Moricizine prevents atrial fibrillation by late sodium current inhibition in atrial myocytes
Introduction
Atrial fibrillation (AF) is the most common cardiac arrhythmia, and results in an increased risk of morbidity and mortality, which reduces the quality of life of patients and leads to serious public health expenses (1). Antiarrhythmic drugs play an important role in the treatment of AF. However, pharmacological options remain limited due to proarrhythmic and extra-cardiac adverse effects, which have been reported previously (2). Therefore, safer and more effective drugs are urgently needed for the management of AF.
In phase 0 of the action potential, sodium channels open with a large instant inward sodium current, which is known as peak sodium current (INaP). In phases 2 and 3, there remains a persistent inward sodium current of 0.1–1.0% of INaP under normal physiological conditions, which is known as late sodium current (INaL) (3). INaL has been found to be increased in numerous pathophysiological circumstances, leading to prolonged action potential duration (APD) and calcium overload. Under this condition, early after depolarization (EAD) and delayed after depolarization (DAD) may likely be triggered, thereby resulting in the development of arrhythmias. Several studies have shown that inhibition of either endogenous or enhanced INaL is a feasible treatment for AF (4,5). Nevertheless, recent evidence has demonstrated that increased INaL activates calcium-calmodulin-dependent protein kinase II (CaMKII), subsequently triggering diastolic sarcoplasmic reticular calcium leak of Ca2+ current, which also induces EAD and DAD with the enlargement of arrhythmogenic effects (6,7). Moreover, phosphorylated CaMKII in turn leads to enhanced INaL, thereby forming a positive feedback loop in AF substrate generation.
According to Williams Vaughan’s classification (8), moricizine belongs to the class I group owing to its INaP blockage effect, which significantly reduces the depolarization velocity. The effect of moricizine on action potential shortening is currently interpreted through the suppression of L-type Ca2+ current (ICa-L) in the repolarization process (9,10), which indicates that moricizine has similar characteristics to Ib-group antiarrhythmic drugs. Moricizine was previously widely prescribed as an effective treatment for ventricular tachycardia. Previous studies confirmed that moricizine had the effect of INaP and ICa-L suppression and do not alter any outward K+ currents on atrial myocytes (10,11).
Meanwhile, a recent prospective cohort study in our center demonstrated that it could also serve as an effective and safe agent in the prevention of paroxysmal AF (12). According to our previous study (12), 65% patients with paroxysmal AF who taken moricizine maintained sinus rhythm in 1-year follow-up, and there was also a significant decrease in AF burden. Meanwhile, the average heart rate did not changed significantly after taken moricizine. No patient occurred death or malignant ventricular arrhythmias during follow-up.
The angiotensin II (Ang II)-mediated AF model shares a similar mechanism to hypertension-mediated AF, and is a widely recognized AF model. Despite the currently known electrical and structural remodeled substrate of AF (13), the alternation of INaL in the Ang II-mediated AF model has not yet been elucidated. Moricizine blocks sodium channels mainly in an inactivated state with a high affinity and slow dissociation kinetics (11). Nevertheless, the alteration of INaL by moricizine requires further investigation.
Although there have been many studies on atrial fibrillation, optimal anti-arrhythmia agents were still crucially needed for patients with slow heart rates under sinus rhythm. Moricizine is a promise choice without the side effect of bradycardia. However, the mechanism of this therapeutic effect remains unclear. Therefore, the innovation of our study is to explore the therapeutic effect of moricizine by INaL inhibition, and determine a more reliable indication on AF treatment.
Accordingly, we hypothesized that INaL increases in the Ang II-mediated AF model and moricizine inhibits INaL, thereby inhibiting the activation of CaMKII under pathophysiological circumstances. Therefore, the objective of this study was to determine the therapeutic role of moricizine by INaL inhibition on Ang II-mediated AF susceptibility. Furthermore, moricizine inhibition enhances INaL and interrupts the INaL-CaMKII positive feedback loop in AF substrate generation, which may shed light on new pharmacological options in AF management. We present the following article in accordance with the ARRIVE reporting checklist (available at https://jtd.amegroups.com/article/view/10.21037/jtd-22-534/rc).
Methods
Animals
In this study, we used male wild-type C57Bl/6 mice (aged 6–8 weeks) obtained from the Cavens Experimental Animal Company (Changzhou, China). An osmotic mini pump (Alzet 2004, USA) was implanted subcutaneously for infusion of saline or Ang II (Sigma, A9525, 750 ng/kg/min) for 4 weeks (14). Moricizine (22.5 mg/kg q8h) was simultaneously administered (15). The mice were randomly assigned into the following groups: saline, Ang II, and Ang II + Moricizine, each of which included 20 mice. The researchers who the built animal models above did not participate in other experiments, and thus, researchers who participated in the remaining experiments were blinded to the grouping of the experimental animals. The mortality rate of all three groups was 0%, and there was no difference in wound healing or infection following implantation of the mini pump. Experiments were performed under a project license (No. 278) granted by the Comments, Provisions or Reservations of Animal Ethics Committee of Zhongshan Hospital, Fudan University, in compliance with the US National Institutes of Health guidelines for the care and use of animals (16). A protocol was prepared before the study without registration.
Echocardiology and in vivo electrophysiology
The mice were anesthetized by isoflurane inhalation (1.5%) on day 28 after implantation of the mini pump. Echocardiology was examined and analyzed using the Vevo 2100 System (Visual Sonics, Toronto, ON, Canada). Surface electrocardiography (ECG) were recorded in the anesthetized mice (sodium pentobarbital 50 mg/kg, intraperitoneal injection) using the BL-420 Biological Functional Experimental System (Techman, Chengdu, China). The mixture of acetylcholine (Ach) and calcium chloride (CaCl2) were prepared fresh and dissolved in saline to induce AF in mice. After recording the baseline ECG of mice, an Ach-CaCl2 mixture 10 mL/kg (Ach 25 µg/mL + CaCl2 6 mg/mL) was injected in 5s through caudal vein of mice without cardiac transient asystole (17), and ECGs were recorded 30 min after injection. AF was defined as an irregular and rapid atrial rhythm (fibrillatory baseline) with irregular RR intervals for at least 1s present on ECG. The body temperature of the mice was maintained at 37 ℃ by using a heating pad during ECG recording.
Atrial myocytes isolation
The mice were given 0.2 mL of heparin (10,000 U/10 mL) via intraperitoneal injection, which lasted 10 min for absorption. After anesthesia, the thoracic cavity of the mice was opened, and the atrial appendage was gently removed to a silicone-coated dissecting dish containing 20 mL of warmed Tyrode’s pH 7.4 solution. The atrial appendage was opened and carefully cut into 8–10 equal-sized strips (approximately 0.7 mm wide). The tissue strips were then transferred into a warm modified Tyrode’s pH 6.9 solution. After a 5 min wait, the tissue strips were washed and transferred into a tube containing enzyme solution for 30 min of incubation, and were swirled every 3–5 min. KB solution was used to terminate enzymatic digestion. After being washed three times, the atrial appendage tissue strips were mechanically dissociated and yield to a cloudy solution filled with individual atrial myocytes. Following incubation for 1 h in room temperature, these cells could be stored at 4 ℃ for up to 7 h (18).
Chemicals and Solutions
Ang II, CsCl2, Mg-ATP2, and Nifedipine were purchased from Sigma-Aldrich Co. (St. Louis, MO, USA). Sea anemone toxin II (ATX II) was purchased from Abcam (Cambridge, United Kingdom). Collagenase Type 2 was purchased from Worthington Biochemical Corporation (Lakewood, NJ, USA). Moricizine was kindly provided by Dandong pharmaceuticals company (Dandong, China). All other chemicals used in the recording solutions were obtained from regular commercial suppliers.
Tyrode’s pH 7.4 solution (in mM): 140 NaCl, 5.4 KCl, 1.2 KH2PO4, 1.8 CaCl2, 1 MgCl2, 5.55 glucose, 5 HEPES, 250 U/mL heparin (pH 7.4 with NaOH). Tyrode’s pH 6.9 solution (in mM): 140 NaCl, 5.4 KCl, 1.2 KH2PO4, 0.066 CaCl2, 18.5 glucose, 5 HEPES, taurine 49.96, 1 mg/mL BSA (pH 6.9 with NaOH). Enzyme solution: 1064U Collagenase Type 2, 5 mL Modified Tyrode’s pH 6.9. KB solution (in mM): 80 KOH, 30 KCl, 30 KH2PO4, 3 MgSO4, 50 glutamic acid, 20 taurine, 10 glucose, 0.5 EGTA and 10 HEPES (pH 7.4 with KOH). INaL was recorded with the pipette solution contained (mM): 133 CsCl, 5 NaCl, 2 Mg-ATP2, 20 TEACl, 5 HEPES, and 20 EGTA (pH 7.3 with CsOH). The bath solution contained the following (mM): 140 NaCl, 5.4 CsCl, 1.8 CaCl2, 2 MgCl2, 10 glucose, 5 HEPES, and 0.02 Nifedipine (pH 7.4 with NaOH) (19).
Patch clamp experiments
The glass electrodes were pulled with a BrownFlamming puller (P97, Sutter Instrument Co., Novato, CA, USA) and polished (MF-900, Narishige, Japan). The electrode resistance was 2–5 MΩ. The whole-cell sodium currents were recorded using an Axopatch 200B Amplifier (Molecular Devices, Inc., Sunnyvale, CA, USA), filtered at 2 kHz and sampled at 50 kHz. A 75–85% series resistance was achieved to minimize voltage errors. INaL was recorded using a 0.2 Hz and 300 ms depolarizing pulse of −90 to −20 mV (4). In the same atrial myocyte, the current densities of INaL and INaP were recorded for comparison in this study. To investigate the effects of moricizine, ATX II (3 nM) and moricizine (30 µM) (20) were perfused cumulatively on one cardiomyocyte, with each drug was lasting for about 10 min until the response reached a steady state. Recording temperatures were maintained at room temperature (22–24 ℃) in all experiments.
Western blotting
Protein samples were extracted from the left and right atrial appendage or harvested from each groups of cultured HL-1 cells. An equal quantity of protein (20–40 µg) was separated by 6% or 10% SDS-polyacrylamide gels and transferred onto PVDF membranes. Next, the membrane was blocked with 5% non-fat milk in TBST (TBS with 1% Tween 20) buffer for 2 h at room temperature and then incubated with antibodies against Nav1.5 (Proteintech Group, 23016-1-AP), CaMKII (Abcam, ab22609), phospho T286 CaMKII (p-CaMKII, Abcam, ab32678) and oxidized CaMKII (ox-CaMKII, Sigma, Met281/282) overnight at 4 ℃. The blots were then incubated with goat anti-rabbit IgG StarBright Blue 700 (Beyotime, Shanghai, China, A0208, dilution 1:1,000) for 1.5 h at room temperature and detected using the ChemiDocTMMP Imaging system. A Gel Imaging System (Tanon, Shanghai, China) and ImageJ software were used to diaplay and analyze the intensity of each band normalized to the GAPDH loading control (Cell Signaling Technology, USA, 5174S, dilution 1:10,000).
Cell culture and grouping
HL-1 cells were maintained in a basic culture media containing high glucose Dulbecco modified Eagle medium (DMEM) with 10% fetal bovine serum (FBS) and 1% penicillin/streptomycin. The cells were plated and sub-cultured until the 3rd–4th passages and then grouped as follows: (I) Control group: the HL-1 cells were cultured with a basic culture media and 0.1% dimethylsulfoxide (DMSO) without any other treatment. (II) Ang II group: the HL-1 cells were cultured with a basic culture media, 0.1% DMSO, and 1 µM Ang II (21) with deionized water solution for 24 h. (III) Ang II + Moricizine group: the HL-1 cells were cultured with basic culture media, 1 µM Ang II, and 30 µM moricizine with DMSO solution (modified to a final concentration of 0.1%) for 24 h. (IV) ATX II group: the HL-1 cells were cultured with a basic culture media, 0.1% DMSO, and 3nM ATX II with deionized water solution for 24 h. (V) ATX II + Moricizine group: the HL-1 cells were cultured with a basic culture media, 3nM ATX II, and 30 µM moricizine with DMSO solution (modified to a final concentration of 0.1%) for 24 h.
Statistical analysis
Data were analyzed using SPSS 24.0 or Graph-Pad Prism 7.0 statistical software, and presented as mean ± standard error of mean (SEM) or percentage of at least five independent experiments (21). Fischer’s exact test was used for AF susceptibility comparisons between multiple groups. One-way ANOVA followed by contrast testing was used for multiple group comparisons. Statistical significance was defined as P<0.05.
Results
Effects of moricizine on Ang II-mediated cardiac structure change
Firstly, we measured the effects of moricizine treatment on Ang II-mediated cardiac structure and function change. Echocardiography assessment (Table 1, Figure 1) demonstrated that administration of Ang II (750 ng/kg/min) for 4 weeks enlarged the left atrial diameter (P<0.05), without alteration on left ventricular volume or ejection function. Moricizine treatment inhibited Ang II-mediated enlargement of the left atrium (P<0.05), without any influence on left ventricular structure or function compared with saline control group.
Table 1
Item | Saline | Ang II | Ang II + moricizine | P value |
---|---|---|---|---|
LVESD (mm) | 2.28±0.19 | 2.70±0.18 | 4.42±0.16 | 0.243 |
LVEDD (mm) | 3.67±0.14 | 3.84±0.20 | 3.72±0.18 | 0.781 |
LVESV (µL) | 19.47±3.72 | 28.62±4.12 | 21.84±2.94 | 0.201 |
LVEDV (µL) | 58.24±5.08 | 65.87±7.21 | 60.89±5.71 | 0.670 |
Stroke volume (µL) | 38.77±2.48 | 37.25±4.21 | 39.06±3.47 | 0.924 |
EF % | 68.65±3.99 | 57.13±3.38 | 65.23±1.96 | 0.065 |
FS % | 38.57±2.35 | 29.87±2.02 | 35.45±1.96 | 0.066 |
CO (mL/min) | 19.76±1.36 | 14.79±2.30 | 16.04±2.31 | 0.296 |
LAD (mm) | 1.80±0.07 | 2.26±0.13 | 1.87±0.01 | 0.004 |
Data are presented means ± SEM; n=9 mice. LVESD, left ventricular end-systolic diameter; LVEDD, left ventricular end-diastolic diameter; LVESV, left ventricular end-systolic volume; LVEDV, left ventricular end-diastolic volume; EF, ejection fraction; FS, fractional shortening; CO, cardiac output; LAD, left atrial diameter; SEM, standard error of mean.
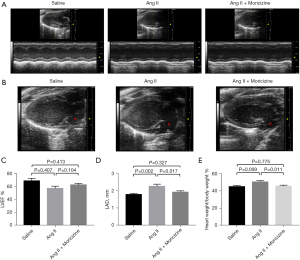
Next, we measured the body weight and heart weight of the mice. The body weight to heart weight ratio shows that Ang II administration caused the hypertrophy of heart, and moricizine treatment alleviated this effect (P<0.05). These results demonstrated that moricizine treatment reduced the atrial enlargement and cardiac hypertrophy caused by Ang II.
Effects of moricizine on Ang II-mediated atrial arrhythmogenesis
A mixture of Ach-CaCl2 was employed to induce AF via venous bolus injection. We recorded the ECG of anesthetized mice treated with saline, Ang II, or those cotreated with moricizine to assess the susceptibility and duration of AF (Figure 2A). Throughout baseline ECG recording, none of the mice in the three groups showed spontaneous AF. However, mice infused with Ang II showed significantly increased AF vulnerability compared with the saline-treated mice (33% vs. 82%, P<0.05). Compared with Ang II-treated mice (P<0.05), only 40% of the moricizine-cotreated mice were induced into AF (Figure 2B). Furthermore, the duration of AF (Figure 2C) was increased in the Ang II-treated mice (P<0.05). In contrast, mice cotreated with moricizine were resistant to the AF-inducing effect of Ang II (P<0.05). These results demonstrated that moricizine reduced Ang II-induced AF vulnerability and duration.
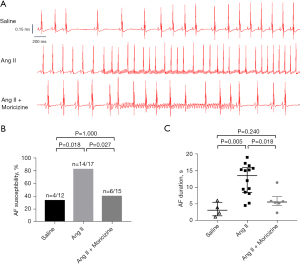
Effects of moricizine on Ang II-mediated atrial INaL enhancement
INaL plays an important role in the incidence and development of atrial fibrillation. In our experiments, the density of INaL in the left atria (Figure 3A,3B) of Ang II-treated mice was significantly increased compared with the saline-treated mice, and was reduced back to normal levels when cotreated with moricizine (0.54±0.06 vs. 0.40±0.07 pA/pF, n=8, P<0.05). Furthermore, the current density of INaL in the right atria (Figure 3C,3D) of Ang II-treated mice also increased compared with the saline-treated mice, and reduced considerably when cotreated with moricizine (1.23±0.32 vs. 0.61±0.09, pA/pF, n=8, P<0.05). However, the current density of INaL of right atrial myocytes did not decrease to normal levels in moricizine-cotreated mice (P<0.05).
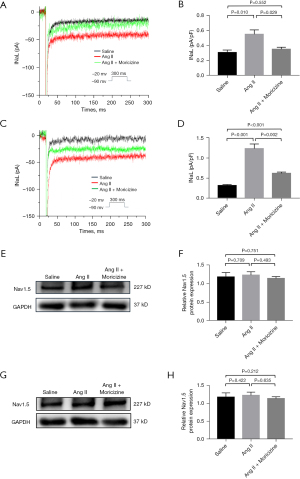
Furthermore, we measured the expression of Nav1.5 in the left and right atria of mice treated with saline, Ang II, and those cotreated with moricizine using western blotting. The results showed that the expression of Nav1.5 was not affected by Ang II or moricizine in the left atria (Figure 3E,3F) or right atria (Figure 3G,3H), which demonstrated that moricizine inhibited Ang II-mediated INaL enhancement in both atria, without alteration of Nav1.5 expression.
Effects of moricizine on the ATX II-enhanced INaL of normal atrial myocytes and protein expression alteration of HL-1 cells
In normal isolated atrial myocytes of mice, the baseline current density of INaL was 0.31±0.02 pA/pF (n=8). ATX II (3 nM) increased the current density of INaL in the same atrial myocyte to 1.44±0.03 pA/pF. Moricizine significantly reduced the current density of INaL to 0.56±0.02 pA/pF, with INaP reduced by 35.13%±3.84% (Nomornalized Blockage =64.88%±3.82%) (Figure 4A-4D). These results demonstrated that moricizine reduced the ATX II-enhanced INaL current density in normal isolated atrial myocytes via the inhibition of peak sodium current. In HL-1 cells, western blot analysis revealed that the Nav1.5 expression was similar in the control, Ang II-stimulated, and moricizine-cotreatment groups (Figure 4E,4F). Therefore, the expression of Nav1.5 was not affected by Ang II or moricizine in HL-1 cells.

Effects of moricizine on CaMKII expression in Ang II or ATX II-treated atria and HL-1 cells
Western blotting was used to measure the expression of CaMKII, p-CaMKII, and ox-CaMKII in the left and right atria of mice treated with saline, Ang II, and those cotreated with moricizine. The results showed that the expression of p-CaMKII was elevated in the Ang II-treated left atria and right atria, and was reduced when cotreated with moricizine (Figure 5). In addition, the expression of CaMKII and ox-CaMKII was not altered in any of the three groups both in the left and right atria (Figure 5), which demonstrated that moricizine inhibited Ang II-induced upregulation of p-CaMKII in the left and right atria of mice.
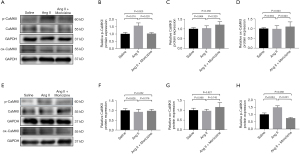
In HL-1 cells, western blot analysis revealed that moricizine reduced the upregulation of p-CaMKII induced by Ang II and ATX II, but the expression of CaMKII was similar across the three groups (Figure 6). Taken together, moricizine reduced the upregulation of p-CaMKII induced by Ang II through INaL inhibition.
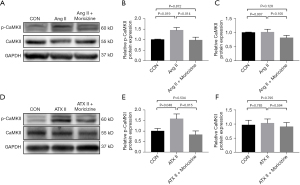
Discussion
Our study demonstrated that the current density of INaL was significantly increased in the cardiomyocytes of both atria in Ang II-treated mice, and this increase was associated with enhanced susceptibility to AF. Furthermore, our results revealed that moricizine, as a previously recognized INaP blocker, also inhibited INaL under therapeutic concentrations, thereby reducing the upregulation of p-CaMKII, collectively suppressing the induction and persistence of AF. Therefore, the main finding of this study indicated that INaL contributed to increased AF vulnerability under Ang II infusion, and the INaL blockage effect of moricizine was involved in its therapeutic effect against AF electrical and structural remodeling.
Previous investigations have indicated that the elevated Ang II is linked to the development of AF and renin-angiotensin system inhibitors are effective for the treatment of both primary AF and AF recurrence (22). Atrial electrical remodeling induced by Ang II associates with several ion channel alterations, presenting with the increase in APD in both the right and left atria. In Ang II-treated mice, INaP reduction in the left atria can lead to slow conduction; meanwhile, transient outward K+ current (Ito) and the ultra rapid delayed rectifier K+ current (Ikur) reduction in both atria could be involved in the delayed repolarization (23-25). These ion channel alterations could lead to EADs, as well as trigger the occurrence and maintain the persistence of AF. Our study showed that the enhancement of INaL in both atria contributed to a new factor of APD prolongation in the Ang II-induced AF model. Our results also indicated the increased current density of INaL in the right atria was larger than that in the left atria. The underlying mechanism for this phenomenon might lie in the significantly prolonged in depolarization duration and the beginning of repolarization in the left atria compared with the right atria, leading to the relatively reduced INaL in the left atria. Moreover, the results also showed that the INaL increases in Ang II-treated mice were consistent with the enhancement of INaL in the right atrial appendages obtained from patients with chronic AF and hypertension (26). These data suggested that the increase of INaL was a novel ionic mechanism for Ang II-induced atria electrical remodeling, which might also account for the electrical remodeling associated with the AF substrate in the development of hypertension.
Several drugs have been verified to had a moderate to high prevention of atrial fibrillation (2): according to Williams Vaughan’s classification (8), flecainide, quinidine and propafenone belong to Class I anti-arrhymia agent which mainly block inward sodium current; dronedarone, amiodarone and dofetilide belong to Class III anti-arrhymia agent which mainly block outward potassium current; sotalol belongs to beta-blocker, and it can also block outward potassium current. However, among these drugs, amiodarone has several potential long term toxic effects. Other drugs may cause QT prolongation, proarrhymia risk, potentially lead to fatal ventricular tachycardia (torsades de pointes). Therefore, optimal anti-arrhythmia agents were still crucially needed for AF patients. A recent prospective cohort study in our center (12) proved that it could also serve as an effective agent in the prevention of paroxysmal AF. Moricizine is a promise choice without serious toxic effects and the side effect of QT prolongation.
Recently, INaL inhibitors have been considered to be a new strategy in the treatment of AF (4,5) and ventricular arrhythmias (27). Our study innovatively revealed that moricizine, in addition to its inhibition of INaP, was also simultaneously an inhibitor of INaL. Consider that a 10% blockage of INaP was speculated to exert a therapeutic effect on cardiac arrhythmias (20), the 65% blockage of INaP provided by moricizine at a concentration of 30 µM undoubtedly exerted the therapeutic effect. Furthermore, when moricizine presented the effect of INaL blockage under the enhancement of ATX II, it also inhibited INaP at a similar level to that reported in previous investigations on atrial or ventricular myocytes (13,28). Several mechanisms were thought to be the manifestation of INaL, including slow inactivation of the sodium channels, single as well as bursts of late reopening sodium channels, and steady state current occurring within a window of voltage representing the overlap of steady state activation and inactivation of the sodium channels (29). The affinity of moricizine for inactivated sodium channels was higher than that of the activated channels, and moricizine predominantly binds to inactivated sodium channels with slow dissociation kinetics. This mechanism supports the INaL blockage of moricizine according to the summary mentioned above.
The current density of INaP in atrial myocytes was proven to be unchanged in paroxysmal, chronic and persistent AF patients compared with sinus rhythm patient (26,29,30). Therefore, INaP seems not to be a part of AF electrical remodeling in human atrial myocytes. The current density of INaL was larger in atrial myocytes of patients with chronic AF than in that of patients with sinus rhythm, but the current density of INaP did not differ between two groups of patients (26,29). The impact of INaL leads to prolonged action potential duration (APD) and calcium overload. Under this condition, early after depolarization (EAD) and delayed after depolarization (DAD) may likely be triggered, thereby resulting in the development of arrhythmias.
A previous study (12) indicated that INaP was reduced in the left atria of Ang II-treated mice, which contributed to a slowing of atrial conduction and a decreasing re-entry wavelength, which promoted persistent AF. In contrast, as a potent INaP blocker, moricizine cotreatment prevented AF vulnerability and persistence in Ang II-treated mice, which was consistent with the previous clinical trial in our center (12). This mechanism is probably attributable to the simultaneous blockage effect of INaL. Although moricizine also exerted a blockage in ICa-L in normal cardiomyocytes, which had antiarrhythmic effect via APD shortening (9), there were no alterations of ICa-L in either the left or right atrial myocytes in Ang II-treated mice (12). Therefore, the above evidence suggested that the blockage effect of INaL by moricizine mainly contributed to its therapeutic effect against electrical remodeling, which is associated with the AF substrate.
Following the reversion of electrical remodeling, our study indicated that moricizine also alleviated Ang II-induced atrial enlargement and cardiac hypertrophy. Recent studies have demonstrated that sustained INaL could cause cardiac structural remodeling (31-33). Aberrantly increased cellular sodium brought by INaL stimulated the reverse mode of NCX (sodium/calcium exchanger) to facilitate the influx of calcium in exchange for efflux of sodium (32). Meanwhile, an elevation of calcium concentration caused by enhanced INaL was sufficient to activate CaMKII in vivo (34). The activation of CaMKII not only triggered EAD and DAD with the enlargement of electrical remodeling, but also activated CaMKII-mediated signaling pathways related to contractile function and structural remodeling in AF (7). However, previous studies have demonstrated that p-CaMKII in its activated state could phosphorylate Nav1.5 under relevant pathological conditions, which leads to chronic INaL augmentation (33,35). Therefore, sustained INaL modulation of CaMKII in turn promotes INaL, which creates a positive feedback loop. This positive feedback loop may lead to AF structural remodeling in an Ang II-induced AF model.
In the Ang II-induced AF model, Nav1.5 expression was neither affected by Ang II infusion nor by the therapeutic effect of moricizine, as the modification of Nav1.5 protein was phosphorylation. The downregulation of p-CaMKII caused by moricizine treatment was consistent between Ang II and ATX II interventions, which demonstrated that moricizine reduced Ang II-induced CaMKII activation via INaL inhibition. Therefore, moricizine interrupts the INaL-CaMKII positive feedback loop in AF substrate generation, which ultimately attenuates Ang II-induced atrial enlargement and cardiac hypertrophy.
The current study has several limitations that should be noted. Firstly, although we used Ach-CaCl2 to induce AF in mice, a 1.2F octopolar electrophysiology catheter inserted into right heart to provoke AF is a more standard method. We will verify the AF susceptibility in our article when the experimental conditions permit. Secondly, further investigation regarding more ion channel-related mechanisms of moricizine in AF treatment is required.
In conclusion, Ang II causes the enhancement of INaL, further creating an electrical and structural substrate for AF. Administrating both acutely and chronically, moricizine inhibits INaL, further suppresses CaMKII activation, and exerts the combined therapeutic effect of reducing AF electrical and structural remodeling. Therefore, moricizine may be a promising pharmacological option for the treatment of AF. Further randomized control trials concerning the therapeutic effect of AF need to be conducted to determine a more reliable indication of moricizine.
Acknowledgments
We are grateful to Feng Li from the Department of Cardiology, The Affiliated Wuxi People’s Hospital of Nanjing Medical University for his assistance on the research and manuscript preparation. We are also grateful to Wei Li and Guojian Fang from the Xin Hua Hospital Affiliated to Shanghai Jiao Tong University School of Medicine for their guidance regarding the patch clamp protocol and technology.
Funding: This work was supported by the Scientific Research and Development Fund of Zhongshan Hospital, Fudan University (No. 331), the Shanghai Municipal Commission of Economy and Informatization (No. GYQJ-2018-2-05), and the Project of Shanghai Science and Technology Committee (No. 21S31906902).
Footnote
Reporting Checklist: The authors have completed the ARRIVE reporting checklist. Available at https://jtd.amegroups.com/article/view/10.21037/jtd-22-534/rc
Data Sharing Statement: Available at https://jtd.amegroups.com/article/view/10.21037/jtd-22-534/dss
Conflicts of Interest: All authors have completed the ICMJE uniform disclosure form (available at https://jtd.amegroups.com/article/view/10.21037/jtd-22-534/coif). The authors have no conflicts of interest to declare.
Ethical Statement: The authors are accountable for all aspects of the work in ensuring that questions related to the accuracy or integrity of any part of the work are appropriately investigated and resolved. Experiments were performed under a project license (No. 278) granted by the Comments, Provisions or Reservations of Animal Ethics Committee of Zhongshan Hospital, Fudan University, in compliance with the US National Institutes of Health guidelines for the care and use of animals.
Open Access Statement: This is an Open Access article distributed in accordance with the Creative Commons Attribution-NonCommercial-NoDerivs 4.0 International License (CC BY-NC-ND 4.0), which permits the non-commercial replication and distribution of the article with the strict proviso that no changes or edits are made and the original work is properly cited (including links to both the formal publication through the relevant DOI and the license). See: https://creativecommons.org/licenses/by-nc-nd/4.0/.
References
- DeLago AJ, Essa M, Ghajar A, et al. Incidence and Mortality Trends of Atrial Fibrillation/Atrial Flutter in the United States 1990 to 2017. Am J Cardiol 2021;148:78-83. [Crossref] [PubMed]
- Michaud GF, Stevenson WG. Atrial Fibrillation. N Engl J Med 2021;384:353-61. [Crossref] [PubMed]
- Horváth B, Hézső T, Kiss D, et al. Late Sodium Current Inhibitors as Potential Antiarrhythmic Agents. Front Pharmacol 2020;11:413. [Crossref] [PubMed]
- Chu Y, Yang Q, Ren L, et al. Late Sodium Current in Atrial Cardiomyocytes Contributes to the Induced and Spontaneous Atrial Fibrillation in Rabbit Hearts. J Cardiovasc Pharmacol 2020;76:437-44. [Crossref] [PubMed]
- Burashnikov A, Petroski A, Hu D, et al. Atrial-selective inhibition of sodium-channel current by Wenxin Keli is effective in suppressing atrial fibrillation. Heart Rhythm 2012;9:125-31. [Crossref] [PubMed]
- Takla M, Huang CL, Jeevaratnam K. The cardiac CaMKII-Nav1.5 relationship: From physiology to pathology. J Mol Cell Cardiol 2020;139:190-200. [Crossref] [PubMed]
- Liu Z, Finet JE, Wolfram JA, et al. Calcium/calmodulin-dependent protein kinase II causes atrial structural remodeling associated with atrial fibrillation and heart failure. Heart Rhythm 2019;16:1080-8. [Crossref] [PubMed]
- Vaughan Williams EM. The experimental basis for the choice of an anti-arrhythmic drug. Adv Cardiol 1970;4:275-89. [Crossref] [PubMed]
- Yamane T, Sunami A, Sawanobori T, et al. Use-dependent block of Ca2+ current by moricizine in guinea-pig ventricular myocytes: a possible ionic mechanism of action potential shortening. Br J Pharmacol 1993;108:812-8. [Crossref] [PubMed]
- Rosenshtraukh LV, Anyukhovsky EP, Nesterenko VV, et al. Electrophysiologic aspects of moricizine HCl. Am J Cardiol 1987;60:27F-34F. [Crossref] [PubMed]
- Ahmmed GU, Hisatome I, Kurata Y, et al. Analysis of moricizine block of sodium current in isolated guinea-pig atrial myocytes. Atrioventricular difference of moricizine block. Vascul Pharmacol 2002;38:131-41. [Crossref] [PubMed]
- Zhu W, Chen Q, Liu Y, et al. Effect of moricizine in patients with paroxysmal atrial fibrillation. Chinese Journal of Cardiac Arrhythmias 2012;16:407-10.
- Jansen HJ, Mackasey M, Moghtadaei M, et al. Distinct patterns of atrial electrical and structural remodeling in angiotensin II mediated atrial fibrillation. J Mol Cell Cardiol 2018;124:12-25. [Crossref] [PubMed]
- Song S, Zhang R, Mo B, et al. EZH2 as a novel therapeutic target for atrial fibrosis and atrial fibrillation. J Mol Cell Cardiol 2019;135:119-33. [Crossref] [PubMed]
- PINKEL D. The use of body surface area as a criterion of drug dosage in cancer chemotherapy. Cancer Res 1958;18:853-6. [PubMed]
- Bayne K. Revised Guide for the Care and Use of Laboratory Animals available. American Physiological Society. Physiologist 1996;39:199-208-11. [PubMed]
- Gao Y, Li P, Ma LX, et al. Effects of acute administration of ethanol on experimental arrhythmia. Chin J Physiol 2012;55:307-13. [PubMed]
- Jansen HJ, Rose RA. Isolation of Atrial Myocytes from Adult Mice. J Vis Exp 2019; [Crossref] [PubMed]
- Jin X, Jiang Y, Xue G, et al. Increase of late sodium current contributes to enhanced susceptibility to atrial fibrillation in diabetic mice. Eur J Pharmacol 2019;857:172444. [Crossref] [PubMed]
- Saeki T, Uchida T, Nishimura M, et al. Block of Na+ channel by moricizine hydrochloride in isolated feline ventricular myocytes. Eur J Pharmacol 1994;261:249-56. [Crossref] [PubMed]
- Yang Y, He J, Yuan M, et al. Xanthine oxidase inhibitor allopurinol improves atrial electrical remodeling in diabetic rats by inhibiting CaMKII/NCX signaling. Life Sci 2020;259:118290. [Crossref] [PubMed]
- Curtis MJ, Alexander S, Cirino G, et al. Experimental design and analysis and their reporting II: updated and simplified guidance for authors and peer reviewers. Br J Pharmacol 2018;175:987-93. [Crossref] [PubMed]
- Ma H, Jiang H, Feng J, et al. Angiotensin Receptor Blocker and Calcium Channel Blocker Preventing Atrial Fibrillation Recurrence in Patients with Hypertension and Atrial Fibrillation: A Meta-analysis. Cardiovasc Ther 2021;2021:6628469. [Crossref] [PubMed]
- Li MY, Chen HX, Hou HT, et al. Biomarkers and key pathways in atrial fibrillation associated with mitral valve disease identified by multi-omics study. Ann Transl Med 2021;9:393. [Crossref] [PubMed]
- Heijman J, Voigt N, Nattel S, et al. Cellular and molecular electrophysiology of atrial fibrillation initiation, maintenance, and progression. Circ Res 2014;114:1483-99. [Crossref] [PubMed]
- Poulet C, Wettwer E, Grunnet M, et al. Late Sodium Current in Human Atrial Cardiomyocytes from Patients in Sinus Rhythm and Atrial Fibrillation. PLoS One 2015;10:e0131432. [Crossref] [PubMed]
- Fukaya H, Plummer BN, Piktel JS, et al. Arrhythmogenic cardiac alternans in heart failure is suppressed by late sodium current blockade by ranolazine. Heart Rhythm 2019;16:281-9. [Crossref] [PubMed]
- Liu W, Clarkson CW, Yamasaki S, et al. Characterization of the rate-dependent effects of ethmozine on conduction, in vivo, and on the sodium current, in vitro, in the newborn heart. J Pharmacol Exp Ther 1992;263:608-16. [PubMed]
- Burashnikov A, Antzelevitch C. Role of late sodium channel current block in the management of atrial fibrillation. Cardiovasc Drugs Ther 2013;27:79-89. [Crossref] [PubMed]
- Voigt N, Heijman J, Wang Q, et al. Cellular and molecular mechanisms of atrial arrhythmogenesis in patients with paroxysmal atrial fibrillation. Circulation 2014;129:145-56. [Crossref] [PubMed]
- Glynn P, Musa H, Wu X, et al. Voltage-Gated Sodium Channel Phosphorylation at Ser571 Regulates Late Current, Arrhythmia, and Cardiac Function In Vivo. Circulation 2015;132:567-77. [Crossref] [PubMed]
- Nie J, Duan Q, He M, et al. Ranolazine prevents pressure overload-induced cardiac hypertrophy and heart failure by restoring aberrant Na+ and Ca2+ handling. J Cell Physiol 2019;234:11587-601. [Crossref] [PubMed]
- Coppini R, Ferrantini C, Yao L, et al. Late sodium current inhibition reverses electromechanical dysfunction in human hypertrophic cardiomyopathy. Circulation 2013;127:575-84. [Crossref] [PubMed]
- Bers DM, Guo T. Calcium signaling in cardiac ventricular myocytes. Ann N Y Acad Sci 2005;1047:86-98. [Crossref] [PubMed]
- Yao L, Fan P, Jiang Z, et al. Nav1.5-dependent persistent Na+ influx activates CaMKII in rat ventricular myocytes and N1325S mice. Am J Physiol Cell Physiol 2011;301:C577-86. [Crossref] [PubMed]
(English Language Editor: A. Kassem)