Clinical outcomes and immune phenotypes associated with STK11 co-occurring mutations in non-small cell lung cancer
Introduction
The 5-year survival for metastatic non-small cell lung cancer (NSCLC) continues to be low at <20% even with the adoption of immunotherapy in standard practice. This is because NSCLC is a molecularly heterogeneous disease and the benefit from immunotherapy does not extend equally across all NSCLC molecular subgroups. STK11 (or LKB1) mutations have emerged as an important predictive marker of response to therapy. STK11 is the third most frequently mutated gene in NSCLC (10% overall; 20% in lung adenocarcinoma) (1). STK11 is a tumor suppressor gene that functions in arresting the cell cycle and inhibiting cell growth through induction of p21/WAF1 expression in a p53-dependent mechanism (2). More importantly, STK11 functions as a key upstream activator of the AMP-activated protein kinase (AMPK), a central metabolic switch that governs glucose and lipid metabolism and autophagy in response to stress (3,4). Loss of STK11 reprograms cancer cell metabolism to efficiently generate energy and biomass components for uncontrolled cell proliferation in order to expand and disseminate. Mutated STK11 is associated with larger tumor size, more frequent lymph node or distant metastasis, and poorer overall survival (OS) (5,6).
STK11 mutations are associated with suppression of immune surveillance due to reduced T cell presence and lower PD-L1 expression in tumors (7,8). Multiple preclinical, as well as clinical, studies have reported that STK11 mutation confers primary resistance to PD-1/PD-L1 therapy with response rates as low as 0–7.4% (1,9,10). Co-occurring mutations with STK11 may play a role in response to therapy as well as overall prognosis. For example, STK11 mutation is associated with worse OS when co-mutated with KRAS (5). To investigate potential predictors of therapy response in STK11-mutated NSCLC, we analyzed the effect of commonly co-occurring mutations and tumor mutation burden (TMB) on treatment responses as well as clinical outcomes in these patients using data from our clinic. We also characterized the effect of these co-occurring mutations on OS as well as intratumoral immune status in patients with STK11-mutated NSCLC using The Cancer Genome Atlas (TCGA) data. We present the following article in accordance with the REMARK reporting checklist (available at https://jtd.amegroups.com/article/view/10.21037/jtd-21-1377/rc).
Methods
Patient population
Under an IRB-approved protocol at the Cancer Institute of New Jersey for a retrospective study, we analyzed data of patients with STK11-mutated NSCLC seen in our Thoracic Oncology clinic between 2013 and 2018. Eligible patients were required to have had their tumor undergo next-generation sequencing by FoundationOne CDx (Foundation Medicine, Inc., Cambridge, MA, USA), which detects alterations in 324 cancer associated genes and select gene rearrangements as well as TMB. The study was conducted in accordance with the Declaration of Helsinki (as revised in 2013) and was approved by Rutgers University Institutional Review Board (Pro2018002434). Informed consent was not taken as this was a retrospective review of patient records.
Variables
Through review of patients’ electronic health records, we obtained patient baseline characteristics (age, gender, race, smoking history, history of any asbestos exposure, performance status) as well as cancer and treatment history (date and stage of diagnosis and progression, lines of therapy, surgery type if any, radiation details, immunotherapy response if any). Information about treatment-related adverse events was also collected. Operative and pathology reports were reviewed to determine disease staging and histology. Staging conformed to the eighth edition of the American Joint Committee on Cancer/International Union Against Cancer TNM stage classification for lung cancer (11), and was summarized as local (stage I), regional (stages II and III), and distant (stage IV) disease. Smoking history was classified as never (smoked <100 cigarettes over lifetime), former (smoked ≥100 cigarettes over lifetime, but quit more than 12 months prior to diagnosis) or current smoker at the time of diagnosis. OS was defined as the time from date of diagnosis until date of death or last follow-up. Progression-free survival (PFS) was calculated from the first administration of a therapy until the first documented tumor progression or death due to any cause, whichever occurred first. Recurrence-free survival (RFS) was calculated from the time of curative surgery or last chemo-radiation administration to the time of recurrence or death. Durable clinical benefit (DCB) was defined as alive and without disease progression [complete response (CR) + partial response (PR) + stable disease (SD)] at 6 months. Therapies that patients received were categorized as localized (focal therapies to the primary lung tumor; i.e., surgical resection or radiotherapy) or systemic (i.e., chemotherapy or immunotherapy). Molecular profiling of all tumor samples was performed using the FoundationOne CDx assay in tumor specimens. Using DNA isolated from formalin fixed paraffin embedded (FFPE) tumor tissue specimens, 50–1,000 ng of extracted DNA was used for whole-genome shotgun library construction and hybridization-based capture of all coding exons from 309 cancer-related genes, one promoter region, one non-coding (ncRNA), and select intronic regions from 34 commonly rearranged genes, 21 of which also included the coding exons. Normalized TMB was calculated as the absolute mutation burden (number of nonsynonymous mutations/sample) divided by the genomic coverage for that sample (12).
TCGA analysis
Tumor immune expression signatures, immune subtypes and immune cell imputation were accessed through the global immune classification of solid tumors based on transcriptomic profiles developed by Thorsson et al. (13). We classified the LUAD tumors into four groups of patients: STK11 wild-type (wt), STK11-mutated, STK11/KRAS co-mutated and STK11/TP53 co-mutated. To characterize intratumoral immune status, we scored 160 tumor immune expression signatures and used these to cluster tumors into six distinct immune subtypes as described previously—wound healing, IFN-g dominant, inflammatory, lymphocyte depleted, immunologically quiet, and TGF-β dominant. Thorsson et al. have characterized these immune subtypes by differences in macrophage or lymphocyte signatures, Th1:Th2 cell ratio, extent of intratumoral heterogeneity, aneuploidy, extent of neoantigen load, overall cell proliferation, expression of immunomodulatory genes, and prognosis using an extensive immunogenomic analysis of more than 10,000 TCGA tumors comprising 33 diverse cancer types (13).
Statistical analysis
Data were presented as the frequency (%) for categorical variables and median (range) for continuous variables. Associations between variables were examined with Wilcoxon rank-sum test, Pearson’s chi-square test, or Fisher’s exact test as appropriate. We evaluated patient characteristics, treatment patterns, PFS with treatment(s) and OS by each of the co-occurring mutations (KRAS, KEAP1, TP53). OS, survival after diagnosis of advanced disease and PFS were estimated by the Kaplan-Meier method and compared using a log-rank test. Patients were followed until death; patients alive at the end of the study were censored at the time of last available follow-up. Univariable and multivariable survival analyses were performed using a Cox proportional hazards model. Statistical analyses were performed using STATA (College Station, TX, USA) with two-sided tests and a significance level of 0.05.
Results
Patient and clinical characteristics
After review of 323 patient charts seen at our thoracic oncology clinic between 1/1/2015 and 6/30/2019, 41 patients were identified with STK11 mutation. Of the 41 patients identified, 22 (54%) had co-occurring KRAS mutation. The patient characteristic for all patients as well as those with KRAS-mutated tumors are listed in Table 1. Median age at diagnosis was 65 years (range, 47 to 81 years) and more than 95% had prior or current history of tobacco smoking. Moreover, about 20% of the patients reported history of exposure to potential carcinogens either through their occupation or exposure to radiation or asbestos. Patients with co-occurring KRAS were not significantly different in stage at diagnosis, presence of brain metastases or frequency of progression to metastatic disease. The median TMB was 8 mut/Mb and 38% of patients had tumors with TMB ≥10 mut/Mb. The median TMB in KRAS co-mutated tumors was 6.5 mut/Mb.
Table 1
Patient characteristics | All STK11-mutated (n=41) | STK11/KRAS co-mutated (n=22) | STK11-mutated/KRAS wt (n=19) |
---|---|---|---|
Age at diagnosis (years), median (range) | 65.4 (47.4 to 87.0) | 64.3 (47.4 to 79.8) | 67.6 (55.4 to 87.0) |
Sex, n [%] | |||
Male | 24 [59] | 13 [59] | 11 [58] |
Female | 17 [41] | 9 [41] | 8 [42] |
Race, n [%] | |||
African American | 1 [2] | 0 | 1 [5] |
White | 36 [88] | 21 [95] | 15 [79] |
Hispanic | 2 [5] | 0 | 2 [11] |
Asian | 2 [5] | 1 [5] | 1 [5] |
Smoking history | |||
Current smoker | 5 [12] | 3 [14] | 2 [11] |
Former smoker | 34 [83] | 18 [82] | 16 [84] |
Never smoker | 2 [5] | 1 [4] | 1 [5] |
Pack-years in smokers, mean (SD) | 36.9 (21.1) | 35.8 (18.3) | 38.3 (24.4) |
ECOG PS at diagnosis | |||
0–1 | 32 [78] | 17 [77] | 15 [79] |
≥2 | 9 [22] | 5 [23] | 4 [21] |
Stage at diagnosis, n [%] | |||
I–II | 12 [29] | 7 [32] | 5 [26] |
III | 7 [17] | 4 [18] | 3 [16] |
IV | 22 [54] | 11 [50] | 11 [58] |
Stage IV at any time, n [%] | 35 [85] | 18 [82] | 17 [89] |
Brain metastases, n [%] | 14 [34] | 7 [32] | 7 [37] |
Histology, n [%] | |||
Adenocarcinoma | 35 [85] | 20 [90] | 15 [79] |
Squamous | 4 [10] | 1 [5] | 3 [16] |
NSCLC, other | 2 [5] | 1 [5] | 1 [5] |
PD-L1 status | |||
N with PD-L1 known | 13 | 5 | 8 |
≥50% | 3 [23] | 2 [40] | 1 [13] |
1–50% | 4 [31] | 1 [20] | 3 [37] |
≤1% | 6 [46] | 2 [20] | 4 [50] |
TMB, mutations/Mb | |||
N with TMB available | 39 | 22 | 17 |
Median TMB, range | 8 (1 to 59.2) | 6.5 (1 to 21.7) | 9 (3.6 to 59.2) |
TMB ≥10, n [%] | 15 [38] | 7 [32] | 8 [47] |
SD, standard deviation; ECOG PS, Eastern Cooperative Oncology group scale of performance status; NSCLC, non-small cell lung cancer; TMB, tumor mutation burden.
Molecular profile
The frequent molecular alterations detected in the tumors are reported in Figure 1. The most common co-occurring alterations with STK11 were KRAS (54%), TP53 (44%), CDKN2A (37%) and KEAP1 (27%). Seven patients had co-mutations in STK11, KRAS and KEAP1. For STK11 mutations, the most common alteration was frameshift (30%), followed by truncating (23%) and missense (23%) mutations (Figure 2). The most common KRAS mutation for tumors with co-occurring KRAS mutation was G12C (n=8) and G12A (n=4).
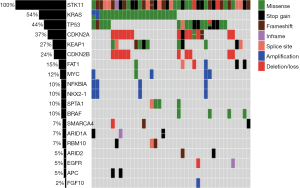
Treatment outcomes for stage I to III tumors
Table 2 describes outcomes for patients who were initially diagnosed with stage I to III NSCLC and received definitive therapy with either surgery or with concurrent chemoradiation. Overall 17 patients received localized therapy (surgery or radiation) with median OS of 8.6 years (SD ±2.1). The OS was similar in all molecular subtypes except for patients with STK11 mutation alone (no KRAS or KEAP1 alteration). Seventeen patients received surgery with 2-year RFS of 70%. Eight patients received definitive chemoradiation with 1-year RFS of 38%. The outcomes were similar across different subgroups by co-occurring mutations although comparative statistics could not be done due to small number of patients in the subgroups.
Table 2
Treatment | All patients (n=17) | KRAS-mutated | KRAS-wt | ||||
---|---|---|---|---|---|---|---|
All (n=10) | KEAP1-mut (n=2) | KEAP1-wt (n=8) | KEAP1-mut (n=3) | KEAP1-wt (n=4) | |||
Surgery, n | 10 | 5 | 2 | 3 | 2 | 3 | |
(Neo)adjuvant chemo, n [%] | 2 [20] | 1 [20] | 1 [50] | 0 | 1 [33] | 0 | |
RFS post-surgery, years | |||||||
Median (SD) | 34.8 (14.1) | 98.4 (18.4) | ** | 34.8 (26.4) | ** | 20.3 (9.0) | |
12-month PFS, n [%] | 9 [90] | 5 [100] | 2 [100] | 3 [100] | 2 [100] | 2 [67] | |
24-month PFS, n [%] | 7 [70] | 5 [100] | 2 [100] | 3 [100] | 2 [100] | 0 | |
Concurrent with radiation, n | 8 | 5 | 0 | 5 | 1 | 2 | |
RFS post-chemoradiation, months | |||||||
Median (SD) | 7.1 (0.9) | 7.5 (1.4) | ** | 7.5 (1.4) | ** | ** | |
6-month PFS, n [%] | 6 [75] | 4 [80] | ** | 4 [80] | 1 [100] | 1 [50] | |
12-month PFS, n [%] | 3 [37] | 2 [40] | ** | 2 [40] | 1 [100] | 0 | |
OS, all patients, years | |||||||
Median (SD) | 8.6 (2.1) | 8.6 (2.4) | ** | 8.6 (2.5) | ** | 1.5 (0.5) | |
% with recurrence, n [%] | 12 [71] | 6 [60] | 0 | 6 [75] | 2 [67] | 4 [100] |
**, unable to calculate due to small number. SD, standard deviation; RFS, recurrence-free survival; PFS, progression-free survival; OS, overall survival.
Treatment outcomes with metastatic disease
Thirty-five patients developed metastatic disease either at initial diagnosis or after treatment with localized therapy with surgery or radiation (Table 3). About 20% of the patients received no therapy at all due to poor performance status or rapidly progressing disease. Nineteen patients (54%) received chemotherapy as first line of therapy and 6 (17%) received immunotherapy. The overall response to chemotherapy for metastatic disease was poor, with median PFS of 2.1 months and only 4 out of 21 patients continuing on therapy for more than 6 months. Only one patient derived clinical benefit for more than one year and this patient had a co-occurring KEAP1 mutation in addition to a KRAS mutation.
Table 3
Treatment | All (n=35) | KRAS-mutated | KRAS-wt | ||||
---|---|---|---|---|---|---|---|
All (n=18) | KEAP1-mut (n=4) | KEAP1-wt (n=14) | KEAP1-mut (n=4) | KEAP1-wt (n=13) | |||
Lines of systemic therapy in metastatic setting, n [%] | |||||||
0 | 7 [20] | 4 [22] | 2 [50] | 2 [14] | 0 | 3 [23] | |
1–2 | 23 [66] | 13 [72] | 1 [25] | 12 [86] | 2 [50] | 8 [62] | |
≥3 | 5 [14] | 1 [6] | 1 [25] | 0 | 2 [50] | 2 [15] | |
First-line therapy in metastatic setting, n [%] | |||||||
Platinum based chemotherapy | 19 [68] | 9 [64] | 2 [50] | 7 [58] | 2 [50] | 8 [80] | |
Immunotherapy | 6 [21] | 3 [21] | 0 | 3 [25] | 2 [50] | 1 [20] | |
Chemotherapy + immunotherapy | 1 [4] | 1 [7] | 0 | 1 [8] | 0 | 0 | |
Targeted therapy | 2 [4] | 1 [7] | 0 | 1 [8] | 0 | 1 [20] | |
Chemotherapy | |||||||
No. received | 21 | 9 | 2 | 7 | 3 | 9 | |
PFS in months, median (SD) | 2.1 (0.7) | 1.4 (0.05) | 2 | 1.3 (0.1) | 3.5 (3.6) | 2.4 (2.4) | |
6-month PFS, n [%] | 4 [19] | 1 [11] | 0 | 1 [14] | 1 [33] | 2 [22] | |
12-month PFS, n [%] | 1 [5] | 0 | 0 | 0 | 1 [33] | 0 | |
Immunotherapy | |||||||
No. received | 15 | 7 | 1 | 6 | 4 | 4 | |
PFS in months, median (SD) | 3.3 (1.6) | 1.4 (0.3) | – | 1.1 (0.4) | 6.8 (5.7) | 1.4 (6.5) | |
6-month PFS, n [%] | 6 [40] | 1 [14] | 0 | 1 [17] | 3 [75] | 2 [50] | |
12-month PFS, n [%] | 3 [20] | 0 | 0 | 0 | 2 [50] | 1 [25] | |
Overall survival from metastatic diagnosis, months | |||||||
Median (SD) | 8.5 (1.3) | 6.7 (3.0) | 2.5 (0.9) | 8.5 (2.8) | 18.8 | 7.8 (1.5) | |
6-month OS, n [%] | 23 [66] | 9 [50] | 1 [25] | 8 | 4 [100] | 10 | |
12-month OS, n [%] | 12 [34] | 4 [22] | 1 [25] | 3 | 3 [75] | 5 |
SD, standard deviation; PFS, progression-free survival; OS, overall survival.
Fifteen patients received immunotherapy, and their median PFS was 3.3 months. Six (40%) patients had DCB of more than 6 months but all had stable disease with no PRs documented. Five of the 6 patients with durable response were KRAS-mutated with 3 having KEAP-mutated and 2 with KEAP-wt tumors. The median OS was worse in tumors with mutation in all three (STK11-mutated /KRAS-mutated/ KEAP1-mutated) at 2.5 months and longest OS was observed in tumors with co-occurring KEAP1 and STK11 mutations. Six of the 15 patients who received immunotherapy had immunotherapy-related adverse events with two grade 3 (infusion reaction and elevated lipase). We also investigated the role of co-occurring TP53 mutation in response to immunotherapy. Of the 6 patients with DCB to immunotherapy, 5 had a co-occurring TP53 mutation including the one patient with co-occurring STK11 and KRAS mutations.
PD-L1 and TMB
We had PD-L1 expression status for only 6 patients who received immunotherapy. Three of these patients had DCB (PD-L1 of 0%, 5% and 90% respectively). Figure 3 shows the Kaplan-Meier curves for median PFS with immunotherapy stratified by TMB (low, medium and high). TMB showed a favorable effect on response to immunotherapy, with median PFS being 1.4 months for TMB <10 mut/Mb and 14.8 months for high TMB ≥10 mut/Mb. Sixty percent of the patients with high TMB had DCB (3 out of 5) while only 1 out of 9 patients with low TMB had DCB.
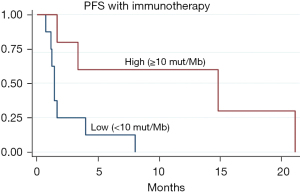
TCGA survival analysis
Univariate and multivariate results from TCGA survival analysis for lung adenocarcinoma (all stages) are summarized in Table 4. In the analysis, 421 STK11 wild-type and 67 STK11-mutated tumors were included. Tumors (all stages) with STK11 mutations as well as both STK11/KRAS co-mutations were associated with worse survival compared to tumors without STK11 or KRAS mutations [HR 3.36 (95% CI: 1.23 to 9.21) and 3.37 (95% CI: 1.33 to 8.49) respectively, P<0.0.5] after adjusting for age, gender, race, stage and smoking status. However, tumors with STK11/TP53 co-mutations did not have a significantly worse survival (HR 1.79, P=0.303) compared to tumors without STK11 or TP53 mutations.
Table 4
Co-mutation | Univariable | Multivariable* | |||||
---|---|---|---|---|---|---|---|
HR | 95% CI | P | HR | 95% CI | P | ||
STK11 wild type | Reference | Reference | Reference | Reference | |||
STK11 mutated | 2.50 | 1.00–6.21 | 0.049 | 3.36 | 1.23–9.21 | 0.018 | |
STK11/KRAS co-mutated | 2.43 | 1.09–5.42 | 0.030 | 3.37 | 1.33–8.49 | 0.010 | |
STK11/TP53 co-mutated | 1.39 | 0.53–3.63 | 0.499 | 1.79 | 0.59–5.41 | 0.303 |
*, adjusted for age, gender, race, stage and smoking status. TCGA, The Cancer Genome Atlas; HR, hazard ratio; CI, confidence interval.
TCGA immune gene expression signature analysis
Lung adenocarcinoma tumors without STK11/KRAS/TP53 mutations were enriched in the inflammatory (C3) immune subtype (Table 5), which is reported in prior studies to be associated with elevated Th17 and Th1 genes, low to moderate tumor cell proliferation, lower levels of aneuploidy and the most favorable prognosis (13). Tumors that were STK11-mutated but without a mutation in TP53 or KRAS were enriched in the inflammatory subtype (C3) but also showed increasing enrichment in the wound healing (C1) phenotype. The C1 subtype is reported to have an elevated level of angiogenic genes, high proliferation rate and low Th1/Th2 ratio related to adaptive immune response as well as exhibit increased resistance to immunotherapy agents (13,14). Lastly, the dominant phenotype in tumors with STK11 and TP53 co-mutations was the IFN-g subtype (C2). C2 is reported to have the highest M1/M2 macrophage polarization, a strong CD8 signal, the greatest T-cell receptor (TCR) diversity and highest intratumor heterogeneity (ITH) making the tumors more susceptible to immunotherapy agents (13,14).
Table 5
Immune subtype | STK11 wild type, n [%] | STK11 mutated, n [%] | STK11/KRAS co-mutated, n [%] | STK11/TP53 co-mutated, n [%] |
---|---|---|---|---|
Wound healing (C1) | 10 [8] | 4 [27] | 7 [23] | 3 [20] |
IFNg dominant (C2) | 28 [34] | 3 [20] | 4 [13] | 7 [37] |
Inflammatory (C3) | 63 [53] | 6 [40] | 18 [60] | 3 [20] |
Lymphocyte depleted (C4) | 8 [7] | 2 [13] | 0 [0] | 1 [7] |
Immunologically quiet (C5) | – | – | – | – |
TGF-β dominant (C6) | 10 [8] | 0 [0] | 1 [3] | 1 [7] |
TCGA, The Cancer Genome Atlas; IFNg, interferon-gamma; TGF-β, transforming growth factor-beta.
Discussion
In this study, we report the response to therapy and clinical outcomes in patients with STK11-mutated NSCLC by co-occurring mutations as well as TMB stratification. Our study found that, in the context of STK11 mutations, both low TMB and the presence of KRAS co-occurring mutations may be associated with poor response to immunotherapy, while TP53 co-mutations may be associated with improved responses to systemic therapies. STK11-mutated tumors also demonstrate primary chemotherapy resistance which is more pronounced in tumors with KRAS co-mutation. However, STK11 tumors diagnosed at earlier stage and treated with surgery or definitive chemoradiation had clinical outcomes similar to historical controls (15). Our findings from our patient data reported worse survival with STK11/KRAS co-mutated tumors. But presence of STK11/TP53 co-mutation did not demonstrate worst survival compared to STK11 wild type tumors.
STK11 is currently the strongest known molecularly driven cause of immunotherapy resistance in NSCLC. STK11 mutations are associated with suppression of immune surveillance due to reduced T cell presence and lower PD-L1 expression in tumors (7,8). Multiple preclinical as well as clinical studies have reported that STK11 depletion confers primary resistance to PD-1/PD-L1 therapy with response rate as low as 0 to 7.4% (9,16-18). Inactivation of STK11 by mutational or non-mutational mechanisms is associated with an inert or “cold” tumor immune microenvironment, with reduced density of infiltrating cytotoxic CD8+ T lymphocytes in both human tumors and GEMMs of NSCLC (1,19). STK11 inactivation is a driver of immune escape and innate resistance to PD-1 blockade in NSCLC tumors with co-mutation in KRAS (the most common driver mutation) (9). In our study, we also observed poor overall response to immunotherapy with median PFS of only 3.3 months. Of note, the two most significant negative predictors of response to immunotherapy were co-occurring KRAS mutation and low TMB. Conversely, TP53 co-mutation had a favorable effect on response to immunotherapy while KEAP1 co-mutation was not observed to have any effect.
Our study also observed poor response to chemotherapy with median PFS of only 2.1 months in STK11-mutated tumors with worst PFS observed in patients with co-occurring KRAS mutations. In a prior study, 102 patients with STK11 mutation treated with combination chemotherapy and immunotherapy in the first-line setting, median PFS was reported to be only 4.8 months compared to 7.2 months in 275 patients without STK11 mutation (P=0.0063) (20). This study demonstrates that STK11 lung tumors have chemotherapy resistance which is not improved with addition of immunotherapy to the therapeutic regimen. A post hoc analysis from KEYNOTE-042 also showed lower chemotherapy efficacy in tumors with STK11 vs. without STK11 mutations (21). However, a secondary analysis from KEYNOTE-189 reported shorter PFS and OS with pembrolizumab plus chemotherapy in patients with vs. without STK11 and KEAP1 mutation, but pembrolizumab plus chemotherapy was associated with numerically better outcomes than placebo plus chemotherapy regardless of mutation status (22). Our study did report that patients with STK11 mutation and unresectable stage III NSCLC treated with definitive chemoradiation had similar outcomes when compared to historical controls. The 12-month PFS was 37% in our study and prior studies have reported it to range from 35% to 42% in patients who received concurrent chemoradiation (23-26). Similarly, median PFS was 7.1 months with chemoradiation and prior studies report it to range from 5.6 to 11.8 months (23,24,26). Turchan et al. have reported worse 1-year RFS in STK11 mutated tumors after chemoradiation but this study was small in size (27). Larger studies are needed to investigate if STK11 tumors may be sensitive to radiation.
With the immune gene expression analysis and clustering into immune subtypes using the methodology developed by Thorsson et al. (13), our TCGA analysis reported that STK11 mutations with or without KRAS co-mutation lead to increased enrichment of wound healing phenotype (C1). Conversely, STK11/TP53 co-mutated tumors were associated with increased enrichment in IFN-g subtype. Mutations in STK11 were associated with an increase in the C1 wound healing signature, while the co-occurrence of a KRAS mutation led to a significant reduction of the C2 IFN-g signature, consistent with recent report (28). This may perhaps explain why STK11/KRAS co-occurring mutations are negative predictors of response to immunotherapy. Meanwhile, the presence of a TP53 co-mutation was associated with increased enrichment for IFN-g immune phenotype which is distinct from the inflammatory/wound healing phenotype seen with STK11-mutated tumors.
In an analysis of TCGA data for colorectal tumors, Soldevilla et al. (14) reported that IFN-g dominant tumors had strong immune activation (high proportion of CD8, follicular helper T cells and M1 macrophages, as well as Tregs, M2 macrophages, dendritic cells and neutrophils) and upregulation of several immune regulatory genes (PD-1/PDL1, CTLA-4, IDO1 and LAG3). Therefore, Soldevilla et al. concluded that tumors with increased enrichment of IFN-g immune subtype may make tumors more susceptible to immune checkpoint inhibition, whereas tumors of the wound healing subtype may likely be more resistant to these agents. These findings are consistent with our observations as well as prior research supporting worse prognosis with KRAS and STK11 co-mutations but improved overall prognosis with STK11/TP53 mutations (29). Moreover, pathway analysis has shown that the wound healing subtype is enriched in metabolic pathways, with greater activation of WNT and hedgehog signaling while the IFN-g dominant subtype has greater activation of pathways related to the immune system, apoptosis and DNA repair (13). Thus, co-mutations such as KRAS or TP53 may alter the immune landscape of STK11-mutated tumors. Further work is needed to determine the functional aspects of these associations. Moreover, a recent large genomic analysis reported that the frequency of STK11 mutations in significantly higher in African Americans compared to European Americans for lung adenocarcinoma (25% vs. 14%) as well as squamous cell lung cancer (8% vs. 1%) (28). This has important implications for future trials investigating therapies for STK11 mutated tumors as increased enrollment of African Americans in these trials is warranted.
STK11 mutation is associated with a more aggressive phenotype and occurs commonly in younger patients (6). These patients have poor prognosis with poor survival compared to other molecular subtypes (30). One limitation of our study was the small sample size due to which tests for statistical significance could not be performed; larger analyses are needed to confirm our findings. We did not have TMB and PD-L1 information available for all patients, although we did have TMB for >90% of the participants. We also did not have data on allele frequency of STK11 mutations in tumor samples. In TCGA analysis as well, due to limited number, we were unable to include tumors with KEAP1 mutations in the analysis. We were also not able to perform TCGA analysis stratified by stage due to the small number of tumors.
In conclusion, our retrospective analysis in patients with STK11 mutated NSCLC found that both TMB and co-occurring mutations may be predictors for response to immunotherapy with worse outcomes in patients with low TMB or KRAS co-mutation. Patients with STK11 mutated NSCLC also demonstrate chemotherapy resistance in the metastatic setting but not in the locally advanced setting when chemotherapy is combined with radiation. Moreover, co-mutations with KRAS and TP53 significantly alter tumor immune status and therefore expected response to immunotherapy. Further research is warranted to investigate radiation and novel combinations for treating metastatic STK11/KRAS co-mutated tumors. Next-generation sequencing of tumor and TMB should be obtained in all newly diagnosed STK11-mutated tumors to guide therapy.
Acknowledgments
Funding: None.
Footnote
Reporting Checklist: The authors have completed the REMARK reporting checklist. Available at https://jtd.amegroups.com/article/view/10.21037/jtd-21-1377/rc
Data Sharing Statement: Available at https://jtd.amegroups.com/article/view/10.21037/jtd-21-1377/dss
Peer Review File: Available at https://jtd.amegroups.com/article/view/10.21037/jtd-21-1377/prf
Conflicts of Interest: All authors have completed the ICMJE uniform disclosure form (available at https://jtd.amegroups.com/article/view/10.21037/jtd-21-1377/coif). JM reports grants from Bristol-Myers Squibb, personal fees from Beigene, Astra Zeneca, Blueprint medicines, and Sanofi, grants from Celldex, Beyond Spring Pharma, and Biohaven, personal fees from Oncocyte, outside the submitted work. MP reports personal fees from Bayer, outside the submitted work. NC reports grants from Merck and Tempus, outside the submitted work. SKJ reports grants and personal fees from Merck, grants from Beigene, personal fees from Syntactx and IMX Medical Reviewer, outside the submitted work. The other authors have no conflicts of interest to declare.
Ethical Statement: The authors are accountable for all aspects of the work in ensuring that questions related to the accuracy or integrity of any part of the work are appropriately investigated and resolved. The study was conducted in accordance with the Declaration of Helsinki (as revised in 2013) and was approved by Rutgers University Institutional Review Board (Pro2018002434). Informed consent was not taken as this was a retrospective review of patient records.
Open Access Statement: This is an Open Access article distributed in accordance with the Creative Commons Attribution-NonCommercial-NoDerivs 4.0 International License (CC BY-NC-ND 4.0), which permits the non-commercial replication and distribution of the article with the strict proviso that no changes or edits are made and the original work is properly cited (including links to both the formal publication through the relevant DOI and the license). See: https://creativecommons.org/licenses/by-nc-nd/4.0/.
References
- Skoulidis F, Byers LA, Diao L, et al. Co-occurring genomic alterations define major subsets of KRAS-mutant lung adenocarcinoma with distinct biology, immune profiles, and therapeutic vulnerabilities. Cancer Discov 2015;5:860-77. [Crossref] [PubMed]
- Zhong DS, Sun LL, Dong LX. Molecular mechanisms of LKB1 induced cell cycle arrest. Thorac Cancer 2013;4:229-33. [Crossref] [PubMed]
- Shackelford DB. Unravelling the connection between metabolism and tumorigenesis through studies of the liver kinase B1 tumour suppressor. J Carcinog 2013;12:16. [Crossref] [PubMed]
- Bhatt V, Khayati K, Hu ZS, et al. Autophagy modulates lipid metabolism to maintain metabolic flexibility for Lkb1-deficient Kras-driven lung tumorigenesis. Genes Dev 2019;33:150-65. [Crossref] [PubMed]
- Xiao J, Zou Y, Chen X, et al. The Prognostic Value of Decreased LKB1 in Solid Tumors: A Meta-Analysis. PLoS One 2016;11:e0152674. [Crossref] [PubMed]
- Ji H, Ramsey MR, Hayes DN, et al. LKB1 modulates lung cancer differentiation and metastasis. Nature 2007;448:807-10. [Crossref] [PubMed]
- Biton J, Mansuet-Lupo A, Pécuchet N, et al. TP53, STK11, and EGFR Mutations Predict Tumor Immune Profile and the Response to Anti-PD-1 in Lung Adenocarcinoma. Clin Cancer Res 2018;24:5710-23. [Crossref] [PubMed]
- Schabath MB, Welsh EA, Fulp WJ, et al. Differential association of STK11 and TP53 with KRAS mutation-associated gene expression, proliferation and immune surveillance in lung adenocarcinoma. Oncogene 2016;35:3209-16. [Crossref] [PubMed]
- Skoulidis F, Goldberg ME, Greenawalt DM, et al. STK11/LKB1 Mutations and PD-1 Inhibitor Resistance in KRAS-Mutant Lung Adenocarcinoma. Cancer Discov 2018;8:822-35. [Crossref] [PubMed]
- Negrao MV, Lam VK, Reuben A, et al. PD-L1 Expression, Tumor Mutational Burden, and Cancer Gene Mutations Are Stronger Predictors of Benefit from Immune Checkpoint Blockade than HLA Class I Genotype in Non-Small Cell Lung Cancer. J Thorac Oncol 2019;14:1021-31. [Crossref] [PubMed]
- Goldstraw P, Chansky K, Crowley J, et al. The IASLC Lung Cancer Staging Project: Proposals for Revision of the TNM Stage Groupings in the Forthcoming (Eighth) Edition of the TNM Classification for Lung Cancer. J Thorac Oncol 2016;11:39-51. [Crossref] [PubMed]
- Chalmers ZR, Connelly CF, Fabrizio D, et al. Analysis of 100,000 human cancer genomes reveals the landscape of tumor mutational burden. Genome Med 2017;9:34. [Crossref] [PubMed]
- Thorsson V, Gibbs DL, Brown SD, et al. The Immune Landscape of Cancer. Immunity 2018;48:812-830.e14. [Crossref] [PubMed]
- Soldevilla B, Carretero-Puche C, Gomez-Lopez G, et al. The correlation between immune subtypes and consensus molecular subtypes in colorectal cancer identifies novel tumour microenvironment profiles, with prognostic and therapeutic implications. Eur J Cancer 2019;123:118-29. [Crossref] [PubMed]
- Siegel RL, Miller KD, Fuchs HE, et al. Cancer Statistics, 2021. CA Cancer J Clin 2021;71:7-33. [Crossref] [PubMed]
- Krishnamurthy N, Goodman AM, Barkauskas DA, et al. STK11 alterations in the pan-cancer setting: prognostic and therapeutic implications. Eur J Cancer 2021;148:215-29. [Crossref] [PubMed]
- Papillon-Cavanagh S, Doshi P, Dobrin R, et al. STK11 and KEAP1 mutations as prognostic biomarkers in an observational real-world lung adenocarcinoma cohort. ESMO Open 2020;5:e000706. [Crossref] [PubMed]
- La Fleur L, Falk-Sörqvist E, Smeds P, et al. Mutation patterns in a population-based non-small cell lung cancer cohort and prognostic impact of concomitant mutations in KRAS and TP53 or STK11. Lung Cancer 2019;130:50-8. [Crossref] [PubMed]
- Koyama S, Akbay EA, Li YY, et al. STK11/LKB1 Deficiency Promotes Neutrophil Recruitment and Proinflammatory Cytokine Production to Suppress T-cell Activity in the Lung Tumor Microenvironment. Cancer Res 2016;76:999-1008. [Crossref] [PubMed]
- Skoulidis F, Arbour KC, Hellmann MD, et al. Association of STK11/LKB1 genomic alterations with lack of benefit from the addition of pembrolizumab to platinum doublet chemotherapy in non-squamous non-small cell lung cancer. J Clin Oncol 2019;37:abstr 102.
- Cho BC, Lopes G, Kowalski DM, et al. Relationship between STK11 and KEAP1 mutational status and efficacy in KEYNOTE-042: pembrolizumab monotherapy versus platinum-based chemotherapy as first-line therapy for PD-L1-positive advanced NSCLC [abstract]. In: Proceedings of the Annual Meeting of the American Association for Cancer Research 2020.80(16 suppl):Abstract nr CT084.
- Gadgeel SM, Rodriguez-Abreu D, Felip E, et al. Abstract LB-397: Pembrolizumab plus pemetrexed and platinum vs placebo plus pemetrexed and platinum as first-line therapy for metastatic nonsquamous NSCLC: analysis of KEYNOTE-189 by STK11 and KEAP1 status. Cancer Res 2020;80:LB-397. [Crossref]
- Antonia SJ, Villegas A, Daniel D, et al. Durvalumab after Chemoradiotherapy in Stage III Non-Small-Cell Lung Cancer. N Engl J Med 2017;377:1919-29. [Crossref] [PubMed]
- Senan S, Brade A, Wang LH, et al. PROCLAIM: Randomized Phase III Trial of Pemetrexed-Cisplatin or Etoposide-Cisplatin Plus Thoracic Radiation Therapy Followed by Consolidation Chemotherapy in Locally Advanced Nonsquamous Non-Small-Cell Lung Cancer. J Clin Oncol 2016;34:953-62. [Crossref] [PubMed]
- Bradley JD, Paulus R, Komaki R, et al. Standard-dose versus high-dose conformal radiotherapy with concurrent and consolidation carboplatin plus paclitaxel with or without cetuximab for patients with stage IIIA or IIIB non-small-cell lung cancer (RTOG 0617): a randomised, two-by-two factorial phase 3 study. Lancet Oncol 2015;16:187-99. [Crossref] [PubMed]
- Choy H, Schwartzberg LS, Dakhil SR, et al. Phase 2 study of pemetrexed plus carboplatin, or pemetrexed plus cisplatin with concurrent radiation therapy followed by pemetrexed consolidation in patients with favorable-prognosis inoperable stage IIIA/B non-small-cell lung cancer. J Thorac Oncol 2013;8:1308-16. [Crossref] [PubMed]
- Turchan WT, Gutiontoc S, Pitroda SP. STK11 Mutation Predicts Disease Recurrence of Medically-Inoperable Early-Stage NSCLC Patients Treated with Definitive Radiation Therapy. International Journal of Radiation Oncology 2020;108:E89. [Crossref]
- Arauz RF, Byun JS, Tandon M, et al. Whole-Exome Profiling of NSCLC Among African Americans. J Thorac Oncol 2020;15:1880-92. [Crossref] [PubMed]
- Bange E, Marmarelis ME, Hwang WT, et al. Impact of KRAS and TP53 Co-Mutations on Outcomes After First-Line Systemic Therapy Among Patients With STK11-Mutated Advanced Non-Small-Cell Lung Cancer. JCO Precis Oncol 2019;3:PO.18.00326.
- El Osta B, Behera M, Kim S, et al. Characteristics and Outcomes of Patients With Metastatic KRAS-Mutant Lung Adenocarcinomas: The Lung Cancer Mutation Consortium Experience. J Thorac Oncol 2019;14:876-89. [Crossref] [PubMed]