Association between hospital-acquired pneumonia and proton pump inhibitor prophylaxis in patients treated with glucocorticoids: a retrospective cohort study based on 307,622 admissions in China
Introduction
Non-ventilator hospital-acquired pneumonia (HAP) is a serious nosocomial infection that is generally under-studied compared to ventilator-associated pneumonia. Recent studies have shown that the incidence of this disease is 0.12–2.28 per 1,000 patient admissions in the U.S. acute care hospital setting and 0.95 per 100 admissions in the Portuguese National Health System hospitals (1,2). The incidence of HAP is closely related to a high mortality rate and high medical burden. In China, Yin et al. (3) conducted a 10-year prospective observational study of HAP and found that 58% of cases with nosocomial pneumonia were HAP and the in-hospital all-cause mortality rate of HAP was 8.8%. Another study in Guangzhou, China, found an 18.5 percent 30-day mortality rate for HAP (4).
Proton pump inhibitors (PPIs) are widely used in clinical practice. However, the long-term adverse effects of PPIs include community-acquired pneumonia, fracture, kidney injury, dementia, vitamin deficiency, and other drug-induced disease (5-8). However, the short-term hazards of PPIs in hospitalized patients usually receive less attention. Some studies have suggested that HAP may be one of the short-term adverse reactions of PPIs (9-11). Herzig et al. (12) conducted a large prospective cohort study to explore whether administration of PPIs increased the risk of HAP in the general ward and found that the odds ratio (OR) of HAP between the PPIs exposed group and unexposed group was 1.3 [95% confidence interval (CI): 1.1 to 1.4]. A meta-analysis which enrolled 5 case-control studies, 3 cohort studies, and 23 randomized controlled trials (RCTs) also showed that the risk of HAP associated with using PPIs was higher than that of the control group (OR: 1.27, 95% CI: 1.11 to 1.46) (13). However, a recent RCT involving 3,298 patients showed no statistically significant difference in the incidence of pneumonia between the pantoprazole and placebo groups in the intensive care unit (14).
In China, glucocorticoid (GC) therapy is frequently combined with the use of PPIs and can result in the overuse of PPIs (15,16). In a German study, the combination of the 2 was also one of the main reasons for the inappropriate use of PPIs (17). Since the use of GC therapy may be associated with the occurrence of nosocomial infection, we aimed to understand whether the combined use of PPIs and GC therapy increased the risk of HAP, which has not been reported in previous studies. The incidence of the HAP is low, and the reported OR (if significant) in many studies is usually less than 2, therefore studies need a large cohort to maintain the test power and detect a change between exposed and unexposed patients. Inadequate sample sizes may have led many previous studies into a type II error in which they failed to detect an association between drug use and HAP risk. The HAP risk associated with the widespread use of PPIs in patients treated with GC therapy has not been effectively assessed in the Chinese hospital-care setting. Therefore, we conducted this study with the aim of exploring whether the use of PPIs in patients treated with GC therapy causes additional HAP risk, if such risk exists, and to understand the optimal dose to not increase HAP risk if PPI therapy is required. We present the following article in accordance with the STROBE reporting checklist (available at https://jtd.amegroups.com/article/view/10.21037/jtd-21-1886/rc).
Methods
Data source
Data was collected from the Medication Data Management Center of Shanghai Changhai Hospital. The database in this platform contains more than 100 general hospitals in China and generates almost 15 million electronic medical records per year. The database is based on structured data of hospital electronic medical records of inpatients in these hospitals. Its main application is to monitor the use of antibiotics in the region, but it includes all patients who use or do not use antibiotics. Therefore, this database can be used to conduct research on other medicines or diseases. The information available includes demographic characteristic, clinical diagnosis based on the International Classification of Diseases 10th version (ICD-10) code, medication records, and laboratory test results. Previous studies have provided detailed descriptions and explanations of this database (18,19).
Design and study population
We designed a large, retrospective cohort study to explore the risk of HAP between the exposed group (GC therapy combined with PPI prophylaxis) and the unexposed group (GC therapy only). The study population was drawn from 20 general hospitals which were all large-volume and had similar numbers of admissions. The study date was from 1 January, 2015 to 31 March, 2018. The inclusion criteria were as follows: (I) adult patients (age ≥18 years) and all visits were the first for each admission; (II) length of stay (LOS) was more than 3 days but less than 100 days; (III) the patient had at least 1 payment record for systemic GC therapy [anatomical therapeutic chemical (ATC) code: H02AB] during hospitalization. The exclusion criteria were as follows: (I) time to the first dose of GC therapy >72 hours since admission; (II) continuous mechanical ventilation (time of duration >48 hours); (III) the patients had a payment record of histamine-2 receptor antagonist during hospitalization; (IV) the patient was admitted with bacterial pneumonia (ICD-10: J13.x-J18.x).
All data used in this study had only been used for scientific research and preserved by reliable measures.
The study was conducted in accordance with the Declaration of Helsinki (as revised in 2013). The database used in this study is anonymous and delinked and the researchers were unable to identify the patients, therefore the ethical review and individual consent for this retrospective analysis was waived by the Ethics Committee of Shanghai Fourth People’s Hospital Affiliated to Tongji University School of Medicine.
Medication exposure and outcome
Medication exposure of an inpatient was defined as at least 1 dose of PPIs including omeprazole, lansoprazole, pantoprazole, rabeprazole, or esomeprazole according to the payment record during hospitalization. The exposure time of PPIs was defined as the billable time. We calculated the exposure dose from the summary of the defined daily dose (DDD). The DDD of omeprazole, lansoprazole, pantoprazole, rabeprazole, and esomeprazole were made with reference to the stipulations of the World Health Organization (20): 20, 30, 40, 20, and 30 mg, respectively.
The outcome in our study was HAP (ventilator associated pneumonia and aspiration pneumonia were not included). We defined HAP as patients who had a discharge diagnosis of bacterial pneumonia (ICD-10: J13.x-J18.x) which did not exist in their admission diagnosis lists. During the study participants’ inclusion and exclusion, we specified that patients on continuous mechanical ventilation (have a ventilator use record, and the total time ≥2 hours) were excluded so that our outcomes would focus on non-ventilator-associated pneumonia. Aspiration pneumonia was usually diagnosed as “pneumonitis due to inhalation of solids and liquids (ICD-10: J69)”, “pneumonitis due to aspiration of food and vomit (ICD-10: J69.0)”, or “aspiration pneumonia (ICD-10: J69.001)” in the database.
Covariates
We incorporated the covariates which might be associated with exposure or outcome as much as possible to decrease confounding factors. They included demographic and admission characteristics, such as gender, age, and admission season. Comorbidities comprised the diseases in the Charlson Score Index (CCI) (21). We compiled comorbidities into ICD-10 code in line with previous research (22). Other diseases or symptoms related to the use of PPIs or HAP included alcohol-related disease, colon disease, hypertension, mental disorder, neuromuscular disorder, gastrointestinal bleeding, and abdominal symptoms. Stress ulcer prophylaxis had a significant impact on the use of PPIs. Therefore, we summarized some characteristics as a covariate called high stress ulcer risk, including traumatic brain injury, traumatic spinal cord injury, burn, sepsis, or coagulopathy. Medication therapy also had an influence on our study; we extracted data for this from the payment record by ATC code, including antithrombotic agents (like vitamin K antagonists, heparin group, platelet aggregation inhibitors, and others), non-selective non-steroidal anti-inflammatory drugs (NSAIDs), coxibs, antidepressants, sedatives, muscle relaxants, bisphosphonates, antineoplastic agents, and antibiotic use in the first 48 hours after admission. Moreover, high dose GC therapy (more than 250 mg hydrocortisone or equivalent), transient mechanical ventilation (less than 48 hours), and LOS were also considered (Table S1).
Statistical analysis
The features of the PPIs exposed and unexposed groups were evaluated through descriptive analysis. Continuous data were presented as their median and interquartile range, while categorical data was counted by frequency and percentage. A multiple logistic regression model was performed to analyze the HAP risk between the PPIs exposed and unexposed groups, and the risk was presented by OR and its 95% CI.
To calculate the propensity score, we used PPIs exposure as a dependent variable and the variable set shown in Table S2 as covariates, then established a logistic regression model. The propensity score reflected the probability of exposure for every patient, and we matched the exposed and unexposed groups in a 1:1 ratio with the greedy matching algorithm (23). The algorithm performed multiple rounds of matching according to the decimal accuracy of the propensity score, and the calipers value in each round of matching was 0.00001, 0.0001, 0.001, 0.01, 0.1, respectively. This method was shown to be effective in previous similar studies (12,24). We compared the difference between the PPIs exposed and unexposed groups before and after propensity score matching (PSM) by testing the standardized difference (Table 1). The difference between the groups was acceptable when the absolute standardized difference was less than 0.1. We used the absolute standardized difference instead of P value because previous research had shown that it did better than P value while PSM was deployed (25,26). Then, we applied a conditional logistics regression (if some covariates remained unbalanced) and analyzed the risk of HAP, including OR, 95% CI, and population-attributable risk based on the cohort after PSM. The covariates used were those that had unbalanced residuals (absolute standardized difference ≥0.1, if it existed) as shown in Table 1.
Table 1
Variables | PPIs exposure group (n=83,786) | Unexposed group (n=83,786) | Absolute standardized difference |
---|---|---|---|
Male, n (%) | 44,420 (53.0) | 44,766 (53.4) | −0.008 |
Age, median (IQR), years | 49 (37–61) | 49 (37–61) | −0.016 |
Age, mean ± SD, years | 49.4±15.9 | 49.6±16.3 | |
Admission season, n (%) | |||
Spring | 21,982 (26.2) | 21,987 (26.2) | 0.000 |
Summer | 19,390 (23.1) | 19,524 (23.3) | −0.004 |
Autumn | 19,402 (23.2) | 19,580 (23.4) | −0.005 |
Winter | 23,012 (27.5) | 22,695 (27.1) | 0.008 |
LOS, median (IQR), days | 8 (6–11) | 8 (6–10) | 0.031 |
Comorbidity, n (%) | |||
Myocardial infarction | 1,110 (1.3) | 1,226 (1.5) | −0.012 |
Congestive heart failure | 1,972 (2.4) | 1,987 (2.4) | −0.001 |
Peripheral vascular disease | 1,390 (1.7) | 1,399 (1.7) | −0.001 |
Cerebrovascular disease | 5,261 (6.3) | 5,392 (6.4) | −0.006 |
Dementia | 67 (0.1) | 66 (0.1) | 0.000 |
Chronic pulmonary disease | 4,476 (5.3) | 4,419 (5.3) | 0.003 |
Rheumatic disease | 1,592 (1.9) | 1,541 (1.8) | 0.004 |
Peptic ulcer disease | 228 (0.3) | 154 (0.2) | 0.019 |
Mild liver disease | 4,736 (5.7) | 4,628 (5.5) | 0.006 |
Diabetes without chronic complication | 5,841 (7.0) | 5,890 (7.0) | −0.002 |
Hemiplegia or paraplegia | 180 (0.2) | 189 (0.2) | −0.002 |
Renal disease | 1,987 (2.4) | 2,027 (2.4) | −0.003 |
Diabetes with chronic complication | 473 (0.6) | 430 (0.5) | 0.007 |
Malignancy | 7,826 (9.3) | 8,138 (9.7) | −0.013 |
Moderate or severe liver disease | 392 (0.5) | 355 (0.4) | 0.007 |
Metastatic solid tumor | 2,094 (2.5) | 2,194 (2.6) | −0.008 |
AIDS | 56 (0.1) | 55 (0.1) | 0.000 |
High stress ulcer risk | 7,568 (9.0) | 7,441 (8.9) | 0.005 |
Alcohol-related disease | 86 (0.1) | 53 (0.1) | 0.014 |
Colon disease | 289 (0.3) | 288 (0.3) | 0.000 |
Hypertension | 13,822 (16.5) | 14,327 (17.1) | −0.016 |
Mental disorder | 558 (0.7) | 529 (0.6) | 0.004 |
Neuromuscular disorder | 1,473 (1.8) | 1,416 (1.7) | 0.005 |
Gastrointestinal bleeding | 102 (0.1) | 57 (0.1) | 0.017 |
Abdominal symptoms | 607 (0.7) | 579 (0.7) | 0.004 |
Medication, n (%) | |||
Vitamin K antagonists | 251 (0.3) | 258 (0.3) | −0.002 |
Heparin group | 9,098 (10.9) | 8,917 (10.6) | 0.007 |
Platelet aggregation inhibitors | 7,270 (8.7) | 7,191 (8.6) | 0.003 |
Other antithrombotic agents | 3,353 (4.0) | 3,406 (4.1) | −0.003 |
Non-selective NSAIDs | 22,893 (27.3) | 23,020 (27.5) | −0.003 |
Antidepressants | 617 (0.7) | 593 (0.7) | 0.003 |
Bisphosphonates | 1,491 (1.8) | 1,467 (1.8) | 0.002 |
Antineoplastic agents | 3,385 (4.0) | 3,292 (3.9) | 0.006 |
Coxibs | 14,720 (17.6) | 15,229 (18.2) | −0.016 |
Sedatives | 32,161 (38.4) | 32,409 (38.7) | −0.006 |
Muscle relaxants | 32,644 (39.0) | 32,856 (39.2) | −0.005 |
Antibiotic therapy in the 48 hours after admission | 44,429 (53.0) | 44,495 (53.1) | −0.002 |
GC therapy dose >250 mg hydrocortisone or equivalent | 67,685 (80.8) | 66,612 (79.5) | 0.032 |
Transient mechanical ventilation* | 29 (0.0) | 15 (0.0) | 0.010 |
*, total duration of mechanical ventilation ≤48 hours. PPIs, proton pump inhibitors; LOS, length of stay; IQR, interquartile range; AIDS, acquired immune deficiency syndrome; NSAIDs, non-steroidal anti-inflammatory drugs; GC, glucocorticoid.
If PPIs exposure increased the HAP risk when patients were treated with GC therapy, we subsequently performed dose-effect relationship analysis to explore the detail of the risk and the numbers needed to harm (NNHs) for different PPIs dose groups. We performed a multiple logistic regression model to adjust the confounding factor (unbalanced variable, as shown in Table S2) and analyzed the independent risk of PPIs for different PPI doses. In terms of DDDs, the cumulative PPI doses which had been prescribed during hospitalization were classified into 3 groups: low-dose (0–2 DDDs), medium-dose (2–7 DDDs), and high-dose (>7 DDDs).
A 0.05 level of significance in a 2-tailed hypothesis test was used in this study, and all the statistical analysis was conducted by SAS 9.4 (SAS Institute, Cary, NC, USA).
Sensitivity analysis
To address the impact of misclassification on the study, 2 sensitivity analyses were performed. First, some patients with community-acquired pneumonia or in the incubation period may not be accurately diagnosed on admission. These patients were diagnosed with other conditions, such as upper respiratory tract infections, at the time of admission. According to our original definition, if these patients had a diagnosis of bacterial pneumonia at discharge, they would be classified as HAP, which led to misclassification and overstated the incidence of HAP. Therefore, we repeated the main analysis after adding an additional condition to the original outcome, which was that a patient received no antibiotic therapy in the first 48 hours after admission. Doing so made the calculated incidence of HAP lower than the true incidence of HAP and the risk of exposure to HAP more difficult to detect, but our sample size was sufficient to detect a small risk (if any). If the main effect of exposure factors remained statistically significant after changing the definition of the outcome, our statistical model and results could be considered to be relatively robust.
In our study, the lack of an obvious time point for HAP occurrence (which was not available from the database) led to a portion of unrelated PPI use being misclassified as exposure (PPI use occurred later than HAP). To address this issue, we performed another sensitivity analysis by changing the definition of exposure and classifying patients whose first use of PPIs was later than 48 hours after admission to the unexposed group. Since HAP always occurs 48 hours after admission, redefinition of exposure ensured that all exposures occurred before the outcome. In addition, we determined when all PPI users first used PPIs to analyze the possibility of exposure to misclassification and how it might affect the results.
Results
Study population
From 1 January, 2015, to 31 March, 2018, there were 701,673 adult patients who satisfied the inclusion criteria discharged from the 20 general hospitals in our database. After excluding GC therapy administration 72 hours later (n=369,589), continuous mechanical ventilation (n=1,135), histamine-2 receptor antagonist administration (n=19,342), and admission with bacterial pneumonia (n=3,958), the final analytic cohort included 307,622 admissions (Figure 1). The median age of the final cohort was 51 years, with an interquartile range of 40 to 62 years. A total of 167,535 (54.5%) admissions of the cohort were male, and 217,460 (70.7%) admissions received at least 1 dose of PPIs during hospitalization. There were significant differences (standardized difference >0.1) between the PPIs exposed and unexposed groups in aspects of their baseline characteristics (Table S2).
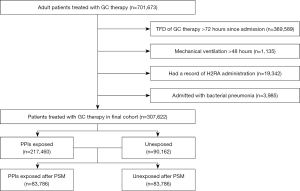
After PSM in a ratio of 1:1 between the PPIs exposed and unexposed groups, both groups included 83,786 admissions. The density of exposure probability of the groups was similar after PSM, and there was no unbalanced residual in all aspect of characteristics in the cohort after PSM (Figure 2, Table 1).
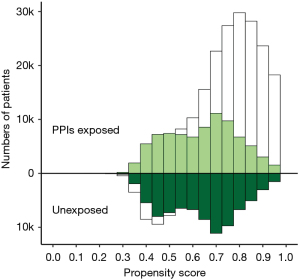
Covariate adjusted and PSM
In the PPIs exposed group, HAP occurred in 5,626 admissions (2.6%), and in 1,292 admissions (1.4%) in the unexposed group. We performed a simple logistic regression, and the crude OR was 1.8 (95% CI: 1.7 to 1.9). To control for confounding factors, we adjusted the covariates which had unbalanced residuals, as shown Table S2, using a multiple logistic regression model. The adjusted OR was 1.7 (95% CI: 1.5 to 1.8).
After PSM, HAP occurred in 1,795 admissions (2.1%) in the exposed group and in 1,286 admissions (1.5%) in the unexposed group, and the risk of HAP increased (OR: 1.4, 95% CI: 1.3 to 1.5). Then, we calculated the population attributable risk percent by the incidence of HAP in the unexposed group and in the total population base on the cohort after PSM. The population attributable risk was 17.2%, which showed that much of HAP might be attributed to administration of PPIs in patients treated with GC therapy (Table 2).
Table 2
Category | Before PSM | After PSM | |||||||
---|---|---|---|---|---|---|---|---|---|
No. | HAP, n (%) | Crude OR (95% CI) | Adjusted OR (95% CI)* | No. | HAP, n (%) | OR (95% CI) | PAR (%) | ||
PPIs exposed | 217,460 | 5,626 (2.6) | 1.8 (1.7–1.9) | 1.7 (1.5–1.8) | 83,786 | 1,795 (2.1) | 1.4 (1.3–1.5) | 17.2 | |
Unexposed | 90,162 | 1,292 (1.4) | 83,786 | 1,268 (1.5) | |||||
Total | 307,622 | 6,918 (2.2) | 167,572 | 3,063 (1.8) |
*, a multiple logistic regression model was performed and the OR was adjusted by covariables set which had unbalanced residuals (absolute standardized difference >0.1) including muscle relaxants, length of stay, sedatives, malignancy, antineoplastic agents, high stress ulcer risk, coxibs, heparin group, non-steroidal anti-inflammatory drugs, male, antibiotic therapy in the 48 hours after admission, peptic ulcer disease, myocardial infarction, gastrointestinal bleeding, and congestive heart failure. HAP, hospital-acquired pneumonia; PPIs, proton pump inhibitors; GC, glucocorticoid; PSM, propensity score matching; OR, odds ratio; CI, confidence interval; PAR, population-attributable risk.
Dose-effect relationship analysis
We calculated the DDDs of PPIs for every PPIs exposed patient in the original cohort during hospitalization and then divided cases into 3 dose groups: low-dose [0–2], medium-dose [2–7], and high-dose [>7]. There were 33,923 admissions (15.6%), 61,794 admissions (28.4%), and 121,743 (56.0%) admissions in the low-, medium- and high-dose groups, respectively.
The HAP risk among the 3 groups was likely different. We performed a multiple logistic regression model to adjust the confounding factor (unbalanced variable in Table S2, such as length of stay) and analyzed the independent risk of PPIs for different PPIs doses. In the low-dose group, only 369 admissions (1.1%) of HAP occurred and the adjusted OR was not statistically significant (OR: 1.0, 95% CI: 0.9 to 1.1) compared to the unexposed group. A total of 989 admissions (1.6%) of HAP occurred in the medium-dose group, and the adjusted OR was 1.3 (95% CI: 1.2 to 1.4) compared to the unexposed group. The NNHs of HAP in the medium-dose group were 243. To our surprise, the risk of HAP greatly increased in the high-dose group. A total of 4,268 admissions (3.5%) of HAP occurred, and the adjusted OR was 1.9 (95% CI: 1.8 to 2.1). The NNHs of HAP in the high-dose group were only 82 (Table 3).
Table 3
Cumulative PPIs dose | Admission No. | HAP, n (%) | Adjusted OR* (95%CI) | NNHs |
---|---|---|---|---|
0 | 90,162 | 1,292 (1.4) | 1* | – |
0< DDDs ≤2 | 33,923 | 369 (1.1) | 1.0 (0.9–1.1) | – |
2< DDDs ≤7 | 61,794 | 989 (1.6) | 1.3 (1.2–1.4) | 243 |
DDDs >7 | 121,743 | 4,268 (3.5) | 1.9 (1.8–2.1) | 82 |
*, OR was adjusted by covariables set which had unbalanced residuals (absolute standardized difference >0.1) including muscle relaxants, length of stay, sedatives, malignancy, antineoplastic agents, high stress ulcer risk, coxibs, heparin group, non-steroidal anti-inflammatory drugs, male, antibiotic therapy in the 48 hours after admission, peptic ulcer disease, myocardial infarction, gastrointestinal bleeding, and congestive heart failure. PPIs, proton pump inhibitors; HAP, hospital acquired pneumonia; GC, glucocorticoid; OR, odds ratio; CI, confidence interval; NNHs, numbers needed to harms; DDD, defined daily dose.
Sensitivity analysis
After we restricted the definition of the outcome, the HAP case numbers dropped to 1,281 (0.6%) in the PPIs exposed group and 354 (0.4%) in the unexposed group. The risk of HAP increased when exposed to PPIs (crude OR: 1.5, 95% CI: 1.4 to 1.8). After adjusting for covariates and PSM, the effect of PPIs was unchanged. The OR was 1.6 (95% CI: 1.4 to 1.8) and 1.3 (95% CI: 1.1 to 1.5), respectively (Table 4).
Table 4
Category | Before PSM | After PSM | |||
---|---|---|---|---|---|
HAP (%) | Adjusted OR* (95% CI) | HAP (%) | OR (95% CI) | ||
PPIs exposed | 0.6 | 1.6 (1.4–1.8) | 0.5 | 1.3 (1.1–1.5) | |
Unexposed | 0.4 | – | 0.4 |
*, the OR was adjusted by covariables set which had unbalanced residuals (absolute standardized difference >0.1) including muscle relaxants, length of stay, sedatives, malignancy, antineoplastic agents, high stress ulcer risk, coxibs, heparin group, non-steroidal anti-inflammatory drugs, male, antibiotic therapy in the 48 hours after admission, peptic ulcer disease, myocardial infarction, gastrointestinal bleeding, and congestive heart failure. HAP, hospital-acquired pneumonia; PPIs, proton pump inhibitors; GC, glucocorticoid; PSM, propensity score matching; OR, odds ratio; CI, confidence interval.
Among the patients prescribed PPIs during hospitalization, 141,746 (65.2%) were prescribed within 48 hours after admission. After we redefined PPIs exposure as their consumption within the first 48 hours of hospitalization and performed a logistic regression model, the OR associated with the HAP between the PPIs exposure and the unexposed groups remained statistically significant (OR: 1.3, 95% CI: 1.2 to 1.3) (Table 5).
Table 5
Category | Before PSM | After PSM | |||
---|---|---|---|---|---|
HAP (%) | Adjusted OR* (95% CI) | HAP (%) | OR (95% CI) | ||
PPIs exposed | 3.1 | 1.4 (1.3–1.5) | 2.5 | 1.3 (1.2–1.3) | |
Unexposed | 1.5 | – | 2.0 | – |
*, the OR was adjusted by covariables set which had unbalanced residuals including age, sex, length of stay, myocardial infarction, congestive heart failure, cerebrovascular disease, peptic ulcer disease, high stress ulcer risk, hypertension, gastrointestinal bleeding, heparin group, platelet aggregation inhibitors, other antithrombotic agents, antineoplastic agents and antibiotic therapy in in the 48 hours after admission. HAP, hospital acquired pneumonia; PPIs, proton pump inhibitors; GC, glucocorticoid; PSM, propensity score matching; OR, odds ratio; CI, confidence interval.
Discussion
We conducted a large retrospective cohort study which included 307,622 adult admissions from Medication Data Management Center of Shanghai Changhai Hospital. All admissions included in this study had been ordered GC therapy in the first 72 hours of hospitalization. We calculated the risk of HAP in the PPIs exposed compared to the PPIs unexposed groups using multiple logistic regression and PSM. The results showed that PPI prophylaxis for GC therapy treatment increased the risk of HAP, and this risk increased with the dose.
Treatment with GC is frequently used in combination with PPIs in China, and the HAP risk associated with this has not been mentioned in previous studies. This phenomenon was confirmed in our study, where 70.7% of GC therapy patients were also prescribed PPIs. In the cohort, after PSM, we found a 40% increased risk of HAP in the PPIs exposed group compared to the unexposed group. Previous studies in this area have reported similar HAP risks. Systematic review and meta-analysis based on RCTs showed that acid-suppressive medication use increased the risk of HAP by 27% (13). Herzig et al. (12) found the OR between PPI use and no acid-suppression medication use was 1.3 (95% CI: 1.1 to 1.4); however, other recent studies did not seem to support these findings. According to a systemic review and meta-analysis published in 2022, antiacid therapy was not a significant predictor factor of HAP (OR: 1.44, 95% CI: 0.79 to 2.62) (27). Strassle et al. (28) conducted a cohort study in a U.S. academic teaching hospital and did not detect a change in non-device-associated pneumonia with the use of PPIs (adjusted HR: 1.16; 95% CI: 0.90 to 1.50). A recent multicenter, parallel-group, blinded trial showed that the mortality at 90 days and the clinically important events, such as pneumonia, occurred similarly between the pantoprazole group and the placebo group among critical adult patients (14). However, the samples in these trials might be insufficient to maintain the test power if we referred to the OR and the incidence of HAP of the above cohort studies. The occurrence of HAP is affected by many aspects, such as the bacterial spectrum, nursing level, and medical habits in different hospitals and wards. These factors have a more direct impact on the risk of HAP, resulting in the underestimation of the risk caused by medication. A large cohort can reduce these potential biases because it balances them out between the 2 groups. In retrospective cohort studies, differences in the baseline between groups are mainly due to differences in the propensity of patients in the 2 groups to receive exposure factors. We addressed this problem by using the PSM method. In the matched cohort, all the observed covariables that might have affected exposure allocation or affect outcome were balanced (no statistical difference). In previous studies on PPIs and HAP risk, GC therapy was generally included as a covariable in the statistical model. However, GC therapies are different from other drugs in that they are widely used in clinical practice, which may lead to differences in various aspects in the medication group compared with the non-medication patients. It is difficult to balance the potential biases caused by GC therapy because many of the differences cannot be observed. If the model is adjusted only by considering GC therapy as a dichotomous covariate, a large bias may be introduced. Therefore, we limited the study to cases treated with GC therapy. In this way, when the cohort is large enough, the bias associated with GC therapy is sufficiently balanced across the groups.
Even though PPI prophylaxis might decrease the risk of gastrointestinal bleeding caused by GC therapy in critical patients, the protective effect associated with GC therapy had not yet been validated and the incidence of gastrointestinal bleeding was very low in the patients who did not have other risk factors. A recent study found that PPIs use was associated with greater mortality for adult inpatients with coronavirus disease of 2019 (29). Therefore, the phenomena of PPI overuse in patients combined with GC therapy should pique our attention. Given the large population attributable risk percentage in our study and the high known mortality of HAP during hospitalization (30), it is necessary to carefully evaluate the risk of HAP before using PPIs.
Our study has shown that the HAP risk was associated with the duration of PPIs after adjusting the numerous covariates including LOS. The NNHs to trigger a HAP case was only 82 when a more than 7-day dose of PPIs was prescribed after GC therapy. Similar to our study, Al Sulaiman et al. (31) found that compared with esomeprazole 20 mg, esomeprazole 40 mg was statistically associated with pneumonia (not excluding the ventilator HAP) in critically ill patients. However, previous studies focusing on the association of PPIs and community-acquired pneumonia have reported findings contrary to ours. Those studies found that the risk of community-acquired pneumonia decreased when PPI duration increased (32-35). The authors of those studies speculated that there was an unknown pharmacological mechanism other than acid-suppression. We concluded that this phenomenon was not triggered by the difference between HAP and community-acquired pneumonia after we reviewed those relevant studies in detail. In the above studies, prolonged use meant prescription for more than 2 months, while the timeframe of our study was limited to about 1 week due to the short length of hospitalization. We assumed that the incidence of infection might increase sharply when the duration of PPIs was extended. Nevertheless, our bodies might balance this pharmacological effect during a longer course of PPIs treatment. The HAP incidence-time curve might reach a peak after 1–4 weeks of PPIs treatment and then decline. Moreover, there was a high probability of overtreatment because existing guidelines recommended few withdrawal criteria of PPIs. If someone required PPIs combined with GC therapy, a 3–7 DDDs dose of PPIs would be appropriate for those with a high risk of gastrointestinal bleeding (36,37); it would be better for inpatients to prescribe less than 2 DDDs for the patients with other indications (38).
The study population was a strength of our study because the included patients were from a large nationwide cohort in China. The incidence of HAP during hospitalization was relatively low and many admissions were required to maintain the power of the test. Our study first enrolled more than 100,000 admissions in China to investigate the adverse drug effect of PPIs during hospitalization. Moreover, the study population was distributed in 20 large hospitals and more than 10 provinces in China. These factors improved the generalizability of our study. A retrospective cohort study was more likely to have a bias between the exposed group and the other group because the exposure group clearly had a higher risk of digestive disease. Normally, it is hard to rule out whether these underlying diseases were contributing factors to HAP. To address this problem in our study, a multiple logistic regression and PSM were performed to adjust the confounding bias. The 2 methods produced similar results. Furthermore, there is no clear guidance for when to stop taking PPIs during a period of hospitalization. The dose-effect relationship between PPIs and HAP in this study might help provide some clues.
Inevitably, there were some limitations to our study. First, we used ICD-10 and ATC code to classify the disease and medication, and misclassification was inevitable, even with a manual check. Previous studies based on this database showed that the misclassification was tolerable (18,19). Second, we could not distinguish the time relationship between PPIs treatment and HAP occurrence because the information on the exact time of HAP occurrence were lacking. The use of some PPIs might not be associated with HAP, given that the medication use was later than the occurrence of disease. This led to the actual risk being lower than our estimated risk. We addressed this limitation with a sensitivity analysis in which all patients receiving initial PPIs more than 48 hours after hospitalization were reclassified as having not received PPIs. According to the original definition of HAP, all HAP occurred during a hospital stay 48 hours after admission. Therefore, all exposures occurred before the outcome in the second sensitivity analysis. Although the OR for the risk decreased from 1.4 to 1.3, it was still statistically significant. Due to accessibility, some important covariates were not included in the study, such as daily GC therapy dose, enteral nutrition, hospital transfer data, living habits, and socioeconomic status, all of which might influence PPIs use and HAP occurrence. Therefore, it is necessary to conduct a prospective cohort study with more complete inclusion data and better control of confounding variables in the future.
Conclusions
In summary, our study found that PPIs prophylaxis increased the risk of HAP in hospitalized patients treated with GC therapy, and the percentage of population-attributable risk of PPIs associated with HAP was high. Careful assessment of HAP risk is necessary before prescribing PPIs in these patients. The risk of HAP increased with the cumulative dose of PPIs. However, it is relatively safe to administer PPIs at doses of 2 days or less. Shortening the duration of PPIs use may be an effective way to reduce the risk of HAP in patients treated with GC therapy.
Acknowledgments
We appreciate the AME editing service for providing language editing for this article.
Funding: This work was supported by Shanghai Municipal Health Commission scientific research project (grant number 201940028).
Footnote
Reporting Checklist: The authors have completed the STROBE Reporting Checklist. Available at https://jtd.amegroups.com/article/view/10.21037/jtd-21-1886/rc
Data Sharing Statement: Available at https://jtd.amegroups.com/article/view/10.21037/jtd-21-1886/dss
Peer Review File: Available at https://jtd.amegroups.com/article/view/10.21037/jtd-21-1886/prf
Conflicts of Interest: Both authors have completed the ICMJE uniform disclosure form (available at https://jtd.amegroups.com/article/view/10.21037/jtd-21-1886/coif). Both authors report that the study was funded by Shanghai Municipal Health Commission scientific research project (grant number 201940028). Both authors have no other conflicts of interest to declare.
Ethical Statement:
Open Access Statement: This is an Open Access article distributed in accordance with the Creative Commons Attribution-NonCommercial-NoDerivs 4.0 International License (CC BY-NC-ND 4.0), which permits the non-commercial replication and distribution of the article with the strict proviso that no changes or edits are made and the original work is properly cited (including links to both the formal publication through the relevant DOI and the license). See: https://creativecommons.org/licenses/by-nc-nd/4.0/.
References
- Baker D, Quinn B. Hospital Acquired Pneumonia Prevention Initiative-2: Incidence of nonventilator hospital-acquired pneumonia in the United States. Am J Infect Control 2018;46:2-7. [Crossref] [PubMed]
- Gonçalves-Pereira J, Mergulhão P, Nunes B, et al. Incidence and impact of hospital-acquired pneumonia: a Portuguese nationwide four-year study. J Hosp Infect 2021;112:1-5. [Crossref] [PubMed]
- Yin Y, Zhao C, Li H, et al. Clinical and microbiological characteristics of adults with hospital-acquired pneumonia: a 10-year prospective observational study in China. Eur J Clin Microbiol Infect Dis 2021;40:683-90. [Crossref] [PubMed]
- Feng DY, Zhou YQ, Zou XL, et al. Differences in microbial etiology between hospital-acquired pneumonia and ventilator-associated pneumonia: a single-center retrospective study in Guang Zhou. Infect Drug Resist 2019;12:993-1000. [Crossref] [PubMed]
- Eusebi LH, Rabitti S, Artesiani ML, et al. Proton pump inhibitors: Risks of long-term use. J Gastroenterol Hepatol 2017;32:1295-302. [Crossref] [PubMed]
- Igaz I, Simonyi G, Balogh S, et al. Adverse effects of long-term proton-pump inhibitor therapy on adults. Orv Hetil 2018;159:735-40. [Crossref] [PubMed]
- Liapikou A, Cilloniz C, Torres A. Drugs that increase the risk of community-acquired pneumonia: a narrative review. Expert Opin Drug Saf 2018;17:991-1003. [Crossref] [PubMed]
- Wilhelm SM, Rjater RG, Kale-Pradhan PB. Perils and pitfalls of long-term effects of proton pump inhibitors. Expert Rev Clin Pharmacol 2013;6:443-51. [Crossref] [PubMed]
- Marchina S, Doros G, Modak J, et al. Acid-suppressive medications and risk of pneumonia in acute stroke patients: A systematic review and meta-analysis. J Neurol Sci 2019;400:122-8. [Crossref] [PubMed]
- Huang M, Kuang L, Han M, et al. Efficacy and safety of stress ulcer prophylaxis in septic patients: a retrospective cohort study based on large database. Zhonghua Wei Zhong Bing Ji Jiu Yi Xue 2021;33:641-7. [PubMed]
- Huang M, Han M, Han W, et al. Proton pump inhibitors versus histamine-2 receptor blockers for stress ulcer prophylaxis in patients with sepsis: a retrospective cohort study. J Int Med Res 2021;49:3000605211025130. [Crossref] [PubMed]
- Herzig SJ, Howell MD, Ngo LH, et al. Acid-suppressive medication use and the risk for hospital-acquired pneumonia. JAMA 2009;301:2120-8. [Crossref] [PubMed]
- Eom CS, Jeon CY, Lim JW, et al. Use of acid-suppressive drugs and risk of pneumonia: a systematic review and meta-analysis. CMAJ 2011;183:310-9. [Crossref] [PubMed]
- Krag M, Marker S, Perner A, et al. Pantoprazole in Patients at Risk for Gastrointestinal Bleeding in the ICU. N Engl J Med 2018;379:2199-208. [Crossref] [PubMed]
- Hu J, Su Y, Du L, et al. Investigation and analysis of prophylactic application of PPIs in AECOPD patients after glucocorticoid use in a hospital. China Pharmacy 2017;28:161-4.
- Mao X, Yang Z. Medical big data analysis of the clinical off-label use of proton pump inhibitors. Journal of Pharmaceutical Practice 2020;38:184-8.
- Ahrens D, Chenot JF, Behrens G, et al. Appropriateness of treatment recommendations for PPI in hospital discharge letters. Eur J Clin Pharmacol 2010;66:1265-71. [Crossref] [PubMed]
- Nie XM, Li YS, Yang ZW, et al. Initial empiric antibiotic therapy for community-acquired pneumonia in Chinese hospitals. Clin Microbiol Infect 2018;24:658.e1-6. [Crossref] [PubMed]
- Mao X, Yang Z. Current usage status of somatostatin and its analogs and trypsin inhibitors: a real-world study of 34,654 Chinese adult patients with acute pancreatitis. Ann Palliat Med 2021;10:1325-35. [Crossref] [PubMed]
- WHO. ATC/DDD Index 2020 2020 [updated 2019-12-16; cited 2020 January 04]. Available online: http://www.whocc.no/atc_ddd_index
- Charlson ME, Pompei P, Ales KL, et al. A new method of classifying prognostic comorbidity in longitudinal studies: development and validation. J Chronic Dis 1987;40:373-83. [Crossref] [PubMed]
- Quan H, Sundararajan V, Halfon P, et al. Coding algorithms for defining comorbidities in ICD-9-CM and ICD-10 administrative data. Med Care 2005;43:1130-9. [Crossref] [PubMed]
- Reducing bias in a propensity score matched-pair sample using greedy matching techniques: Proceedings of the Twenty-Sixth Annual SAS (Users Group International Conference); 2009 [cited 2020 January 07]. Available online: http://www2.sas.com/proceedings/sugi26/p214-26.pdf
- Herzig SJ, Vaughn BP, Howell MD, et al. Acid-suppressive medication use and the risk for nosocomial gastrointestinal tract bleeding. Arch Intern Med 2011;171:991-7. [Crossref] [PubMed]
- Austin PC. An Introduction to Propensity Score Methods for Reducing the Effects of Confounding in Observational Studies. Multivariate Behav Res 2011;46:399-424. [Crossref] [PubMed]
- Leisman DE. Ten Pearls and Pitfalls of Propensity Scores in Critical Care Research: A Guide for Clinicians and Researchers. Crit Care Med 2019;47:176-85. [Crossref] [PubMed]
- Lukasewicz Ferreira SA, Hubner Dalmora C, Anziliero F, et al. Factors predicting non-ventilated hospital-acquired pneumonia: systematic review and meta-analysis. J Hosp Infect 2022;119:64-76. [Crossref] [PubMed]
- Strassle PD, Sickbert-Bennett EE, Klompas M, et al. Incidence and risk factors of non-device-associated pneumonia in an acute-care hospital. Infect Control Hosp Epidemiol 2020;41:73-9. [Crossref] [PubMed]
- Oddy C, McCaul J, Keeling P, et al. Pharmacological Predictors of Morbidity and Mortality in COVID-19. J Clin Pharmacol 2021;61:1286-300. [Crossref] [PubMed]
- Kollef MH, Shorr A, Tabak YP, et al. Epidemiology and outcomes of health-care-associated pneumonia: results from a large US database of culture-positive pneumonia. Chest 2005;128:3854-62. [Crossref] [PubMed]
- Al Sulaiman K, Al Aamer K, Al Harthi A, et al. Comparison between esomeprazole 20 mg vs. 40 mg as stress ulcer prophylaxis (SUP) in critically ill patients: A retrospective cohort study. Pharmacol Res Perspect 2020;8:e00624. [Crossref] [PubMed]
- Gulmez SE, Holm A, Frederiksen H, et al. Use of proton pump inhibitors and the risk of community-acquired pneumonia: a population-based case-control study. Arch Intern Med 2007;167:950-5. [Crossref] [PubMed]
- Laheij RJ, Sturkenboom MC, Hassing RJ, et al. Risk of community-acquired pneumonia and use of gastric acid-suppressive drugs. JAMA 2004;292:1955-60. [Crossref] [PubMed]
- Sarkar M, Hennessy S, Yang YX. Proton-pump inhibitor use and the risk for community-acquired pneumonia. Ann Intern Med 2008;149:391-8. [Crossref] [PubMed]
- Moayyedi P, Eikelboom JW, Bosch J, et al. Safety of Proton Pump Inhibitors Based on a Large, Multi-Year, Randomized Trial of Patients Receiving Rivaroxaban or Aspirin. Gastroenterology 2019;157:682-691.e2. [Crossref] [PubMed]
- Sung JJ, Barkun A, Kuipers EJ, et al. Intravenous esomeprazole for prevention of recurrent peptic ulcer bleeding: a randomized trial. Ann Intern Med 2009;150:455-64. [Crossref] [PubMed]
- Bai Y, Chen DF, Wang RQ, et al. Intravenous Esomeprazole for Prevention of Peptic Ulcer Rebleeding: A Randomized Trial in Chinese Patients. Adv Ther 2015;32:1160-76. [Crossref] [PubMed]
- Savarino V, Marabotto E, Furnari M, et al. Latest insights into the hot question of proton pump inhibitor safety - a narrative review. Dig Liver Dis 2020;52:842-52. [Crossref] [PubMed]