with 10 cm defects were sacrificed at 14 (n=3) or 60 days (n=3), and those with 5 cm defects were sacrificed at 73, 269, 378 and 399 days, or at 30 days (n=2) in control animals. Animals were euthanized at study endpoints (n=10), or based on humane endpoint criteria (n=2) like inability to eat or drink, excessive weight loss, or irreparable esophageal stenosis, as outlined in the IACUC protocol.
Animals were fasted and induced using xylazine (2 mg/kg, VetOne, Boise, ID, USA) and telazol (5 mg/kg, Zoetis, Parsippany-Troy Hills, NJ, USA) and maintained under general anesthesia using inhaled isoflurane. Perioperative (Cefazolin, 25 mg/kg, Novaplus, Lake Zurich, IL, USA) and long-acting antibiotics (Fluorphenicol, 15 mg/kg, Intervet (Merck), Kenilworth, NJ, USA) and non-steroidal anti-inflammatory (Carprofen, 4 mg/kg, SQ, Zoetis, Parsippany-Troy Hills, NJ, USA) and delayed release analgesic drugs (Buprenorphine-SR, 0.18 mg/kg, Zoopharm, Laramie, WY, USA) were administered peri-operatively. Pre-operative endoscopy was performed (GIF, Olympus, Japan) and a right thoracotomy performed at the fifth intercostal space. The esophagus was transected and no esophageal tissue resected in animals where transected ends naturally contracted forming a 5 cm gap (9,14). To create 10 cm defects, 4 cm of tissue was resected. A tubular PU graft was placed with oral and aboral anastomoses performed in simple continuous fashion using 4-0 PDS to bridge defects, and gap length was physically measured based on bridging scaffold length once ends were anastomosed. A WallFlex (12 cm, Boston Scientific, Natick, MA, USA) covered stent was deployed and secured with aid of direct transthoracic visualization inside the scaffold to maintain luminal patency (Figure 1A,1B). In stent only animals, the guide wire was deployed prior to esophageal transection, and subsequently the stent was placed. Hemoclips were placed surrounding the lesion both on esophageal and mediastinal tissue of long-term animals. The lung was allowed to expand and a single tube thoracostomy placed during closure only. Animals were on full liquid (Ensure Plus Vanilla, Abbott Laboratories, Columbus, OH, USA) diet for two days then supplemented with wet canine food (Purina Pro Plan Critical Nutrition Diet, St. Louis, MO, USA). In long-term animals, soaked pig chow slurry was gradually introduced subsequent to stent removal, progressing eventually to a completely dry diet. When on liquid diet, fecal output was observed to monitor for evidence of constipation, and fiber was supplemented as needed.
Histology
For short-term animals, the esophagus was longitudinally transected and stent removed prior to fixation (Figure 1C). Tissue was then trimmed and specimens acquired encompassing both native esophagus and the healing region. For long-term animals, the esophagus was examined with a veterinary pathologist (JB) and regions sectioned after en bloc fixation. Tissue sections were processed routinely, and paraffin embedded to prepare 5 µm sections. H&E and Masson’s Trichrome stains were used to semi-quantitatively assess the defect area and reviewed by a veterinary pathologist (JB).
Endoscopy
Esophageal endoscopy was performed at terminal endpoints in short-term (n=8), and at stent removal in long-term animals. For long-term animals, the stent was removed at 78–93 days, with concurrent endoscopy performed to validate tissue healing at the defect site. Following stent removal, endoscopy was performed at regular intervals, or earlier if complications such as difficulty eating or regurgitation arose, with intervention pursued. If complications arose that could not be treated effectively within the confines of IACUC protocol, the animals were humanely euthanized.
Barium swallow
Fluoroscopy ((Siemens Siremobil C-Arm, Siemens Medical Solutions USA Inc., Malvern, PA, USA), was performed in conjunction with barium administration to evaluate esophageal function in clicker trained, sling-restrained (Lomir Biomedical Inc., Malone, NY, USA) pigs at pre-operative baseline (n=2), and at 6 (n=2), 10 (n=2), 12 months (n=2) post-operatively in long-term study animals. Barium suspension (Barium E-Z-HD, 98% w/w, Bracco Diagnostics Inc., NJ, USA) was delivered at a 60% concentration via liquid bolus and at 98% concentration via wet food bolus administration. Barium paste was used in conjunction with cookie wafers (Fig Newton, Nabisco; Varibar Pudding Oral Paste, 40% w/v, Bracco Diagnostics Inc., NJ, USA).
Statistical analysis
All data are qualitatively reported from representative histological sections and from imaging modalities. No statistical techniques were employed in data interpretation.
Results
Graft transplantation into pig model
An unseeded PU graft was successfully implanted to bridge 5 or 10 cm full thickness esophageal defects. One animal was noted to have vomiting and inappetence at 14 days, with a fistula from the chest wall to repair area noted. Half of the long-term group survived to 12 and 13 months, however, two animals succumbed to stricture. One animal was inappetent and vomited the stent at 42 days post-operatively. Intervention was pursued, but restricture occurred. The second animal succumbed to stricture after initial stent removal. Bouginage was performed several times and the stent ultimately replaced for 2 months, however, repeat stricture occurred with stent removal and the animal was euthanized.
Histological examination
At 14 and 60 days, the tissue grossly had two distinguishable regions, notably an epithelialized region adjacent to a variably sized and centralized region with ulcerated and denuded epithelium (Figure 2A,2B). In control animals, there was partial epithelialization, but full integrity of the luminal structure. In surviving long-term animals without stricture, identification of the operated region was challenging grossly, but apparent (Figure 2C). Small diverticuli were seen and a small, scarred landmark served to identify the operated region, with thinning of the defect area. A significant luminal narrowing was obvious in strictured animals when longitudinally transected.
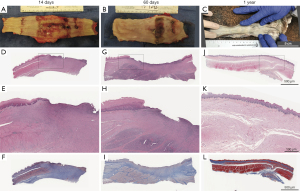
Histological evaluation of sections along the length of repaired and healing area of short-term specimens correlates to the central ulcerated region seen grossly with an area of non-epithelialized tissue in the middle of the defect zone, and likely the remnant region where esophageal ends did not appose after secondary wound contraction. Organizing fibrous connective tissue could be appreciated extending to the deeper adventitial layer and substantial inflammation could be appreciated in all animals, with variable fibrous lung adhesion. In control animals, re-epithelialization was present though variable. There was regional ulceration noted with fibroplasia seen in addition to superficial bacterial colonization. The extent of fibrosis was transmural with collagen separating muscle bundles and extending to adventitia (Figure 2D-2I).
At the site of surgical repair in long-term animals, there was flattening of squamous epithelium in regions of re-epithelialization and regional, lymphoplasmacytic and eosinophilic esophagitis. Less organized smooth muscle with intermingled scar and disorganization of mural smooth muscle was noted beneath the mucosal layer. Islands of muscle, not contiguous with muscle layers from native esophageal edges, were organized in small bundles surrounded by excessive fibrous connective tissue (Figure 2J-2L). The haphazard arrangement of smooth muscle likely results from wound contraction and scar formation approximating muscle bundles together, rather than newly regenerated muscle. Though healing occurred across the defect, the repaired tissue does not mimic architecturally normal tissue and there is an approximately 1cm scarred region, in contrast to the original 5 cm surgical defect.
Endoscopic evaluation
Endoscopy was performed prior to explant in short-term animals at 60 days and the scope passed easily between the stent and esophageal wall, revealing trapped debris/foodstuff. After stent removal at 78 and 93 days post-operatively in the two surviving long-term animals, endoscopy revealed complete epithelialization across the defect at 120 days. Both animals had evidence of small diverticulum formation first noted one month after stent removal at the proximal end of the repair, presenting as a shallow clefted region either within or near the healed region, with slight deepening of the clefts over time (Figure 3A,3B). One pig remained asymptomatic and transitioned to regular chow diet. Dysphagia and regurgitation associated with feeding dry food persisted for 10 months in the other animal, but she tolerated moistened chow mash and transitioned to dry diet for 2 months before study completion. At 12 months, there was mild rostral narrowing with formation of a small shelf on endoscopy (Figure 3C,3D), with reduced motility noted aboral to the rostral margin of repair, and very reduced motility across the grafted region. Motility could be observed caudal to the repaired region and on entry into the stomach.
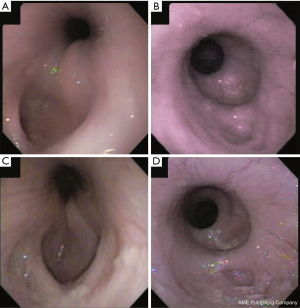
Barium esophagram
Fluoroscopic barium esophagram was successful in sling-restrained pigs with no complications from oral barium administration. Fluoroscopy could not resolve hemoclips placed at surgery and barium paste was less radiopaque than desired and not commonly used. Esophagrams revealed passage of both liquid barium and barium soaked food. Baseline images were acquired prior to surgery (Figure 4A) and with serial evaluation, reduced peristalsis and esophageal dilation were present, but there was active propagation of the bolus across the aboral esophagus and gravity assisted bolus propagation across the lower esophageal sphincter into the stomach. Some retrograde barium flow was also seen mostly with liquid administration. Retention of barium across the defect area likely corresponded with diverticulum on corroborative endoscopy (Figure 4B-4G).
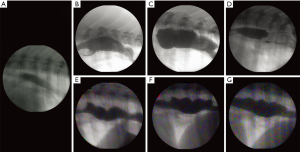
Discussion
An acellular PU graft across a 5 or 10 cm defect was investigated in this study, and functional healing evaluated and compared to healing noted in a limited control population. Complete muscle regeneration was not apparent in any group, however, epithelialization of the repaired region occurred. While the gap was not repopulated with similar architecture to native esophageal tissue, the region healed with no leak, serving as a conduit for food and fluid passage. Healing was also seen in control animals over a longer time course.
Re-epithelialization was seen by 60 days, with further tissue healing and maturation noted at one year. In an effort to capture intermediate time points, we evaluated multiple post-operative time points for 5 and 10 cm esophageal defects. Prior to re-epithelialization, there appeared to be proliferation of fibroblasts and deposition of connective tissue across the defect. Subsequently, epithelial cells proliferated and migrated across the defect. Islands of smooth muscle were seen in long-term animals, but the resultant tube was largely fibrotic without continuity as a “regenerated” layer. There was shortening of the healed area compared to the size of graft implanted, both grossly and histologically, implying some degree of tissue regeneration, contraction over the fibrotic region, or some combination of both. Potential models of tissue growth across the gap bridged by the PU graft are depicted in Figure 5.
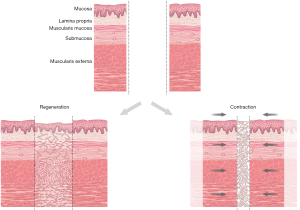
The tissue populating the gap does not maintain normal architecture as would be expected if tissue purely regenerated to mimic native esophageal structure. Similar findings of longitudinal shrinkage and progressive approximation of esophageal edges was noted in Freud’s study where a segment of cervical esophagus was replaced with lyophilized dura mater (29). This observation was also made by Gaujoux et al. who describe contraction of the graft area of at least 50% of graft length in animals surviving over one month after receiving aortic allograft across a gap in the cervical esophagus (12).
Differentiating regeneration from constriction or scar formation is paramount. Submucosal glands are variably present along the proximal third of the porcine esophagus specifically (14,30), and would not be present within regenerated margins early in the repair process. In our study, no histologic evidence of glandular tissue formation was appreciated by 12 months. Takimoto et al. note esophageal glands at 21 (5) and 42 days (31) post-operatively in a canine model of cervical esophageal repair and Poghosyan et al. note glandular tissue at 7 months in Yucatan pigs (19). Results could vary dependent on esophageal location, or between species, however, the presence of glandular tissue across a repaired gap should prompt inquiry to determine if native tissue migrated into margins of the repair from wound contraction, rather than primary tissue regeneration.
A limiting factor of all studies is defining margins of initial surgical gaps. Hemoclips were placed at transected esophageal ends for visualization, but they were not resolved via fluoroscopy, and were not physically present in resected esophageal margins at necropsy. However, there were histological landmarks indicative of original wound edges, and tissue contraction and reduction in gap width noted over time. Takimoto et al. used radiographs to visualize metal clips at measured intervals in the thoracic esophagus and show clips present with no change in distance measured (5), however, in another study from the same group, authors use a shrinkage index to calculate change in length of the 5 cm defect over time, and report up to 75% change (6). Results from radiographic interpretation could be confusing, as clips may still present in the vicinity of, but not actually on the esophagus, resulting in false positive results for regeneration over contraction.
Regardless of healing mechanism, scaffolds can be used to bridge esophageal defects and provide a functional tubular conduit to span the resected gap. Repairing the wound with scaffold and stent is a successful alternative to bridge a larger esophageal defect, even if fully regenerated esophageal tissue is lacking, as there is enough healing to permit restitution of esophageal anatomy conducive for nutritional delivery (12).
Given results seen in control animals in this study, there is questionable need for ECM use, and some studies showing no benefit of ECM placement over walled stent alone, and placement of a temporary conduit could actually enhance inflammation and fibrosis in the region (12). Jensen et al. (21) describe a regenerated patent tube by 21 days whereby cell seeded scaffolds use promoted tissue ingrowth, and formation of two muscular layers by 4–5 months in piglets. Authors describe scaffold extrusion as a favorable response when translated into a patient with esophageal atresia, though placement of a scaffold that does not integrate into healing tissue could perpetuate a foreign body response. La Francesca et al. also note scaffold extrusion and highlight scaffolds only used as a temporary template to guide esophageal regeneration, with complete longitudinal reconstruction using cell seeded scaffolds in this study (14). The scaffold used in this study is non-resorbable with no ability to undergo degradation or integration in vivo. Though biocompatible, there is no expectation of incorporation with time. The scaffold is placed with intent for removal, and to serve as a mechanical conduit during the initial phase of healing across the large gap defect. A limitation of our study is the lack of stent only long-term control animals. Though the stent and allograft are likely not mutually exclusive to healing of the esophagus, it should be considered that the allograft may contribute to fibrosis over regeneration. Le Baleur et al. suggested that the allograft can serve mechanical approximation and prevent leaking, while stenting keeps the lumen patent and fluid tight if the graft fails (15).
Though duration of stent placement is variable in animal and human studies, the optimal time seems to coincide with full healing to prevent stenosis across the repaired region. If animals are not stented, or the stent is removed too early, stricture has been seen (5-7,11,13,15,19,20,32), and human patients treated with ECM have also needed stents post-operatively for up to 3.5 years (24). The deposition of increased collagen across the wound indeed may ultimately be clinically responsible for stricture formation in clinical patients. Seemingly, for complete healing to occur, there is an ideal time that the patient must remain stented, otherwise, stricture will occur. However, in both animal and human patient populations, the duration for required stenting is variable. Though treatment is available to restent the patient, or open the strictured region via balloon dilation or bougienage, this is far from ideal and often requires multiple procedures, which can be problematic in human patients and often multiple surgical procedures would not be approved for in vivo research studies.
Conclusions
Though muscle regeneration and formation of all esophageal layers was not appreciated in this study, esophageal healing was seen with stent use. Healing of an intact tube with luminal patency and no leak could be highly translational, even if bridging architecture does not mimic native esophagus. Though stricture was seen, this complication is not unexpected and is frequently encountered in human patients. Although other groups may report more favorable outcomes, results should be scrutinized for histologic evidence of tissue regeneration in a reasonable timeframe, with consideration for the possibility of secondary wound contraction serving to appose native esophageal ends.
Acknowledgments
The authors thank Joanna King at Mayo Clinic for illustrations and Chrisoula Skouritakis at University of California, Davis for assistance with figure preparation, and Jodie Deters at Mayo Clinic for help with fluoroscopy.
Funding: This work was supported by Mayo Clinic benefactors Walter S. and Theolyn B. Dowdle.
Footnote
Reporting Checklist: The authors have completed the ARRIVE reporting checklist. Available at https://jtd.amegroups.com/article/view/10.21037/jtd-21-1559/rc
Data Sharing Statement: Available at https://jtd.amegroups.com/article/view/10.21037/jtd-21-1559/dss
Conflicts of Interest: All authors have completed the ICMJE uniform disclosure form (available at https://jtd.amegroups.com/article/view/10.21037/jtd-21-1559/coif). JJ reports that Nanofiber Solutions provided the scaffolds free of charge simply to facilitate the research and move towards a patient treatment. JJ is a co-founder and current Chief Technology Officer for Nanofiber Solutions. He is a paid employee and owns equity in the company. DAW reports that he has a relationship via Mayo Clinic with Biostage and Nanofiber Solutions. The other authors have no conflicts of interest to declare.
Ethical Statement: The authors are accountable for all aspects of the work in ensuring that questions related to the accuracy or integrity of any part of the work are appropriately investigated and resolved. Experiments were performed under protocol number A25614 and granted by Mayo Clinic Institutional Animal Care and Use Committee in compliance with AVMA guidelines for the care and use of animals.
Open Access Statement: This is an Open Access article distributed in accordance with the Creative Commons Attribution-NonCommercial-NoDerivs 4.0 International License (CC BY-NC-ND 4.0), which permits the non-commercial replication and distribution of the article with the strict proviso that no changes or edits are made and the original work is properly cited (including links to both the formal publication through the relevant DOI and the license). See: https://creativecommons.org/licenses/by-nc-nd/4.0/.
References
- In H, Palis BE, Merkow RP, et al. Doubling of 30-Day Mortality by 90 Days After Esophagectomy: A Critical Measure of Outcomes for Quality Improvement. Ann Surg 2016;263:286-91. [Crossref] [PubMed]
- Raymond DP, Seder CW, Wright CD, et al. Predictors of Major Morbidity or Mortality After Resection for Esophageal Cancer: A Society of Thoracic Surgeons General Thoracic Surgery Database Risk Adjustment Model. Ann Thorac Surg 2016;102:207-14. [Crossref] [PubMed]
- Seder CW, Wright CD, Chang AC, et al. The Society of Thoracic Surgeons General Thoracic Surgery Database Update on Outcomes and Quality. Ann Thorac Surg 2016;101:1646-54. [Crossref] [PubMed]
- Freud E, Efrati I, Kidron D, et al. Comparative experimental study of esophageal wall regeneration after prosthetic replacement. J Biomed Mater Res 1999;45:84-91. [Crossref] [PubMed]
- Takimoto Y, Nakamura T, Yamamoto Y, et al. The experimental replacement of a cervical esophageal segment with an artificial prosthesis with the use of collagen matrix and a silicone stent. J Thorac Cardiovasc Surg 1998;116:98-106. [Crossref] [PubMed]
- Yamamoto Y, Nakamura T, Shimizu Y, et al. Intrathoracic esophageal replacement in the dog with the use of an artificial esophagus composed of a collagen sponge with a double-layered silicone tube. J Thorac Cardiovasc Surg 1999;118:276-86. [Crossref] [PubMed]
- Badylak S, Meurling S, Chen M, et al. Resorbable bioscaffold for esophageal repair in a dog model. J Pediatr Surg 2000;35:1097-103. [Crossref] [PubMed]
- Isch JA, Engum SA, Ruble CA, et al. Patch esophagoplasty using AlloDerm as a tissue scaffold. J Pediatr Surg 2001;36:266-8. [Crossref] [PubMed]
- Barron MR, Blanco EW, Aho JM, et al. Full-thickness oesophageal regeneration in pig using a polyurethane mucosal cell seeded graft. J Tissue Eng Regen Med 2018;12:175-85. [Crossref] [PubMed]
- Catry J, Luong-Nguyen M, Arakelian L, et al. Circumferential Esophageal Replacement by a Tissue-engineered Substitute Using Mesenchymal Stem Cells: An Experimental Study in Mini Pigs. Cell Transplant 2017;26:1831-9. [Crossref] [PubMed]
- Doede T, Bondartschuk M, Joerck C, et al. Unsuccessful alloplastic esophageal replacement with porcine small intestinal submucosa. Artif Organs 2009;33:328-33. [Crossref] [PubMed]
- Gaujoux S, Le Balleur Y, Bruneval P, et al. Esophageal replacement by allogenic aorta in a porcine model. Surgery 2010;148:39-47. [Crossref] [PubMed]
- Jönsson L, Gatzinsky V, Jennische E, et al. Piglet model for studying esophageal regrowth after resection and interposition of a silicone stented small intestinal submucosa tube. Eur Surg Res 2011;46:169-79. [Crossref] [PubMed]
- La Francesca S, Aho JM, Barron MR, et al. Long-term regeneration and remodeling of the pig esophagus after circumferential resection using a retrievable synthetic scaffold carrying autologous cells. Sci Rep 2018;8:4123. [Crossref] [PubMed]
- Le Baleur Y, Gaujoux S, Bruneval P, et al. Self-expanding removable plastic stents for the protection of surgical anastomoses after esophageal replacement in a porcine model. Gastrointest Endosc 2010;72:790-5. [Crossref] [PubMed]
- Nakase Y, Nakamura T, Kin S, et al. Intrathoracic esophageal replacement by in situ tissue-engineered esophagus. J Thorac Cardiovasc Surg 2008;136:850-9. [Crossref] [PubMed]
- Nieponice A, Gilbert TW, Badylak SF. Reinforcement of esophageal anastomoses with an extracellular matrix scaffold in a canine model. Ann Thorac Surg 2006;82:2050-8. [Crossref] [PubMed]
- Poghosyan T, Gaujoux S, Vanneaux V, et al. In vitro development and characterization of a tissue-engineered conduit resembling esophageal wall using human and pig skeletal myoblast, oral epithelial cells, and biologic scaffolds. Tissue Eng Part A 2013;19:2242-52. [Crossref] [PubMed]
- Poghosyan T, Sfeir R, Michaud L, et al. Circumferential esophageal replacement using a tube-shaped tissue-engineered substitute: An experimental study in minipigs. Surgery 2015;158:266-77. [Crossref] [PubMed]
- Marzaro M, Algeri M, Tomao L, et al. Successful muscle regeneration by a homologous microperforated scaffold seeded with autologous mesenchymal stromal cells in a porcine esophageal substitution model. Therap Adv Gastroenterol 2020;13:1756284820923220. [Crossref] [PubMed]
- Jensen T, Wanczyk H, Sharma I, et al. Polyurethane scaffolds seeded with autologous cells can regenerate long esophageal gaps: An esophageal atresia treatment model. J Pediatr Surg 2019;54:1744-54. [Crossref] [PubMed]
- Bozuk MI, Fearing NM, Leggett PL. Use of decellularized human skin to repair esophageal anastomotic leak in humans. JSLS 2006;10:83-5. [PubMed]
- Clough A, Ball J, Smith GS, et al. Porcine small intestine submucosa matrix (Surgisis) for esophageal perforation. Ann Thorac Surg 2011;91:e15-6. [Crossref] [PubMed]
- Dua KS, Hogan WJ, Aadam AA, et al. In-vivo oesophageal regeneration in a human being by use of a non-biological scaffold and extracellular matrix. Lancet 2016;388:55-61. [Crossref] [PubMed]
- Morse CR. Repair of bronchial esophageal fistula using Alloderm. Ann Thorac Surg 2009;88:1018-9. [Crossref] [PubMed]
- Nieponice A, Ciotola FF, Nachman F, et al. Patch esophagoplasty: esophageal reconstruction using biologic scaffolds. Ann Thorac Surg 2014;97:283-8. [Crossref] [PubMed]
- Thomas M, Allen MS, Shen KR, et al. A novel use of human acellular dermis for conduit salvage after esophagectomy. Ann Thorac Surg 2014;97:1459-63. [Crossref] [PubMed]
- Badylak SF, Hoppo T, Nieponice A, et al. Esophageal preservation in five male patients after endoscopic inner-layer circumferential resection in the setting of superficial cancer: a regenerative medicine approach with a biologic scaffold. Tissue Eng Part A 2011;17:1643-50. [Crossref] [PubMed]
- Freud E, Greif M, Rozner M, et al. Bridging of esophageal defects with lyophilized dura mater: an experimental study. J Pediatr Surg 1993;28:986-9. [Crossref] [PubMed]
- Krüger L, Gonzalez LM, Pridgen TA, et al. Ductular and proliferative response of esophageal submucosal glands in a porcine model of esophageal injury and repair. Am J Physiol Gastrointest Liver Physiol 2017;313:G180-91. [Crossref] [PubMed]
- Takimoto Y, Nakamura T, Teramachi M, et al. Replacement of long segments of the esophagus with a collagen-silicone composite tube. ASAIO J 1995;41:M605-8. [Crossref] [PubMed]
- Natsume T, Ike O, Okada T, et al. Experimental studies of a hybrid artificial esophagus combined with autologous mucosal cells. ASAIO Trans 1990;36:M435-7. [PubMed]