Long chain noncoding RNA-ROR promotes hypoxic injury of cardiomyocytes by targeting the miR-145/HAX-1 axis
Introduction
Myocardial hypertrophy is a slow-generating, but effective compensatory function that occurs mainly in cases of chronic pressure overload, where the total amount of myocardium increases and contractility is enhanced, allowing the heart to maintain normal blood circulation while having considerable reserve capacity. However, this compensatory function also has its disadvantages, mainly because the hypertrophied myocardium has an increased oxygen demand which is often not met by the coronary artery supply, resulting in myocardial ischemia, which will eventually lead to a reduction in myocardial contractility. Hypertrophic cardiomyopathy is an autosomal dominant disorder, 60–70% familial and 30–40% sporadic, with familial and disseminated cases, and pediatric and adult cases having the same causative gene mutations. Mutations in at least 14 genes have been shown to be associated with the development of hypertrophic cardiomyopathy, 10 of which are genes encoding structural proteins of the myocardium, and the vast majority of mutations are in these genes. It is more common in young adults and often has a family history. It may be asymptomatic or may present with palpitations, exertional dyspnea, precordial boredom, easy fatigue, syncope, or even sudden death, with advanced manifestations of left heart failure. In patients with obstructive hypertrophic myocardium, a coarse, late systolic jet murmur may be present at the left sternal border, which may be accompanied by tremor and is enhanced by digitalis preparations, nitroglycerin, intravenous isoprenaline, and Valsalva manoeuvres, and conversely diminished by beta-blockers, norepinephrine, and squatting. In some patients, systolic murmurs of S3 and S4 heart sounds and relative mitral valve insufficiency in the apical region are heard.
Myocardial hypertrophy is associated with an adaptive response of the heart that maintains essentially normal cardiac function in the early stages of myocardial injury. However, persistent myocardial hypertrophy in its advanced stages is usually accompanied by cardiac remodeling, which may lead to heart failure or even cardiogenic death (1). Although a range of factors such as specific peptide hormones, growth factors and micro RNAs (miRNA) have been identified in association with cardiac hypertrophy, the underlying molecular mechanisms remain unclear (2). Long non-coding RNAs (lncRNAs) are a class of non-protein coding RNAs greater than 200 nucleotides (nt) in length that play a key role in regulating different biological behaviors (3). The lncRNA reprogramming regulator (ROR) is a well-studied lncRNA in malignancy, but little research has been conducted on its role in cardiovascular disease. The miRNAs are a class of non-protein coding RNAs less than 22 nt in length. Many miRNA have been reported to play a key role in the development and progression of various diseases including cardiac hypertrophy (4), for example, there is abnormally low expression of miR-22 in cardiac hypertrophy (5); miR-301a, a newly identified cardiac regulator, is abnormally highly expressed in hypertrophic myocardium (6). In contrast, ROR knockdown resulted in reduced cell volume and diminished levels of atrial natriuretic peptide (ANP) and B-type natriuretic peptide (BNP) expression after phenylephrine (PE) treatment. Although there have been many studies on hypoxic injury of cardiomyocytes, the thoracic aortic arch constriction (TAC) model is the most stable one among the many rat models of myocardial injury established, and LncRNA ROR is highly expressed in the TAC model, so our study has stable reproducibility. In the present study, we discussed the molecular correlation between lncRNAs and miRNAs with a view to further revealing the role of both in the development and progression of myocardial hypertrophy. We present the following article in accordance with the ARRIVE reporting checklist (available at https://jtd.amegroups.com/article/view/10.21037/jtd-22-501/rc).
Methods
Experimental animals
A total of 40 specific-pathogen-free (SPF)-grade 8-week-old male C57BL6 mice, weighing 20–25 g, were purchased from the First Hospital of Sun Yat-sen University [production license number: SCXK (Guangdong) 2020-0056]. All mice were housed at room temperature (22±1 ℃) and relative humidity (40–70%) in a 12-hour day/night cycle. The rats were allowed to eat and drink freely. After a week of adaptive feeding, the mice were randomly divided into a Model group (n=20) and Sham group (n=20). For the Model group, conventional TAC was used to prepare the myocardial hypertrophy model. After satisfactory anesthesia, the mice were fixed on the animal operating table, the trachea was intubated and connected to the ventilator, the free aortic arch was exposed after opening the chest, a 27 G needle was padded between the left common carotid artery and the innominate artery, and the aortic arch was ligated with a 7-0 silk thread. The needle was then removed to create a narrowing zone and the procedure was completed with closed chest sutures layer by layer (2). For the Sham-operated group, all steps were the same as in the model group, but without ligation of the aortic arch. At the end of the experiments, the animals were euthanized by decapitation and all animals were included in the data analysis. A protocol was prepared before the study without registration. Animal experiments were performed under a project license (No. 2019-182) granted by the Animal Ethics Committee of Chongqing General Hospital, in compliance with Guide for the Care and Use of Laboratory Animals, 8th edition for the care and use of animals.
Hypertrophic cardiomyocyte culture and detection of myocardial injury indicators
Cardiomyocyte isolation was performed on mice. The extracted myocardial tissue was cut into small pieces and transferred to 4-(2-hydroxyethyl)-1-piperazineethanesulfonic acid (HEPES)-buffered saline (Gibco, USA) containing 0.1% type IV collagenase (Gibco, USA), 0.1% trypsin (Gibco, USA), and 15 µg/mL DNase I at 37 ℃. After centrifugation, the lysed cells were resuspended in Dulbecco’s modified Eagle’s medium (DMEM; Gibco, USA) with nutrients and then inoculated on collagen-coated silica sheets in preparation for extraction of cardiomyocytes. After 24 hours post inoculation, the original culture medium was replaced with serum-free medium and the culture was continued for 24 hours. All cells used in the experiments were pre-treated with PE. Lactate dehydrogenase (LDH), malondialdehyde (MDA), superoxide dismutase (SOD) and creatine kinase (CK) assay kits were purchased from Nanjing Jiancheng Biological Engineering Research Institute, all tests are performed according to kit instructions.
Plasmid construction and transfection
The pcDNA3.0-ROR and pcDNA3.0-HAX-1 (HS1-associated protein X-1) vectors were constructed on the basis of specific primer amplification. Complementary DNAs (cDNA) for ROR and HAX-1 were cloned into the mammalian expression vector pcDNA3.0. The specific primers used in this procedure were purchased from Bioengineering (Shanghai, China) Co. miR-145 mimics (miR-145 mimics) and negative control (NC) mock plasmid vectors were purchased from Thermo Fisher Scientific (Shanghai, China). The pcDNA3.0-ROR and pcDNA3.0-HAX-1 vector plasmids and miR-145 mimics were transfected with Lipofectamine 2000 (Invitrogen, Carlsbad, CA, USA) in cardiomyocytes. After 48 hours of transfection, cardiomyocytes were taken and subjected to reverse transcription-polymerase chain reaction (RT-PCR) and western blot.
RT-PCR
Total RNA was extracted from cardiomyocytes using TRIzol reagent (Invitrogen, USA). The concentration and quality of RNA were determined. The RNA integrity was determined using agarose gel electrophoresis. Glyceraldehyde 3-phosphate dehydrogenase (GAPDH) was used as an endogenous control. According to the reverse transcription kit instructions, 500 ng of RNA template was added, and cDNA was obtained by RT using a 20 µL reaction system. The RT conditions were 37 ℃ for 15 minutes, 85 ℃ for 5 seconds. Quantitative (q)RT-PCR was performed using a Phusion reaction system (Thermo Fisher Scientific, Waltham, MA, USA) and SYBR PreMix Ex Taq™II (Takara, Shiga, Japan) for qRT-PCR. reaction conditions were 95 ℃ for 30 seconds, 40 cycles of 95 ℃ for 5 seconds, and 60 ℃ for 30 seconds. The primer sequences for each gene are shown in Table 1.
Table 1
Genes | Coding strand | Anticoding strand |
---|---|---|
ANP | 5'-CTCCGATAGATCTGCCCTTGAA-3' | 5'-GGTACCGGAAGC TTGCAGCCTA-3' |
BNP | 5'-GCTTGAAGGACCAAGC-3' | 5'-GATCCGATCCGGGTCTCGTGC-3' |
miRNA-145 | 5'-CCACCCATGGCAAATTCCATGGCA-3' | 5'-TCTAGACGGCAGGTCAGGTCAGCCC-3' |
ROR | 5'-GTGGTTCTCCTCCAGTTGATAG-3' | 5'-AGGAGCCCAAAGTAACAG-3' |
GAPDH | 5'-ctggaacggtgaaggtgaca-3' | 5'-gggacttcctgtaacaatgca-3' |
ANP, atrial natriuretic peptide; BNP, B-type natriuretic peptide; miRNA, micro ribonucleic acid; ROR, reprogramming regulators; GAPDH, glyceraldehyde 3-phosphate dehydrogenase.
Analysis of luciferase reporter genes
The luciferase reporter gene was cloned using a specific plasmid for luciferase reporter gene analysis. The luciferase reporter plasmid containing the lncRNA ROR sequence was constructed by Guangzhou Ribo Biotechnology Co. (Guangdong, China). We then transfected the plasmids into cells using the luciferase plasmids. We co-transfected HeLa cells with 50 or 100 nM of miRNA-145 NC or miRNA-145 mimics and 0.4 µg of luciferase reporter vector in 12-well plates. After 48 hours, luciferase activity was detected using a Monolight 3010 luminometer (Analytical Lab, St. Paul, MN, USA).
Hematoxylin-eosin (HE) staining
Rats in the model and sham-operated groups were executed, and myocardial tissues were fixed in 10% formaldehyde solution by volume, made into slices. The slices were baked at 60 ℃ for 20 min, dewaxed in xylene I and II for 10 min each, dehydrated in 100%, 95%, 85% and 75% gradient ethanol for 5 min each, washed in distilled water for 5 min, stained with hematoxylin for 5 min, and washed in water for 5 min, divided by 1% ethanol hydrochloride, washed with water to reverse the blue, and stained with eosin for 5 s, full water washing, 75%, 85%, 95%, 100% I, II gradient ethanol dehydration for 2 min each, xylene I and II transparent for 5 min each. The films were sealed with neutral gum, microscopically observed and photographed.
Western blot
Total myocardial tissue proteins were extracted and protein quantification was performed using the bicinchoninic acid (BCA) protein analysis kit (Thermo Fisher, USA). A 12% sodium dodecyl sulfate (SDS) polyacrylamide gel and a 5% concentrated gel were configured for electrophoresis. Proteins were transferred to polyvinylidene fluoride (PVDF) membranes, blocked with 5% bovine serum albumin (BSA) for 1 hour, incubated overnight with the corresponding rabbit anti-human polyclonal antibodies ANP (dilution 1:1,000; Abcam, Cambridge, UK), BNP (dilution 1:1,000; Abcam, UK), and GAPDH (dilution 1:1,000; Abcam, UK). Membranes were washed 3 times with tris-buffered saline with Tween 20 (TBST), incubated with goat anti-rabbit horseradish peroxidase (HRP)-coupled secondary polyclonal antibody (diluted 1:5,000; Abcam, UK) for 1 hour, washed 3 times with TBST for 5 minutes each time, and fixed by adding appropriate amounts of enhanced chemiluminescence (ECL) light solution in the dark room. Bands were scanned and then analyzed for grey-scale values using ImageJ software (National Institutes of Health, Bethesda, MD, USA) to calculate the relative expression of proteins.
Statistical analysis
The software SPSS 20.0 (IBM Corp., Armonk, NY, USA) and GraphPad Prism 5.0 (GraphPad Software, San Diego, CA, USA) were used for data processing. All animal were enrolled in the analysis. The statistical data were expressed as the number of cases and the measurement data were expressed as the mean ± standard deviation, and the measurement data were all normally distributed. The t-test was used for comparison between groups. Correlation analysis using Pearson analysis. A P value <0.05 was considered statistically significant.
Results
Morphological observation of hypoxic hypertrophied myocardium
Microscopic observation of myocardial tissues of rats showed that myocardial cells in the sham group were in a normal state, with only a small amount of collagen fibers and no pathological changes. In the model group, the collagen fibers proliferated into a network, the arrangement was disordered, a large number of fibrous scar formation, cardiomyocyte hypertrophy, uneven coloring, nucleus consolidation, fragmentation, and even disappearance. See Figure 1.
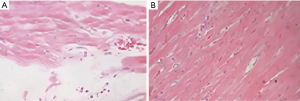
Upregulation of lncRNA ROR expression in hypertrophic cardiomyocytes
The RT-PCR showed that the relative expression of lncRNA ROR in the cardiomyocytes of the Sham and Model groups were (1.11±0.19) and (4.82±0.29), respectively, and the expression level of ROR in the Model group was significantly higher than that in Sham group. Meanwhile, the relative expression of ANP messenger ribonucleic acid (mRNA) was (1.12±0.15), (5.64±0.72) and BNP mRNA was (1.08±0.14), (6.13±0.88) in the cardiomyocytes of Sham and Model groups, respectively, and the expression levels of ANP and BNP in the Model group were significantly higher than those in the Sham group, which was consistent with the western blot assay trend. This suggests that lncRNA ROR can be involved in the pathological process of cardiac hypertrophy. See Figure 2. LDH, CK and MDA levels in the Model group were higher than those in the Sham group, and SOD levels were lower than those in the Sham group, indicating that myocardium was significantly damaged in the Model group after hypoxia. See Table 2.
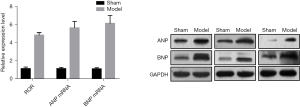
Table 2
Grouping | LDH (U/L) | CK (U/mL) | SOD (U/L) | MDA (nmol/mL) |
---|---|---|---|---|
Sham group | 71.35±9.45a | 2.58±0.28a | 131.32±12.65a | 6.47±0.78a |
Model group | 243.88±25.64 | 11.21±1.66 | 42.31±3.98 | 9.42±1.02 |
Data is presented as mean ± standard deviation. a, P<0.05 compared to model group. LDH, lactate dehydrogenase; CK, creatine kinase; SOD, superoxide dismutase; MDA, malondialdehyde.
Knockdown of lncRNA ROR inhibits PE-induced hypertrophic response in cardiomyocytes
The RT-PCR analysis showed that the expression levels of ROR were significantly enhanced in hypertrophic myocardium after PE intervention. After transfection with si-ROR, the expression level of ROR was significantly reduced in PE-treated hypertrophic cardiomyocytes, while the increase in ANP and BNP mRNA and protein levels was also significantly attenuated (Figure 3). LDH, CK and MDA levels were higher in the si-RNA group than in the si-ROR group, and SOD levels were lower than in the si-ROR group, indicating that myocardial injury was significantly reversed after knockdown of lncRNA ROR. See Table 3.
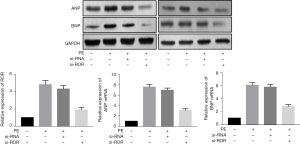
Table 3
Grouping | LDH (U/L) | CK (U/mL) | SOD (U/L) | MDA (nmol/mL) |
---|---|---|---|---|
si-RNA | 235.67±23.23a | 12.19±1.54a | 43.56±4.21a | 9.88±1.21a |
si-ROR | 69.27±8.31 | 2.46±0.22 | 144.56±14.43 | 5.67±0.83 |
Data is presented as mean ± standard deviation. a, P<0.05 compared to si-ROR group. LDH, lactate dehydrogenase; CK, creatine kinase; SOD, superoxide dismutase; MDA, malondialdehyde; si-RNA, small interfering ribonucleic acid; ROR, reprogramming regulators.
miR-145 as a target for ROR inhibition of cardiac hypertrophy
The RT-PCR assay showed that miR-145 expression was significantly down-regulated in cardiomyocytes after PE treatment. In hypertrophic cardiomyocytes, upregulation of miR-145 expression was followed by a further decrease in the relative mRNA and protein expression of ANP and BNP induced by PE. Pearson correlation analysis showed that miR-145 was negatively correlated with ROR expression in cardiac hypertrophy (Figure 4).
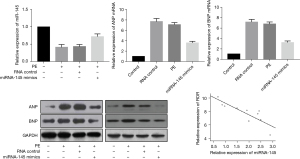
ROR-targeted regulation of miR-145 expression
Bioinformatics software analysis of the 3'untranslated region (3'UTR) of ROR was able to target binding to miR-145, and the specific nt sequence is shown in Figure 5. The dual luciferase reporter gene assay revealed that wild type (WT)-ROR activity was significantly inhibited (P<0.05) and MUT-ROR activity was not significantly changed (P>0.05) in the miR-145 group compared to the miR-NC group, as shown in Table 4. This suggests that in hypertrophic myocardium, ROR can bind directly to miR-145 for effect.
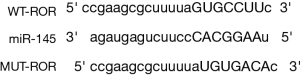
Table 4
Grouping | WT-ROR | MUT-ROR |
---|---|---|
miR-NC group | 1.05±0.10 | 1.06±0.09 |
miR-145 group | 0.33±0.04 | 0.98±0.06 |
t | 11.326 | 0.720 |
P value | 0.000 | 0.513 |
WT, wild type; ROR, reprogramming regulators; MUT, mutant; miR, micro ribonucleic acid.
lncRNA ROR upregulates HAX-1 expression by targeting binding to miR-145
When cardiomyocytes were transfected with HAX-1 3'URT WT vector and simultaneously transfected with miRNA-145 mimics, miRNA-145 inhibitors, and their respective controls, it was found that cellular luciferase properties were significantly down-regulated after transfection with mimics (P<0.05) and up-regulated after transfection with inhibitors compared to controls (P<0.05). When cardiomyocytes were transfected with HAX-1 3'UTR mutant vector and simultaneously transfected with miRNA-145 mimics, miRNA-145 inhibitors, and their respective controls, there was no significant difference in cellular luciferase activity between the groups (P>0.05). The above results suggest that miR-145 can be formulated to target the expression of HAX-1 (Table 5). The results of western blotting assay showed that knockdown of ROR downregulated HAX-1 protein expression level, while inhibition of miR-143-3p expression or upregulation of HAX-1 expression both promoted HAX-1 protein expression level, and the differences were statistically significant (All P values <0.001). Pearson correlation analysis showed that miR-145 was negatively correlated with HAX-1 mRNA expression in myocardial hypertrophy, and ROR was positively correlated with HAX-1 mRNA. The results indicate that lncRNA ROR upregulates HAX-1 expression by targeting binding to miR-145. See Figure 6.
Table 5
Report gene vectors | miRNA control group | miRNA-145 mimics | siRNA control group | miRNA-145 inhibitor |
---|---|---|---|---|
WT-HAX-1 | 1.00±0.05 | 0.67±0.08a | 1.00±0.16 | 1.42±0.07b |
MT-HAX-1 | 1.00±0.04 | 1.05±0.06 | 1.00±0.05 | 1.04±0.03 |
a, P<0.05 compared to miRNA control; b, P<0.05 compared to siRNA control. HAX-1, HS1-associated protein X-1; miRNA, micro ribonucleic acid; siRNA, small interfering ribonucleic acid; WT, wild type; MT, mutant.
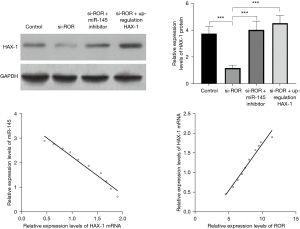
Discussion
Cardiac hypertrophy is a powerful form of compensation; however, it is not unlimited. If the cause of the disease is not eventually eliminated, the function of the hypertrophied myocardium cannot be maintained in the long term, and will eventually turn into heart failure. Chronic heart failure usually develops gradually on the basis of compensatory hypertrophy of the myocardium. Why hypertrophy leads to failure has long been explored and studied. It is now believed that compensatory cardiac hypertrophy is a form of unbalanced growth. This unbalanced growth, which manifests itself characteristically at different organ, tissue, cellular, and molecular levels, underlies the shift of the hypertrophied myocardium towards insufficiency. In the heart as a whole, unbalanced growth is manifested by an increase in the weight of the heart exceeding the growth of the axons of the sympathetic neurons innervating the heart, so that the distribution of sympathetic nerves is significantly less dense than normal. In addition, catecholamine synthesis is reduced and consumption is increased in hypertrophied myocardium, resulting in a significant reduction in intracardiac norepinephrine content. This change in innervation and transmitter content contributes to an impaired excitation-contraction coupling in the myocardium, resulting in reduced contractility. At the tissue level, this is characterized by a marked lag in the growth of micro-arteries and capillaries within the myocardium compared to the growth of cardiomyocyte volume, so that the number of capillaries per unit weight of hypertrophied myocardium is reduced. The average capillary spacing is 16.8 µm. When the heart is stressed or hypoxic, the precapillary sphincter relaxes and the approximately 2,100 capillaries that were in storage also open. The capillary spacing is thus reduced to 5.5 µm. As a result, the increased myocardial oxygen demand due to increased load is quickly met by accelerated primary transport. In myocardial hypertrophy, however, the total number of capillaries is relatively reduced and the diffusion spacing of oxygen is increased, so the myocardium is hypoxic.
In such patients, most of the storage capillaries are already open in the quiet state, so when the load is increased, there is no significant increase in the number of functional capillaries and the diffusion spacing of oxygen is not significantly reduced. As a result, hypertrophied myocardium is often hypoxic during an increased load, resulting in reduced aerobic metabolism, insufficient energy production, and reduced myocardial contractility. Whether the hypertrophied myocardium is hypoxic has been the subject of different opinions, as the difference in oxygen content per unit weight of coronary arterial and venous blood in the hypertrophied myocardium has been measured and the results are not significantly different from those of normal myocardium, indicating that the hypertrophied myocardium is not hypoxic.
Pathological hypertrophy caused by chronic hypertension, genetic predisposition, or aortic stenosis leads to maladaptive remodeling and is often accompanied by ventricular dysfunction, followed by heart failure and death (7). Many lncRNA have been reported to play key roles in cardiac hypertrophy (8). The highly expressed lncRNA ROR has been shown to play an important role in the regulation of growth in the mouse embryo and early birth (9). However, it is unclear whether H19 is cardioprotective or whether it promotes cardiac disease. The ROR expression peaked after TAC-induced pressure overload induced left ventricular heart failure and was also significantly highly expressed during the decompensated phase of hypertrophic cardiac remodeling, so we hypothesized that lncRNA ROR may have myocardial-specific damaging effects and could be used as a therapeutic target.
Cardiac hypertrophy is associated with a variety of pathophysiological factors, and its progression is closely linked to the expression of embryonic genes such as ANP and BNP, and to protein synthesis (5). LDH and CK are markers of myocardial tissue damage, and increased levels indicate myocardial injury. SOD is an important antioxidant enzyme in the body, and the higher its activity, the stronger the cellular antioxidant capacity. Myocardial injury can lead to lipid peroxidation and excessive production of MDA (10). lncRNA and miRNA have been reported to play an important role in the pathogenesis of cardiovascular disease in cardiac hypertrophy. A study on the molecular mechanisms of these lncRNA and miRNA has clarified the potential role of both in clinical applications (11). Although lncRNA ROR is a marker of poor prognosis in chronic heart failure and miR-145 is a potent suppressor of congenital heart disease, the potential mechanisms targeting the biological role of ROR and miR-145 in myocardial hypertrophy remain unclear (12). In the present study, a model of ROR expression was enhanced in the hearts of mice with postoperative myocardial hypertrophy in the TAC group compared with those in the sham-operated group. Inhibition of ROR expression decreased cardiomyocyte ANP and BNP expression, suggesting that ROR has a pro-growth role in myocardial hypertrophy. In addition, the postoperative LDH, CK, MDA levels of mice in the TAC group were found to be higher than those in the sham group, and the SOD levels were lower than those in the sham group. Inhibition of ROR expression decreased LDH, CK, MDA levels and increased SOD levels. This indicates that inhibition of ROR expression can improve myocardial injury.
Meanwhile, miRNA have also been extensively studied to explore the pathogenesis of cardiac hypertrophy due to their diverse biological functions. Many miRNA were found to be dysregulated in expression in hypertrophic cardiomyocytes. Some miRNA are highly expressed in hypertrophic cardiomyocytes (13), such as miR-21, miR-208, and miR-499, while another part of miRNA are lowly expressed in cardiac hypertrophy, such as miR-1, miR-133, and miR-30 (14). However, the role of miR-145 is not clear in myocardial hypertrophy. In the present study, we confirmed that miR-145 expression was downregulated in PE-induced hypertrophic cardiomyocytes and that overexpression of miR-184 significantly reduced the elevated ANP and BNP mRNA and protein levels. Furthermore, miR-145 was shown to be a direct target of ROR during myocardial hypertrophy through a dual luciferase gene reporter, negatively correlating with ROR expression levels during the progression of hypertrophic cardiomyocytes.
Numerous studies have reported the mechanism of action of lncRNA as competing endogenous RNAs (ceRNA) in various diseases including cardiac hypertrophy (15). For example, in advanced gastric cancer, lncRNA HOTAIR may function as ceRNA (16); in cardiac fibrosis, lncRNA H19 functions as a ceRNA (17). In this study, we found that HAX-1 mRNA expression was upregulated in PE-treated cardiomyocytes and that silencing of HAX-1 inhibited the expression of ANP and BNP. In hypertrophied cardiomyocytes, high expression of HAX-1 was negatively correlated with miR-145, and reporter analysis confirmed that HAX-1 is a target of miR-145. However, overexpression of ROR rescued the miR-145 mimic-mediated attenuation of HAX-1 luciferase activity, suggesting that ROR regulates HAX-1 expression in cardiac hypertrophy by competitively binding to miR-145. Currently, a large number of studies have elaborated the molecular mechanisms of lncRNA involvement in diseases, and lncRNA therapies have been the focus of research in the last decade, but no lncRNA-targeted therapies have yet entered clinical development. As biomarkers, researchers are actively searching for a general link between lncRNAs and diseases, and as research progresses, lncRNA-targeted therapies will be widely used in the clinic.
A limitation of this study is that it was an animal study, and the mechanism has not been confirmed in patients.
In summary, this study found that lncRNA ROR can promote the expression of HAX-1 by competitively binding to miR-145, providing a new explanation for the promoting role of lncRNA ROR in myocardial hypertrophy, with the expectation that this study will bring some new insights into the diagnosis and treatment of myocardial hypertrophy.
Acknowledgments
Funding: None.
Footnote
Reporting Checklist: The authors have completed the ARRIVE reporting checklist. Available at https://jtd.amegroups.com/article/view/10.21037/jtd-22-501/rc
Data Sharing Statement: Available at https://jtd.amegroups.com/article/view/10.21037/jtd-22-501/dss
Conflicts of Interest: All authors have completed the ICMJE uniform disclosure form (available at https://jtd.amegroups.com/article/view/10.21037/jtd-22-501/coif). The authors have no conflicts of interest to declare.
Ethical Statement: The authors are accountable for all aspects of the work in ensuring that questions related to the accuracy or integrity of any part of the work are appropriately investigated and resolved. Animal experiments were performed under a project license (No. 2019-182) granted by the Animal Ethics Committee of Chongqing General Hospital, in compliance with Guide for the Care and Use of Laboratory Animals, 8th edition for the care and use of animals.
Open Access Statement: This is an Open Access article distributed in accordance with the Creative Commons Attribution-NonCommercial-NoDerivs 4.0 International License (CC BY-NC-ND 4.0), which permits the non-commercial replication and distribution of the article with the strict proviso that no changes or edits are made and the original work is properly cited (including links to both the formal publication through the relevant DOI and the license). See: https://creativecommons.org/licenses/by-nc-nd/4.0/.
References
- Song C, Qi H, Liu Y, et al. Inhibition of lncRNA Gm15834 Attenuates Autophagy-Mediated Myocardial Hypertrophy via the miR-30b-3p/ULK1 Axis in Mice. Mol Ther 2021;29:1120-37. [Crossref] [PubMed]
- Yu B, Zhao Y, Zhang H, et al. Inhibition of microRNA-143-3p attenuates myocardial hypertrophy by inhibiting inflammatory response. Cell Biol Int 2018;42:1584-93. [Crossref] [PubMed]
- Zeng Z, Pan Y, Wu W, et al. Myocardial hypertrophy is improved with berberine treatment via long non-coding RNA MIAT-mediated autophagy. J Pharm Pharmacol 2019;71:1822-31. [Crossref] [PubMed]
- Sucharov C, Bristow MR, Port JD. miRNA expression in the failing human heart: functional correlates. J Mol Cell Cardiol 2008;45:185-92. [Crossref] [PubMed]
- Zhou X, Zhang W, Jin M, et al. lncRNA MIAT functions as a competing endogenous RNA to upregulate DAPK2 by sponging miR-22-3p in diabetic cardiomyopathy. Cell Death Dis 2017;8:e2929. [Crossref] [PubMed]
- Zhen L, Zhao Q, Lü J, et al. miR-301a-PTEN-AKT Signaling Induces Cardiomyocyte Proliferation and Promotes Cardiac Repair Post-MI. Mol Ther Nucleic Acids 2020;22:251-62. [Crossref] [PubMed]
- Gao R, Wang L, Bei Y, et al. Long Noncoding RNA Cardiac Physiological Hypertrophy-Associated Regulator Induces Cardiac Physiological Hypertrophy and Promotes Functional Recovery After Myocardial Ischemia-Reperfusion Injury. Circulation 2021;144:303-17. [Crossref] [PubMed]
- Li J, Wu Z, Zheng D, et al. Bioinformatics analysis of the regulatory lncRNA miRNA mRNA network and drug prediction in patients with hypertrophic cardiomyopathy. Mol Med Rep 2019;20:549-58. [Crossref] [PubMed]
- Li X, Zhou J, Huang K. Inhibition of the lncRNA Mirt1 Attenuates Acute Myocardial Infarction by Suppressing NF-κB Activation. Cell Physiol Biochem 2017;42:1153-64. [Crossref] [PubMed]
- Yu W, Qin X, Zhang Y, et al. Curcumin suppresses doxorubicin-induced cardiomyocyte pyroptosis via a PI3K/Akt/mTOR-dependent manner. Cardiovasc Diagn Ther 2020;10:752-69. [Crossref] [PubMed]
- Yuan Y, Wang J, Chen Q, et al. Long non-coding RNA cytoskeleton regulator RNA (CYTOR) modulates pathological cardiac hypertrophy through miR-155-mediated IKKi signaling. Biochim Biophys Acta Mol Basis Dis 2019;1865:1421-7. [Crossref] [PubMed]
- Bao Q, Chen L, Li J, et al. Role of microRNA-124 in cardiomyocyte hypertrophy inducedby angiotensin II. Cell Mol Biol (Noisy-le-grand) 2017;63:23-7. [Crossref] [PubMed]
- Mao ZJ, Zhang QL, Shang J, et al. Shenfu Injection attenuates rat myocardial hypertrophy by up-regulating miR-19a-3p expression. Sci Rep 2018;8:4660. [Crossref] [PubMed]
- Roncarati R, Viviani Anselmi C, Losi MA, et al. Circulating miR-29a, among other up-regulated microRNAs, is the only biomarker for both hypertrophy and fibrosis in patients with hypertrophic cardiomyopathy. J Am Coll Cardiol 2014;63:920-7. [Crossref] [PubMed]
- Xu Y, Fang H, Xu Q, et al. LncRNA GAS5 inhibits NLRP3 inflammasome activation-mediated pyroptosis in diabetic cardiomyopathy by targeting miR-34b-3p/AHR. Cell Cycle 2020;19:3054-65. [Crossref] [PubMed]
- Liu XH, Sun M, Nie FQ, et al. Lnc RNA HOTAIR functions as a competing endogenous RNA to regulate HER2 expression by sponging miR-331-3p in gastric cancer. Mol Cancer 2014;13:92. [Crossref] [PubMed]
- Zhang BF, Jiang H, Chen J, et al. LncRNA H19 ameliorates myocardial infarction-induced myocardial injury and maladaptive cardiac remodelling by regulating KDM3A. J Cell Mol Med 2020;24:1099-115. [Crossref] [PubMed]
(English Language Editor: J. Jones)