Role of TRPA1/TRPV1 in acute ozone exposure induced murine model of airway inflammation and bronchial hyperresponsiveness
Introduction
Ozone is a secondary atmospheric pollutant that acts as a strong oxidant (1). Exposure to high concentrations of ozone induces oxidative stress and damages the airways, resulting in airway diseases such as asthma and chronic obstructive pulmonary disease (COPD) (2). Inflammation and tissue injury are crucial mechanisms underlying ozone-induced respiratory diseases. When ozone is inhaled and dissolves into the epithelial cell lining fluid, reactive oxygen species (ROS) are generated and lead to the release of cytokines by activation of underlying airway epithelial cells (AECs) (3). These trigger airway inflammation and bronchial hyperresponsiveness (BHR) in both humans and in murine models of airway disease (4).
Mitochondria are double-membrane organelles present in almost all eukaryotic organisms (5). Mitochondria play an essential role in energy metabolism, synthesizing a large proportion of the adenosine triphosphate (ATP) required by the cell via the mitochondrial respiratory chain (MRC) complex V (6). In addition to ATP synthesis, mitochondria also perform critical roles in immune and inflammatory responses, cell survival and proliferation, and the generation and response to cellular metabolites. Mitochondrial fusion/fission and mitophagy are the main mechanisms of mitochondrial quality control, which play a crucial role in the pathological mechanism of respiratory diseases (7). Optic atrophy 1 (OPA1) and mitofusin 1 and 2 (MFN1 and MFN2) are key fusion-regulated proteins, while dynamin-related protein (DRP1), mitochondrial fission 1 (FIS1) and mitochondrial fission factor (MFF) are involved in mitochondrial fission (8). The major pathway of mitophagy is mediated by PTEN-induced putative kinase 1 (PINK1) and Parkinson protein 2 E3 ubiquitin protein ligase (PARK2) (9). The specific process includes the stabilization of PINK1 on the mitochondrial outer membrane, recruitment and phosphorylation of E3-ubiquitin ligase PARK2, ubiquitination of the substrates (such as voltage-dependent anion channel-1 (VDAC1), MFN 1/2) by PARK2, and the binding of ubiquitinated substrates and microtubule-associated protein light chain 3 (LC-3) on autophagosomes through Sequestosome 1 (SQSTM1)/p62 (9). Mitochondria produce ROS to maintain cellular homeostasis under normal physiological conditions, but excess generation of ROS by dysregulated mitochondria induces or aggravates respiratory diseases (10).
Transient receptor potential (TRP) channels are activated by oxidative stress, inflammation and hypoxia and may be involved in the pathogenesis of airway diseases (11). TRP ankyrin 1 (TRPA1) and TRP vanilloid 1 (TRPV1) are predominantly expressed in sensory nerves and are also present in airway epithelial and smooth muscle cells within the respiratory tract (12). TRPA1 is the major airway irritant sensing receptor with a unique feature of being activated by both hypoxia and hyperoxia (13), while TRPV1 is a polymodal transducer detecting low pH, high temperature, anandamide, bradykinin and other endogenous inflammatory mediators (14). Acute ozone exposure could directly stimulate TRPA1 channel and then activate bronchopulmonary C-fibers, which is an important trigger of airway inflammation and BHR (15,16). It has been reported that ozone selectively stimulates TRPA1 not TRPV1 (16). Our previous studies have indicated that both TRPA1 and TRPV1 induce inflammatory signaling by cigarette smoke extract (CSE) in AECs in vitro and by fine particulate matter (PM2.5) in vivo (17,18).
In the present study, we hypothesized that TRPA1 and TRPV1 directly or indirectly sense ozone exposure in the airways. We determined ozone induced airway inflammation and BHR model by separately administering A967079, a TRPA1 antagonist, and AMG9810, a TRPV1 antagonist, in mice before or after acute ozone exposure. We present the following article in accordance with the ARRIVE reporting checklist (available at https://jtd.amegroups.com/article/view/10.21037/jtd-22-315/rc).
Methods
Ozone exposure and inhibitor administration
Ethics approval for this study was obtained from the ethics committee of Shanghai Chest Hospital for animal experimentation (No. KS1875), in compliance with national or institutional guidelines for the care and use of animals (Shanghai, China; SYXK2018-0016). Male specific-pathogen-free (SPF) C57BL/6 mice (8–10-week-old), weighing 23–24 g, were purchased from Shanghai SLAK Laboratory Animal Corp. Ltd. (Shanghai, China) and housed in specific-pathogen-free (SPF) conditions. Mice were exposed to ozone at a concentration of 2.5 ppm for 3 h produced by a generator (Model 300, AB Aqua Medick GmbH, Bissendorf, Germany) in a whole-body exposure chamber (65 cm × 45 cm × 37 cm) maintained. Control mice were exposed to filtered air. Mice were administered intraperitoneally with vehicle (phosphate buffered saline, PBS), A967079 (30 mg/kg, Abcam, USA) or AMG9810 (30 mg/kg, Abcam, USA) 1 hour before or after ozone exposure (n=8 per group, total n=64). The doses of TRPA1 and TRPV1 antagonists were chosen based on our previous publication (18). The individual mouse was considered as an experimental unit in the study.
BHR
BHR was measured 24 h after ozone exposure. After anesthesia with injection of 1% pentobarbital and tracheotomy, mice were transferred to a whole-body plethysmograph to measure resistance and compliance (EMMS, Hants, UK). Mice were challenged with a range of doses of aerosolized acetylcholine (ACh) (Sigma-Aldrich, St Louis, MO, USA) (0, 8, 16, 32, 64, 128 and 256 mg/mL) for 3 min. Lung resistance (RL) was recorded and presented as the percentage change from baseline RL of nebulizing PBS. The concentration of ACh required to increase lung resistance by 100% from baseline was calculated (PC100), and –logPC100 was taken as a measure of bronchial responsiveness.
Bronchoalveolar lavage (BAL) fluid and cell counting
Following terminal anesthesia, a total of 1.5 mL of PBS was injected through the endotracheal tube and then collected as BAL fluid. After centrifuging at 4 ℃, 1,000 rpm, for 10 min, the BAL fluid supernatant was stored at −80 ℃, whilst the cell pellet was re-suspended in PBS for cell counting. Total cell and differential cell counts (including macrophages, neutrophils, lymphocytes, eosinophils, and basophils) were determined using a hematology analyzer for common laboratory animals (Mindray, Shenzhen, Guangdong, China).
Oxidant levels in the lung
Approximately 40 mg of mice lung tissue was weighed before homogenizing in RIPA Lysis Buffer (Beyotime, Haimen, Jiangsu, China). Protein concentrations were measured using a BCA assay kit (Beyotime). The levels of malondialdehyde (MDA) in lung tissue homogenates were measured by lipid peroxidation MDA assay kits (Beyotime) to calculate MDA content per unit of protein weight according to the manufacturer’s instructions. The reduced glutathione (GSH) and oxidized glutathione (GSSG) levels in lung tissues were examined by a GSH and GSSG assay kit (Beyotime) according to the manufacturer’s instructions.
Real-time (RT) qPCR analysis
The mRNA levels of cytokines including Substance P (SP), Keratinocyte-Derived Chemokine (KC), interleukin-18 (IL-18) and chemokine (C-X-C motif) ligand 8 (CXCL8) were measured by RT-qPCR. Total RNA was extracted by TRIzol reagent (Invitrogen, Carlsbad, CA, USA) and the concentration of RNA was determined using an ultraviolet spectrophotometer. cDNA was reverse transcribed from 1µg RNA with HiScript II qRT SuperMix II (Vazyme, Nanjing, Jiangsu, China) according to the manufacturer’s instructions. Specific genes were PCR-amplified and quantified using the ChamQ Universal SYBR qPCR Master Mix (Vazyme). RT-qPCR was conducted in an ABI ViiATM 7 System (Applied Biosystems, Foster City, CA, USA). The forward and reverse primers of cytokines and β-actin are listed in Table 1.
Table 1
Gene | Forward | Reverse |
---|---|---|
SP | AAACACACAGACTTGAGCATTC | TTAGGGTCTAGGACTAGCTTGT |
KC | ATGGCTGGGATTCACCTCAAGAA | AGTGTGGCTATGACTTCGGTTTG |
CXCL8 | AACTGAGAGTGATTGAGAGTGG | ATGAATTCTCAGCCCTCTTCAA |
IL-18 | GCTGAAGATGATGAAAACCTGG | CAAATAGAGGCCGATTTCCTTG |
β-actin | GGCCAACCGCGAGAAGATGAC | GGATAGCACAGCCTGGATAGCAAC |
SP, Substance P; KC, Keratinocyte-Derived Chemokine; CXCL8, chemokine (C-X-C motif) ligand 8; IL-18, interleukin-18.
Western blot analysis
Total proteins were extracted from lung tissues of mice using a RIPA Lysis Buffer (Beyotime) and protein concentrations were determined by a BCA assay kit (Beyotime). 30 µg protein per lane was separated using 8–10% SDS PAGE, transferred to a 0.45-µm PVDF membrane, blocked with 5% non-fat milk, and incubated with primary antibodies against DRP1, MFF, OPA1, MFN2 (Cell Signaling Technology, Danvers, MA, USA), PINK1, PARK2, SQSTM1/p62 (Abcam, Cambridge, MA, USA), TRPA1 (Novus Biologicals, Littleton, CO, USA), TRPV1 (Immunoway, Newark, DE, USA ) and GAPDH (Proteintech, Wuhan, Hubei, China) overnight at 4 ℃. Membranes were incubated with the secondary antibodies, HRP-conjugated goat anti-rabbit IgG or HRP-conjugated goat anti-mouse IgG (all 1:3,000, Cell Signaling Technology) for 2 h at room temperature, and the bands were visualized by chemiluminescence.
Activities of MRC
Mitochondrial function was assessed using an MRC complex III and V Activity Assay Kit (Solarbio Life Sciences, Beijing, China). Briefly, lung tissues were homogenized to detect MRC complex activity according to the manufacturer’s instructions. The protein concentrations of the lung tissue homogenates were measured to calculate the expression of the enzyme activity as U/mg protein.
Statistical analysis
Statistical analysis was described as the mean ± SEM by using GraphPad InStat software (Prism 8, GraphPad Software, Inc.). Two-way ANOVA was performed to compare the percentage increase in RL of different groups. One-way ANOVA with Bonferroni’s post hoc test (for normally distributed data) or Dunnett’s T3 post hoc test (for non-normally distributed data) was used to compare multiple groups. P<0.05 was considered significant.
Results
A967079 and AMG9810 inhibited BHR
There were significant increases in RL following ozone exposure for 3 h compared to air exposure at 128 and 256 mg/mL of ACh in mice within the group to be pre-ozone treated with inhibitors (P<0.05 and P<0.001, Figure 1A) and in mice within the group to be post-ozone treated with inhibitors (P<0.01 and P<0.01, Figure 1B). This was associated with decreased –logPC100 indicating an increase in airway responsiveness to ACh challenge and increased lung resistance in ozone-exposed mice compared to air-exposed mice (Figure 1C,1D for animals in the pre- and post-ozone drug groups respectively).
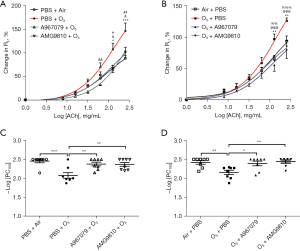
Pretreatment with A967079 inhibited RL at 64, 128 and 256 mg/mL of ACh (P<0.01, P<0.05, and P<0.05, respectively, Figure 1A) and AMG9810 inhibited ozone-induced RL at 128 and 256 mg/mL ACh (P<0.05, and P<0.01, Figure 1A). Pretreatment with A967079 and AMG9810 also inhibited ozone-induced bronchial responsiveness in terms of –logPC100 to a similar extent (Figure 1C). Therapeutic treatment with A967079 and AMG9810 1h after ozone exposure also reversed RL at 128 and 256 mg/L of ACh (P<0.001 and P<0.001 for A967079, and P<0.01 and P<0.001 for AMG9810, Figure 1B). Therapeutic treatment with A967079 and AMG9810 also reversed the ozone-induced bronchial responsiveness in terms of –logPC100 (Figure 1D).
A967079 and AMG9810 reduced BAL cells and lung cytokine levels
Ozone exposure resulted in significant increases in total BAL cell counts and in mononuclear cells (macrophages and lymphocytes), neutrophils, eosinophils and basophils compared with air-exposed mice in the group to be pre-treated with inhibitors (all P<0.001, Figure 2A). Ozone exposure enhanced the mRNA expression of SP, KC, CXCL8 and IL-18 compared to air-exposed mice in the group to be pre-treated with drugs (P<0.05, P<0.001, P<0.05 and P<0.05, respectively, Figure 2B-2E). Similar results were seen in ozone-exposed mice in the group treated with inhibitors 1 h following ozone exposure (Figure 2F). Ozone induction of SP, KC, CXCL8 and IL-18 mRNA was similar but with a greater level of significance (all P<0.001, Figure 2G-2J).
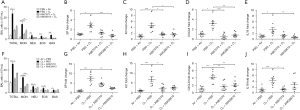
Pretreatment with A967079 reduced the number of total cells and mononuclear cells (P<0.05, P<0.001 respectively, Figure 2A), and inhibited ozone-induced mRNA levels of KC and CXCL8 (P<0.05, P<0.001 respectively, Figure 2C,2D), whilst pretreatment with AMG9810 reduced mononuclear cells (P<0.01, Figure 2A), and inhibited mRNA levels of SP, KC, CXCL8 and IL18 (P<0.001, P<0.05, P<0.01 and P<0.01 respectively, Figure 2B-2E). Both A967079 and AMG9810 showed a trend towards decreasing neutrophil, eosinophil and basophil cell counts but this did not reach significance (Figure 2A).
Therapeutic treatment with A967079 reduced the number of total cells, mononuclear cells, neutrophils and eosinophils (P<0.05, P<0.001, P<0.01 and P<0.01 respectively, Figure 2F) and attenuated ozone-enhanced expression of KC, CXCL8 and IL-18 mRNA (P<0.05, P<0.01 and P<0.05 respectively, Figure 2H-2J). In contrast, therapeutic treatment with AMG9810 reduced ozone-induced mononuclear cell counts only (P<0.001, Figure 2F), and attenuated ozone-stimulated mRNA levels of SP and CXCL8 (P<0.01 and P<0.01, respectively, Figure 2G,2I).
A967079 and AMG9810 ameliorated lung oxidative stress
Ozone exposure increased MDA levels (P<0.001, Figure 3A) and reduced GSH/GSSG levels (P<0.05, Figure 3B) in lung tissue in the pre-treatment group compared with air-exposed control mice. Ozone also increased MDA levels (P<0.001, Figure 3C) and reduced GSH/GSSG levels (P<0.05, Figure 3D) in lung tissue in the therapeutic treatment group compared with air-exposed control mice.
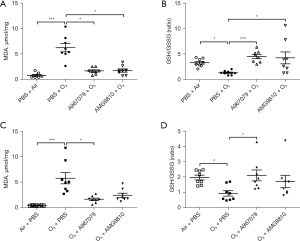
Preventive treatment with A967079 ameliorated MDA levels similar to levels seen in air-exposed animals in the pre-treatment (P<0.05, Figure 3A) and therapeutic treatment (P<0.05, Figure 3C) groups. In contrast, pre-treatment (P<0.05, Figure 3A), but not therapeutic treatment, with AMG9810 ameliorated the MDA levels (Figure 3C).
Similarly, preventive treatment with A967079 enhanced GSH/GSSG levels back to levels seen in air-exposed animals in the pre-treatment (P<0.001, Figure 3B) and therapeutic treatment (P<0.05, Figure 3D) groups. In contrast, only pre-treatment, but not therapeutic treatment, with AMG9810 restored ozone-attenuated GSH/GSSG levels (P<0.05, Figure 3B).
A967079 and AMG9810 attenuated lung TRPA1/ TRPV1 expression
TRPA1 and TRPV1 were expressed in the lungs of control air-exposed mice. In the pre-treatment group, ozone-exposed mice demonstrated increased protein levels of TRPA1 (P<0.01, Figure 4A) and TRPV1 (P<0.001, Figure 4B) in lung tissue. Similarly, ozone enhanced TRPA1 (P<0.001, Figure 4C) and TRPV1 (P<0.05, Figure 4D) in the therapeutic treatment group.
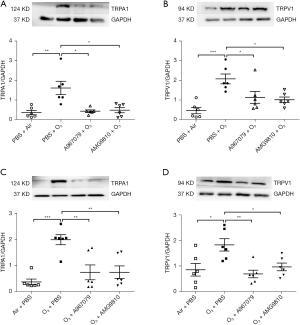
Preventive treatment with A967079 and AMG9810 attenuated (all P<0.05) ozone-enhanced protein levels of TRPA1 (Figure 4A) and TRPV1 (Figure 4B). Therapeutic treatment with A967079 or AMG9810 also attenuated the ozone-elevated protein levels of TRPA1 (both P<0.01, Figure 4C) and TRPV1 (P<0.01 and P<0.05 respectively, Figure 4D).
A967079 and AMG9810 regulated lung mitochondrial dynamics- and mitophagy-related protein expression
In the pre-treatment group, ozone elevated the protein expression of DRP1 and MFF, and decreased that of OPA1 and of MFN2 (P<0.01, P<0.001, P<0.05 and P<0.01, respectively, Figure 5A-5D). Exposure to ozone decreased the protein expression of PINK1 and increased the protein expression of PARK2 and SQSTM1/p62 (P<0.05, P<0.01 and P<0.01, respectively, Figure 5E-5G) compared with air-exposed control mice.
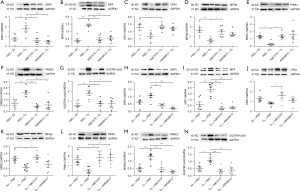
In the therapeutic treatment group, ozone elevated the protein expression of DRP1 and MFF, and decreased that of OPA1 and of MFN2 (P= ns, P<0.001, P= ns and P<0.05, respectively, Figure 5H-5K). Similar results were seen in the therapeutic group with ozone decreasing PINK1 protein expression whilst increasing PARK2 and SQSTM1/p62 protein expression (P<0.05, P<0.001 and P<0.05, respectively, Figure 5L-5N) compared with air-exposed control mice.
Preventive treatment with A967079 inhibited ozone-induction of DRP1 and MFF proteins, and enhanced ozone-suppression OPA1 and MFN2 protein expression (P<0.01, P<0.001, P<0.05 and P<0.05, respectively, Figure 5A-5D). Preventive treatment with AMG9810 suppressed the ozone induction of DRP1 and MFF proteins (both P<0.001, Figure 5A,5B). AMG9810 enhanced ozone-induced suppression of OPA1 and MFN2 (Figure 5C,5D) but these effects did not reach significance. Preventive treatment with A967079 up-regulated the ozone-reduced PINK1 expression and suppressed the ozone-enhanced levels of PARK2 and SQSTM1/p62 (P<0.05, P<0.001 and P<0.05, respectively, Figure 5E-5G) proteins. Similar results were seen with AMG9810, although these did not reach significance for PINK1 (P=ns, P<0.01, and P<0.05, respectively, Figure 5E-5G).
Therapeutic treatment with A967079 reversed ozone induction of DRP1 and MFF, and increased ozone-suppressed OPA1 and MFN2 (P<0.01, P<0.001, P<0.05 and P<0.05, respectively, Figure 5H-5K). Whilst therapeutic treatment with AMG9810 reversed ozone induction of DRP1 and MFF (both P<0.001, Figure 5H,5I), AMG9810 did not significantly elevate the repressed levels of OPA1 and MFN2 (Figure 5J,5K). Therapeutic treatment with both A967079 and AMG9810 enhanced ozone-attenuated levels of PINK1 expression (both P<0.01, Figure 5L) and down-regulated ozone-enhanced expression of PARK2 (both P<0.001, Figure 5M) and SQSTM1/p62 (P<0.001 and P<0.01, respectively, Figure 5N).
A967079 and AMG9810 regulated lung activity of MRC
The MRC complex III and V activities were set to 100% in the lungs of air-exposed animals (Figure 6). Ozone exposure caused a significant suppression of MRC complex III and MRC complex V (P<0.05 and P<0.001, respectively, Figure 6A,6B) compared to air controls in the pre-treatment group. Similar results were observed in the therapeutic treatment group for both MRC complex III and MRC complex V (P<0.01 and P<0.001, respectively, Figure 6C,6D).
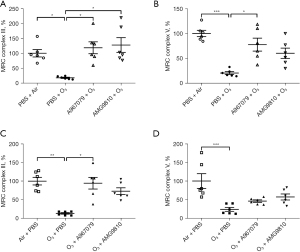
Pretreatment with A967079 increased the ozone-suppressed activity of MRC complexes III and V (both P<0.05, Figure 6A,6B) while pretreatment with AMG9810 increased the activity of MRC complex III (P<0.05, Figure 6A) but this did not reach significance for MRC complex V (Figure 6B). In the therapeutic treatment groups, although both A967079 and AMG9810 raised ozone-suppressed MRC complex III and MRC complex V activities (Figure 6C,6D), this was only significant for A967079 effects on MRC complex III activity (P<0.05, Figure 6C).
Discussion
In the present study, we demonstrated that acute ozone exposure enhanced BHR (increased lung resistance), airway inflammation (BAL cell counts and inflammatory cytokine mRNA) and oxidative stress (elevated MDA levels and decreased GSH/GSSG levels). This was associated with mitochondrial dysfunction as represented by an abnormal expression of mitochondrial fission/fusion-related protein and mitophagy-related protein, and impaired activities of MRC complexes. These processes were partially or fully prevented or reversed by treatment with the TRPA1 inhibitor A967079 or the TRPV1 inhibitor AMG9810. We conclude that the TRPA1/TRPV1 pathways play a significant contributing factor to acute ozone-induced lung injury in mice.
Previous studies have confirmed that acute ozone inhalation is an important trigger of airway inflammation and BHR (15). BHR is defined by increased airway narrowing, which is caused by enhanced bronchial smooth muscle (BSM) contraction, neutrophilic inflammation and increased inflammatory cytokines (19,20). BHR is a characterized feature of airway diseases such as asthma and COPD, and acute exposure to ozone in mice has been used as a model of BHR (21). Numerous studies have detected the relationship between airway inflammation and BHR particularly with neutrophilic inflammation (21). In our acute ozone exposure model, the increased RL was inhibited by both A967079 and AMG9810. These findings indicated that ozone exposure may induce BHR in mice by activating the TRPA1/TRPV1 pathway.
A series of cytokines and chemokines have been implicated in ozone-induced BHR and airway inflammation. SP increases under inflammatory conditions, activates eosinophils, and mediates neural plasticity and hyperreactivity (22). Previous studies have demonstrated that interleukin-1β (IL-1β) increased the release of SP in airway neurons mediated by nerve growth factor (NGF) after ozone exposure, which can stimulate sensory nerves and trigger airway reflexes (23). CXCL1 (KC) and CXCL8 (IL-8) are both chemokines for neutrophil migration, which were induced to drive neutrophil recruitment during ozone exposure (24). IL-18 is a member of the IL-1 family particularly related to IL-1β (25). Ozone may induce neutrophilic airway inflammation by activating the inflammasome to generate IL-1β and IL-18 (26). In the present study, ozone exposure increased the numbers of total cells, mononuclear cells, neutrophils, eosinophils and basophils and enhanced the levels of SP, KC, CXCL8 and IL-18 in lung tissue. Preventive treatment with A967079, but not AMG9810, inhibited the total cell counts in BAL fluid and showed a trend towards suppressing differential cell counts, while preventive treatment with A967079 or AMG9810 reduced the mRNA levels of IL-18, which indicate that both TRPA1 and TRPV1 pathway could be related to NOD-like receptor thermal protein domain associated protein 3 (NLRP3)-caspase-1 pathway. Surprisingly, therapeutic treatment with the TRPA1 inhibitor appeared to be more effective than the TRPV1 inhibitor, as therapeutic treatment with A967079 reduced the number of total cells, mononuclear cells, neutrophils and eosinophils in BAL fluid, and attenuated the mRNA levels of KC in lung tissue, while therapeutic treatment with AMG9810 only reduced the number of mononuclear cells in BAL fluid, and attenuated the mRNA levels of SP in lung tissue. The trend towards efficacy in these experiments and the wide variation in some of the responses suggest that additional time-points following ozone exposure may provide greater insights into the effectiveness of TRPA1 and TRPV1 pathways in this model.
Epithelial cells and their lining fluid interact with ozone in the airway to release ROS and cause oxidative stress. Ozone-induced ROS at the epithelial cell membrane can further mediate barrier disruption, inflammation and emphysema (3). Inflammation is closely related to the release of ROS by epithelial cells (27) whilst increased levels of oxidative stress markers and/or antioxidant deficiencies may pose risk factors for respiratory diseases (28). In the present study, we used MDA and GSH as markers of oxidative stress. MDA is the major biomolecule produced by lipid oxidation (29), while the glutathione antioxidant system plays a protective role against the toxic effects of oxidant stress after ozone exposure (30). Consistent with a previous study that showed ozone aggravated oxidative damage by reducing GSH and increasing MDA (31), our study demonstrated that acute ozone exposure enhanced MDA levels and reduced GSH/GSSG levels in lung tissue. Emerging evidence has indicated that TRPA1 and TRPV1 can sense environmental irritants through activation of these channels by ROS (32). A recent study showed that the TRPA1 inhibitor alleviated oxidative stress, as evidenced by the decreasing MDA levels and increasing GSH levels (29). Similarly, both preventive and therapeutic treatment with A967079 decreased MDA levels and increased GSH/GSSG levels. However, in our study only the preventive treatment with AMG9810 decreased MDA levels and increased GSH levels. Therefore, inhibition of TRPA1 channels may be more effective than targeting TRPV1 channels in reducing oxidative stress. Future studies using inhibitors of different structural classes or using genetically modified mice will confirm whether this is the case.
TRPA1 and TRPV1 are co-expressed in nociceptive C fibers innervating the airways (16), and are also found in AECs and airway smooth muscle (ASM) cells, and both channels can be activated by exogenous environmental irritants, such as diesel exhaust particles (DEP), cigarette smoke and particulate matter (PM) (11,33). Moreover, there are significant interactions and synergy between TRPV1 and TRPA1 (11). A recent study has demonstrated that liquiritin inhibited capsaicin- and allyl isothiocyanate-evoked TRPV1 and TRPA1 whole-cell currents, and suppressed LPS-induced increases in TRPV1 and TRPA1 protein expression in the lung tissue. TRPA1 and TRPV1 antagonists HC030031 and capsazepine reduced TRPV1 and TRPA1 expression at both protein and mRNA levels in THP-1 cells (34). Our recent study with PM2.5-induced lung inflammation and BHR in mouse showed that these two antagonists inhibited the protein levels of TRPV1 and TRPA1 in lung tissue (18). The activation of TRPA1 and TRPV1 leads to neurogenic inflammation, while inhibition of neurogenic inflammation by the channel inhibitor may reduce the protein expression of these channels. The present study indicates that the protein expression of TRPA1 and TRPV1 is linked to their activities and is an important mechanism underlying the ozone-induced airway inflammation and BHR.
Mitochondrial dynamics (mainly by continuous fission and fusion) and mitophagy control the structural and functional status of mitochondria (35). The most studied fission- and fusion-related proteins are DRP1 and MFF and MFN2 and OPA1 respectively (36). Mitochondrial dysfunction induced by ROS could lead to the cleavage of fusion proteins and upregulation of fission proteins, which would further generate more mitochondrial ROS, aggravating airway inflammation and lung injury (37). We confirm here a previous report that acute ozone exposure enhanced DRP1 and MFF protein expression and decreased MFN2 and OPA1 proteins (38). We extended this data to show that both A967079 and AMG9810 reduced the ozone-elevated expression of DRP1 and MFF proteins and increased the ozone-suppression of MFN2 and OPA1 proteins. Moreover, the activation of DRP1 triggered by mitochondrial dysfunction induces PINK1-PARK2-mediated mitophagy (39), and enhanced mitophagy induced by cigarette smoke would worsen AECs and mitochondria injury (40). We further examined the protein expression of PINK1, PARK2 and SQSTM1/p62 as indicators of ozone-induced mitophagy in lung tissue. We showed that ozone exposure enhanced mitophagy, which can be reversed by both A967079 and AMG9810. This indicates that TRPA1/TRPV1 pathways may mediate ozone-induced airway inflammation and BHR via regulating mitochondrial dynamics and mitophagy.
The deficiency in mitochondrial dynamics can impair mitochondrial function, leading to a toxic increase of ROS level and a decrease in ATP generation (37). MRC complex III (Ubiquinol-Cytochrome c reductase) is a major site of homeostatic ROS production while Complex V is a multi-subunit complex that synthesizes ATP (41). Our study showed that acute ozone exposure decreased the activities of complex III and Complex V, and that inhibition of TRPA1 channels resulted in a more significant improvement in complex III and V activity than inhibition of TRPV1 channels.
There are several limitations in our study. First, we did not use in vitro model to examine the activity of TRPA1 and TRPV1 channel using calcium flux assay, as an indication of the underlying mechanism. Second, we did not measure the protein levels of cytokines in lung, and we failed to investigate protein localization of TRPA1 and TRPV1 in lung sections. Third, we did not measure the signaling pathways underlying inhibition of TRPA1 and TRPV1 in cells or tissue. Although these have been performed in our previous work (17,18,38), a comprehensive study would provide more mechanistic information, which is under our planning.
Conclusions
In summary, acute ozone exposure elicits profound effects on airway inflammation, BHR, oxidative stress and mitochondrial dysfunction in mice. TRPA1/TRPV1 pathways play a critical role in the mechanisms of ozone induced lung injury. Inhibition of these pathways may be useful in preventing or reversing the onset of airway disease in susceptible subjects.
Acknowledgments
Funding: This work was supported by the project of the National Nature Science Foundation of China (No. 81870031) and Shanghai Jiao Tong University Medical Engineering Cross Research Fund (No. YG2019ZDA24).
Footnote
Provenance and Peer Review: This article was commissioned by the Section Director (Junfeng Zhang) for the series “Air Pollution” published in Journal of Thoracic Disease. The article has undergone external peer review.
Reporting Checklist: The authors have completed the ARRIVE reporting checklist. Available at https://jtd.amegroups.com/article/view/10.21037/jtd-22-315/rc
Data Sharing Statement: Available at https://jtd.amegroups.com/article/view/10.21037/jtd-22-315/dss
Peer Review File: Available at https://jtd.amegroups.com/article/view/10.21037/jtd-22-315/prf
Conflicts of Interest: All authors have completed the ICMJE uniform disclosure form (available at https://jtd.amegroups.com/article/view/10.21037/jtd-22-315/coif). The series “Air Pollution” was commissioned by the editorial office without any funding or sponsorship. The authors have no conflicts of interest to declare.
Ethical Statement: The authors are accountable for all aspects of the work in ensuring that questions related to the accuracy or integrity of any part of the work are appropriately investigated and resolved. Ethics approval for this study was obtained from the ethics committee of Shanghai Chest Hospital for animal experimentation (No. KS1875), in compliance with national or institutional guidelines for the care and use of animals (Shanghai, China; SYXK2018-0016).
Open Access Statement: This is an Open Access article distributed in accordance with the Creative Commons Attribution-NonCommercial-NoDerivs 4.0 International License (CC BY-NC-ND 4.0), which permits the non-commercial replication and distribution of the article with the strict proviso that no changes or edits are made and the original work is properly cited (including links to both the formal publication through the relevant DOI and the license). See: https://creativecommons.org/licenses/by-nc-nd/4.0/.
References
- Mudway IS, Kelly FJ. Ozone and the lung: a sensitive issue. Mol Aspects Med 2000;21:1-48. [Crossref] [PubMed]
- Goodman JE, Zu K, Loftus CT, et al. Short-term ozone exposure and asthma severity: Weight-of-evidence analysis. Environ Res 2018;160:391-7. [Crossref] [PubMed]
- Wiegman CH, Li F, Ryffel B, et al. Oxidative Stress in Ozone-Induced Chronic Lung Inflammation and Emphysema: A Facet of Chronic Obstructive Pulmonary Disease. Front Immunol 2020;11:1957. [Crossref] [PubMed]
- Yang Q, Ge MQ, Kokalari B, et al. Group 2 innate lymphoid cells mediate ozone-induced airway inflammation and hyperresponsiveness in mice. J Allergy Clin Immunol 2016;137:571-8. [Crossref] [PubMed]
- Ashrafi G, Schwarz TL. The pathways of mitophagy for quality control and clearance of mitochondria. Cell Death Differ 2013;20:31-42. [Crossref] [PubMed]
- Ganetzky RD, Stendel C, McCormick EM, et al. MT-ATP6 mitochondrial disease variants: Phenotypic and biochemical features analysis in 218 published cases and cohort of 14 new cases. Hum Mutat 2019;40:499-515. [Crossref] [PubMed]
- Hara H, Kuwano K, Araya J. Mitochondrial Quality Control in COPD and IPF. Cells 2018;7:86. [Crossref] [PubMed]
- Patrushev MV, Mazunin IO, Vinogradova EN, et al. Mitochondrial Fission and Fusion. Biochemistry (Mosc) 2015;80:1457-64. [Crossref] [PubMed]
- Tsubouchi K, Araya J, Kuwano K. PINK1-PARK2-mediated mitophagy in COPD and IPF pathogeneses. Inflamm Regen 2018;38:18. [Crossref] [PubMed]
- Hadrava Vanova K, Kraus M, Neuzil J, et al. Mitochondrial complex II and reactive oxygen species in disease and therapy. Redox Rep 2020;25:26-32. [Crossref] [PubMed]
- Akopian AN, Fanick ER, Brooks EG. TRP channels and traffic-related environmental pollution-induced pulmonary disease. Semin Immunopathol 2016;38:331-8. [Crossref] [PubMed]
- Bonvini SJ, Belvisi MG. Cough and airway disease: The role of ion channels. Pulm Pharmacol Ther 2017;47:21-8. [Crossref] [PubMed]
- Trevisan G, Benemei S, Materazzi S, et al. TRPA1 mediates trigeminal neuropathic pain in mice downstream of monocytes/macrophages and oxidative stress. Brain 2016;139:1361-77. [Crossref] [PubMed]
- Gültekin F, Nazıroğlu M, Savaş HB, et al. Calorie restriction protects against apoptosis, mitochondrial oxidative stress and increased calcium signaling through inhibition of TRPV1 channel in the hippocampus and dorsal root ganglion of rats. Metab Brain Dis 2018;33:1761-74. [Crossref] [PubMed]
- Lambert JA, Song W. Ozone-induced airway hyperresponsiveness: roles of ROCK isoforms. Am J Physiol Lung Cell Mol Physiol 2015;309:L1394-7. [Crossref] [PubMed]
- Taylor-Clark TE, Undem BJ. Ozone activates airway nerves via the selective stimulation of TRPA1 ion channels. J Physiol 2010;588:423-33. [Crossref] [PubMed]
- Wang M, Zhang Y, Xu M, et al. Roles of TRPA1 and TRPV1 in cigarette smoke -induced airway epithelial cell injury model. Free Radic Biol Med 2019;134:229-38. [Crossref] [PubMed]
- Xu M, Zhang Y, Wang M, et al. TRPV1 and TRPA1 in Lung Inflammation and Airway Hyperresponsiveness Induced by Fine Particulate Matter (PM2.5). Oxid Med Cell Longev 2019;2019:7450151. [Crossref] [PubMed]
- Sakai H, Suto W, Kai Y, et al. Mechanisms underlying the pathogenesis of hyper-contractility of bronchial smooth muscle in allergic asthma. J Smooth Muscle Res 2017;53:37-47. [Crossref] [PubMed]
- McGovern TK, Chen M, Allard B, et al. Neutrophilic oxidative stress mediates organic dust-induced pulmonary inflammation and airway hyperresponsiveness. Am J Physiol Lung Cell Mol Physiol 2016;310:L155-65. [Crossref] [PubMed]
- Cockcroft DW, Davis BE. Mechanisms of airway hyperresponsiveness. J Allergy Clin Immunol 2006;118:551-9; quiz 560-1. [Crossref] [PubMed]
- Verhein KC, Hazari MS, Moulton BC, et al. Three days after a single exposure to ozone, the mechanism of airway hyperreactivity is dependent on substance P and nerve growth factor. Am J Physiol Lung Cell Mol Physiol 2011;300:L176-84. [Crossref] [PubMed]
- Barker JS, Wu Z, Hunter DD, et al. Ozone exposure initiates a sequential signaling cascade in airways involving interleukin-1beta release, nerve growth factor secretion, and substance P upregulation. J Toxicol Environ Health A 2015;78:397-407. [Crossref] [PubMed]
- Gabehart K, Correll KA, Loader JE, et al. The lung response to ozone is determined by age and is partially dependent on toll-Like receptor 4. Respir Res 2015;16:117. [Crossref] [PubMed]
- Dinarello CA, Novick D, Kim S, et al. Interleukin-18 and IL-18 binding protein. Front Immunol 2013;4:289. [Crossref] [PubMed]
- Che L, Jin Y, Zhang C, et al. Ozone-induced IL-17A and neutrophilic airway inflammation is orchestrated by the caspase-1-IL-1 cascade. Sci Rep 2016;6:18680. [Crossref] [PubMed]
- Dröge W. Free radicals in the physiological control of cell function. Physiol Rev 2002;82:47-95. [Crossref] [PubMed]
- Barnes PJ. Inflammatory mechanisms in patients with chronic obstructive pulmonary disease. J Allergy Clin Immunol 2016;138:16-27. [Crossref] [PubMed]
- Del Rio D, Stewart AJ, Pellegrini N. A review of recent studies on malondialdehyde as toxic molecule and biological marker of oxidative stress. Nutr Metab Cardiovasc Dis 2005;15:316-28. [Crossref] [PubMed]
- Liu RM, Gaston Pravia KA. Oxidative stress and glutathione in TGF-beta-mediated fibrogenesis. Free Radic Biol Med 2010;48:1-15. [Crossref] [PubMed]
- Zhang JH, Yang X, Chen YP, et al. Nrf2 Activator RTA-408 Protects Against Ozone-Induced Acute Asthma Exacerbation by Suppressing ROS and γδT17 Cells. Inflammation 2019;42:1843-56. [Crossref] [PubMed]
- Ogawa N, Kurokawa T, Mori Y. Sensing of redox status by TRP channels. Cell Calcium 2016;60:115-22. [Crossref] [PubMed]
- Dietrich A, Steinritz D, Gudermann T. Transient receptor potential (TRP) channels as molecular targets in lung toxicology and associated diseases. Cell Calcium 2017;67:123-37. [Crossref] [PubMed]
- Liu Z, Wang P, Lu S, et al. Liquiritin, a novel inhibitor of TRPV1 and TRPA1, protects against LPS-induced acute lung injury. Cell Calcium 2020; [Epub ahead of print]. [Crossref] [PubMed]
- Chan DC. Mitochondrial Dynamics and Its Involvement in Disease. Annu Rev Pathol 2020;15:235-59. [Crossref] [PubMed]
- Youle RJ, van der Bliek AM. Mitochondrial fission, fusion, and stress. Science 2012;337:1062-5. [Crossref] [PubMed]
- Cid-Castro C, Hernández-Espinosa DR, Morán J. ROS as Regulators of Mitochondrial Dynamics in Neurons. Cell Mol Neurobiol 2018;38:995-1007. [Crossref] [PubMed]
- Xu M, Wang L, Wang M, et al. Mitochondrial ROS and NLRP3 inflammasome in acute ozone-induced murine model of airway inflammation and bronchial hyperresponsiveness. Free Radic Res 2019;53:780-90. [Crossref] [PubMed]
- Mizumura K, Cloonan SM, Nakahira K, et al. Mitophagy-dependent necroptosis contributes to the pathogenesis of COPD. J Clin Invest 2014;124:3987-4003. [Crossref] [PubMed]
- Zhang M, Shi R, Zhang Y, et al. Nix/BNIP3L-dependent mitophagy accounts for airway epithelial cell injury induced by cigarette smoke. J Cell Physiol 2019;234:14210-20. [Crossref] [PubMed]
- Nolfi-Donegan D, Braganza A, Shiva S. Mitochondrial electron transport chain: Oxidative phosphorylation, oxidant production, and methods of measurement. Redox Biol 2020;37:101674. [Crossref] [PubMed]