Phrenic nerve stimulation in an ovine model with temporary removable pacing leads
Introduction
Mechanical ventilation leads to underuse of the respiratory muscles, which may result in diaphragm dysfunction within a few hours (1,2). Ventilation-induced diaphragm dysfunction is a risk factor for weaning failure and is associated with poor outcomes and increased morbidity and mortality in the intensive care unit (3-5). The mechanisms of ventilation-induced diaphragm dysfunction include time-dependent muscle atrophy which occurs because of changes in microstructure: no preventive or curative treatments are currently available (6-9). It has been proposed that electrical pacing of the diaphragm could prevent or alleviate ventilation-induced diaphragm dysfunction (10-12). Phrenic nerve neurostimulation has already been used in patients with high spinal injuries for short-term weaning from mechanical ventilation and for long-term ventilation where weaning is not possible (13,14). Critically ill patients may benefit from temporary phrenic nerve neurostimulation to mitigate ventilator-induced diaphragm dysfunction and to anticipate and prevent weaning failure, which may be of particular interest in thoracic/cardiac surgery patients (15-17). The present study evaluated a new method of electrical pacing of the diaphragm by stimulation of the distal portion of the phrenic nerve, which is free from the pericardium in the cardiophrenic angle and easily accessible through anterior thoracotomy (18). The objectives of the present study were to assess the feasibility and safety of a surgically implanted and non-invasively removable temporary neurostimulation system in an ovine model using the distal portion of the phrenic nerve. We investigated the hypothesis that this technique would enable similar minute-ventilation compare to mechanical ventilation. We present the following article in accordance with the ARRIVE reporting checklist (available at https://jtd.amegroups.com/article/view/10.21037/jtd-21-1944/rc).
Methods
This interventional animal study used an adult female ovine model and was carried out at the Carpentier laboratory (Georges Pompidou European Hospital, Paris, France) in accordance with the guidelines for the care and use of laboratory animals. This study was approved by the French Ministry of Research and Superior Studies, University of Paris institutional ethical committee and received authorization under the number APAFIS#26050-2020041918484991 v5.
Animal preparation
Surgery was performed after induction of general anesthesia using intravenous propofol (4 mg/kg). Animals were intubated orally and no muscle paralysis was used. Minute-ventilation was set to ensure normocapnia (Evita XL, Dräger, Germany). Volume-controlled ventilation was used with initial tidal volume set to 8 mL/kg of ideal body weight, respiratory rate of 18 breaths·min−1, and positive end-expiratory pressure set to 5 cmH2O. Continuous fluid infusion was provided during the procedure, and electrocardiogram (ECG) and transcutaneous oxygen saturation were monitored.
Surgical procedure and implantation of the phrenic nerve electrodes
Right and left anterior thoracotomy in the 7th intercostal space were used to access the phrenic nerves (Figure 1A) in 4 anaesthetized female animals. Median weight of the animals was 43 kg (95% CI: 30–45 kg). Median diameter of the right and left phrenic nerves was respectively, 2 mm (95% CI: 1.21–2.90 mm) and 1.305 mm (95% CI: 1.12–1.7 mm).
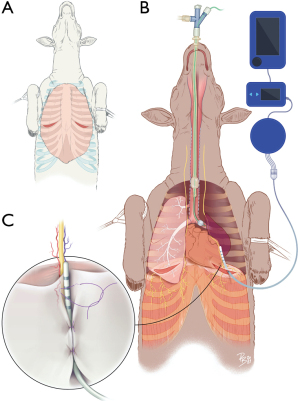
Surgery was performed first on the right side. Lung exclusion for uni-pulmonary ventilation was done using EZ-blocker (Cook Medical, Bloomington, Indiana, USA) (Figure 1B). The trunk of the phrenic nerve was then identified and followed to its distal portion. Access to the distal portion of the right and left phrenic nerves before entry to the diaphragm was obtained by moving the lung parenchyma manually. A prototype temporary phrenic pacer lead was positioned and maintained on the distal portion of the phrenic nerves using absorbable thread (polyglactine 910 3/0, Ethicon, New Jersey, USA) (Figure 1C). The temporary phrenic nerve stimulator (tPNS) electrode was a biocompatible electrode (Atrotech, Tampere, Finland) that was specially designed for this study. The electrode was 16 mm long with a diameter of 1.35 mm and comprised 4 contact rings, each separated by 4 mm. The 4 contact rings allowed sequential multipolar simulation. Only a portion of motor units in the diaphragm muscle were activated by a single stimulus pulse. Most of the motor units were at rest during successive stimulus pulses, allowing an extended recovery time after the contractile phase.
The lung was then re-inflated to release any potential atelectasis, and the procedure was repeated on the left side. The electrode wires were externalized through intercostal spaces and connected to the stimulator to initiate the stimulation sessions (see below).
Phrenic nerve stimulation settings
Day 1 stimulator settings were respiratory rate of 18 breaths·min−1, pulse duration 200 µs and pulse frequency of 20 Hz (adapted from previous investigations (19,20). Correct functioning of the stimulations was checked by visual examination directly inside the chest and palpation of the abdomen.
Experimental protocol
After the preparation described above, bilateral phrenic nerve stimulations were applied for 15 minutes (first session). During this time, mechanical ventilation was stopped and oxygen administration was maintained. Diaphragm contraction from the stimulator allowed the animals to inspire air enriched in oxygen from the ventilation unit and expire air into the ventilation unit (Video 1). We were able to assess ventilation-minute by using the ventilation unit monitor. The electrode wires were then disconnected from the stimulator and concealed under the skin, and the chest was surgically closed. The animals were woken up and returned to their care facility. On day 15, two of the animals were anaesthetized and a neurostimulation session was conducted using each electrode separately (second session). Bilateral thoracotomy was then performed to allow visualization of the percutaneous removal of the electrodes. Afterwards, two portions of the phrenic nerve were harvested for pathological examination: a proximal portion that was not in contact with the electrode and a distal portion that was in contact and stimulated by the electrode. At day 30, the two remaining animals underwent the same procedure as described for day 15. Animals were sacrificed after the end of the procedure on day 15 or day 30.
Primary and secondary endpoints
The primary endpoint was the ability to successfully match the animal’s minute ventilation upon implantation of both phrenic nerve pacers on day 1. This was compared to ventilation-minute produced by mechanical ventilation only. Ventilation-minute over 1 minute was obtained from the ventilator screen before initiating bilateral neurostimulation and at the end of the 15-minute stimulation sessions.
Secondary endpoints were successful neurostimulation by both electrodes on day 15 and day 30 using the same thresholds as day 1. We also assessed the safe removal of the electrodes on day 15 or day 30. The integrity of right and left phrenic nerves was confirmed by pathologic analysis.
Pathological analysis
Each portion of the phrenic nerve was fixed by immersion in 4% paraformaldehyde, embedded in paraffin, and sectioned in 4 µm slices. Axial sections of the phrenic nerve were then obtained. After hematoxylin-eosin staining, microscopic evaluation was conducted to assess the integrity of the distal portion of the phrenic nerve. This was compared to the proximal portion that had not been in contact with the electrode (Leica Microsystems, Switzerland; nanozoomer digital slide scanner and NDP viewing software, Hamamatsu photonics, Japan).
Statistical analysis
Categorical variables were presented as numbers and proportions, and were compared using Fisher’s exact test. Continuous variables were reported as median (95% confidence interval) and compared using the Wilcoxon test. A P value less than 0.05 was considered to be statistically significant. Statistics were done using Prism GraphPad© (San Diego, CA, USA).
Results
Electrode implantation and phrenic nerve neurostimulation
Six of eight leads were successfully implanted into three of the four animals and neurostimulation was considered effective for each of these three animals (Table 1). In the first ovine model, it was impossible to fix the electrode on the right phrenic nerve because of intense pleural adhesions. No specific diagnosis of the right lung could be determined after sacrifice and pathologic analysis. In the same ovine model, we were able to place the electrode on the left phrenic nerve but it was not possible to pace the diaphragm because of technical difficulties.
Table 1
Animal | Side | Electrode implantation | Pacing | ||
---|---|---|---|---|---|
First session | Day 15 | Day 30 | |||
Animal #1 | Left phrenic nerve | Possible | Not possible | Possible | ![]() |
Right phrenic nerve | Not possible | Not possible | Not possible | ![]() |
|
Animal #2 | Left phrenic nerve | Possible | Possible | Possible | ![]() |
Right phrenic nerve | Possible | Possible | Not possible | ![]() |
|
Animal #3 | Left phrenic nerve | Possible | Possible | ![]() |
Possible |
Right phrenic nerve | Possible | Possible | ![]() |
Possible | |
Animal #4 | Left phrenic nerve | Possible | Possible | ![]() |
Possible |
Right phrenic nerve | Possible | Possible | ![]() |
Not possible |
For the six successfully implanted electrodes, median stimulation threshold was significantly higher on the right phrenic nerve (0.7 mA; 95% CI: 0.6–1.0 mA) compared to the left phrenic nerve (0.5 mA; 95% CI: 0.4–0.7 mA) (P=0.01) (Figure 2A).
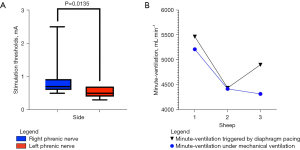
Minute ventilation
On day 1 (controlled-volume ventilation), median minute-ventilation was 4.4 L·min-1 (95% CI: 4.3–5.2 L·min-1) which was not significantly different from the minute-ventilation produced during the first bilateral phrenic nerves neurostimulation session (4.9 L·min-1; 95% CI: 4.4–5.5 L·min-1) (P=0.40) (Figure 2B).
Neurostimulation on day 15 and day 30
Neurostimulation was successful on day 15 and day 30 in all left phrenic nerves (n=4). On the left side, the level of the thresholds was the same as the day of implantation (0.5 mA; 95% CI: 0.4–0.7 mA). In two of the right side phrenic nerves, stimulation was ineffective due to an electrode displacement. The remaining right electrode kept the same thresholds of stimulation as the first day of implantation. Percutaneous removal of the electrodes was possible for all the electrodes (n=6) except one (n=1) of the displaced right side electrodes. This electrode was blocked in intense fibrotic tissue in the pulmonary fissure. Table 1 summarizes electrode implantation and efficacy in each animal.
Pathologic analysis
Pathologic analysis showed integrity of distal portion of the phrenic nerve after percutaneous removal (Figure 3). Fibrotic tissue was visible in the mediastinal pleura that was in contact with the electrodes 15 days after implantation. In Animal #1, the intense fibrotic tissue in the left phrenic nerve was characterized by the presence of fibroblastic cells (blue arrow); yellow arrows indicate the presence of absorbable thread. The same intense fibrotic tissue was visible in Animal #2 (blue arrow) with an intact left phrenic nerve. However, the right phrenic nerve did not show any fibrotic tissue, as the electrode was displaced. Thirty days after implantation, fibrotic tissue was either completely absent (Animal #4) or minimal (Animal #3, blue arrow). The phrenic nerves were all intact.
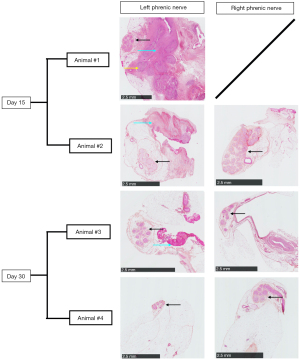
Discussion
In this experimental study, we explored the feasibility and safety of a new anatomical approach enabling bilateral temporary phrenic nerve stimulation. Specially designed electrodes were surgically implanted on the distal portion of the phrenic nerve. Efficient bilateral neurostimulation was possible in 75% (3 out of 4) of the animals included on day 1. Both the right and left distal portion of the phrenic nerve were easily accessible through an anterior mini-thoracotomy without any additional intra-thoracic dissection. This approach minimizes the risk of injuring the phrenic nerve. Even though the current thresholds were significantly different on the right and left phrenic nerve, they remained low (<1 mA) and were comparable to those reported for conventional intra-thoracic phrenic neurostimulation (21). With the low current threshold, we were able to provide efficient bilateral neurostimulation at baseline. This is important as it minimizes the risk of adverse events such as referred shoulder pain or cardiac arrhythmias that have been seen in other stimulation techniques (10,22).
It was possible to provide efficient minute-ventilation 15 and 30 days after implantation. Upon withdrawal of the electrodes, the phrenic nerves were visually intact, which was confirmed by the pathological analysis. The development of fibrotic tissue due to the presence of the electrode was limited to the thoracic cavity. This did not directly affect the phrenic nerve, which was protected by the mediastinal pleura. This inflammatory reaction seemed to decrease over time and was less evident on day 30 than day 15. The use of rapidly absorbable surgical thread allowed the safe removal of the electrode without resistance.
However, diaphragm neurostimulation was not possible in all animals. At day 1, we faced technical problems related to the learning curve of a new stimulation system and an ill animal. In addition, at day 15 and day 30, displacement of the electrode on the right phrenic nerve was observed in one animal. Importantly, no surgical complications occurred in any animal. The temporary feasibility of the technique was demonstrated, and it appeared to be safe for both the animals and the phrenic nerves. However, following the significant displacement of the leads on the right side, further research is needed to improve the design of the leads and the method of fixation before starting clinical studies in patients undergoing cardio-thoracic surgery.
Short-term phrenic or direct diaphragmatic pacing may be an option to preserve diaphragmatic structure and function. It may slow the biological mechanisms responsible for ventilator induced diaphragmatic dysfunction and have a consequent impact on morbidity and mortality. In the future, temporary phrenic stimulation may help optimise ventilator management in patients with respiratory failure (23). We previously published a pilot study of unilateral phrenic nerve neurostimulation in an ovine model and showed reduced muscle atrophy and muscle fiber injury in the neurostimulated hemi-diaphragm (12). These results have been corroborated by other experimental studies: pigs under mechanical ventilation that received transvenous phrenic nerve stimulation had less diaphragm atrophy (11). Similarly, in small cohort of cardio-thoracic patients, intermittent neurostimulation during surgery significantly increased the force of specific myofibers and mitochondrial respiration (24,25). Markers of oxidative stress are also significantly decreased in the stimulated hemi-diaphragm (26). In post-operative cardio-thoracic patients or patients in intensive care under prolonged mechanical ventilation, single arm studies have confirmed the feasibility and safety of specifically designed electrodes for phrenic nerve stimulation (15,27-29). However, the potential benefits of temporary phrenic nerve neurostimulation still need to be confirmed by randomized controlled trials (10).
Diaphragmatic stimulation, whether via the motor points within the diaphragm itself or via phrenic stimulation, has been used successfully for decades in the context of high spinal cord injuries (14,30). Outside the scope of central respiratory paralysis, providing neurostimulation of the diaphragm may be useful in different clinical scenarios (8). First, it can be used in critically ill patients to improve the likelihood of successful weaning (10). It could be also used to enhance diaphragm contraction after major cardiac or thoracic surgery (16,17). Such strategy would require phrenic nerve electrodes to be positioned during the surgery and subsequently removed non-invasively as is currently the case for epicardial electrodes. Our approach offers this possibility. We only studied bilateral diaphragm stimulation to stabilize minute-ventilation. We cannot confirm that we can stabilize minute-ventilation with only a single hemidiaphragm stimulation. Further investigations are necessary to assess this possibility as well as effect on esophageal pressure and tidal volume. Randomized controlled clinical trials will be necessary to establish whether phrenic nerve neurostimulation during the post-surgery period in intubated patients can be effective in reducing the risk of atelectasis and pneumonia, leading to improved outcomes.
Our experimental animal study aimed to assess the feasibility and safety of a novel temporary neurostimulation system. One significant limitation was the secondary displacement of two electrodes, by day 15 and 30, both in the right thoracic cavity. This can be accounted for by anatomical considerations. Mediastinal pleura in sheep are thinner than in humans and tear more easily when manipulated. Moreover, in the right thoracic cavity of the ovine model, the phrenic nerve is in close proximity to both the superior and inferior vena cava, which complicates the attachment of the electrode compared to the left thoracic cavity. Since anatomic relations of the right phrenic nerve with the right atrium in ovine models are limited, we were aware of possible vascular injury and the electrodes on the right side may have been attached less tightly than on the left side. As a result, although the initial stimulation was efficient, on the right, side two lead displacements occurred following the first surgical procedure. Such difficulties would not be expected in humans as the right phrenic nerve in humans descends along the right atrium before terminating in the diaphragm, in front of the inferior vena cava. Furthermore, it is accompanied by fatty tissue that is not present in the ovine model (18).
Other limitations of this study included the small number of animals. We were able, however, to achieve the primary objective of the study and address the secondary endpoints. It was therefore ethical to refrain from further including other animals to respect the 3 Rs principle: replace, reduce, and refine (31). Further studies will be necessary to assess implantation of the electrodes in a clinical setting.
Conclusions
In an experimental animal model, the use of temporary and easily removable electrodes for neurostimulation of the distal portion of the phrenic nerve was feasible and safe. Neurostimulation remained possible at day 15 and day 30 if the electrodes were still in contact with the phrenic nerves. Pathological analysis confirmed that the electrodes could safely be removed percutaneously at the end of the procedure without any damage to the phrenic nerve.
Acknowledgments
The authors would like to thank Serge Renaux (Neuroresp, Montpellier, France) who supervised reception of the tPNS electrodes and their stimulation system in France, and who provided assistance in the design of the electrodes. We also thank Tommi Majaus (Atrotech Oy, Tampere, Finland) who supervised the manufacturing of the electrodes and their shipment to France. This study was presented as poster presentation at the 29th European Society Thoracic Surgery Congress from June 20th to June 22nd.
Funding: The study was funded by Atrotech Oy (Tampere, Finland) (grant number C20/0037-P20/1445) in order to buy the animals used.
Footnote
Reporting Checklist: The authors have completed the ARRIVE reporting checklist. Available at https://jtd.amegroups.com/article/view/10.21037/jtd-21-1944/rc
Data Sharing Statement: Available at https://jtd.amegroups.com/article/view/10.21037/jtd-21-1944/dss
Peer Review File: Available at https://jtd.amegroups.com/article/view/10.21037/jtd-21-1944/prf
Conflicts of Interest: All authors have completed the ICMJE uniform disclosure form (available at https://jtd.amegroups.com/article/view/10.21037/jtd-21-1944/coif). All authors report funding of the experimental procedures by Atrotech (Tampere, Finland). The authors have no other conflicts of interest to declare.
Ethical Statement: The authors are accountable for all aspects of the work in ensuring that questions related to the accuracy or integrity of any part of the work are appropriately investigated and resolved. This study was approved by the French Ministry of Research and Superior Studies, University of Paris institutional ethical committee and received authorization under the number APAFIS#26050-2020041918484991 v5. This interventional animal study used an adult female ovine model and was carried out at the Carpentier laboratory (Georges Pompidou European Hospital, Paris, France) in accordance with the guidelines for the care and use of laboratory animals.
Open Access Statement: This is an Open Access article distributed in accordance with the Creative Commons Attribution-NonCommercial-NoDerivs 4.0 International License (CC BY-NC-ND 4.0), which permits the non-commercial replication and distribution of the article with the strict proviso that no changes or edits are made and the original work is properly cited (including links to both the formal publication through the relevant DOI and the license). See: https://creativecommons.org/licenses/by-nc-nd/4.0/.
References
- Levine S, Nguyen T, Taylor N, et al. Rapid disuse atrophy of diaphragm fibers in mechanically ventilated humans. N Engl J Med 2008;358:1327-35. [Crossref] [PubMed]
- Welvaart WN, Paul MA, Stienen GJ, et al. Selective diaphragm muscle weakness after contractile inactivity during thoracic surgery. Ann Surg 2011;254:1044-9. [Crossref] [PubMed]
- Dres M, Dubé BP, Mayaux J, et al. Coexistence and Impact of Limb Muscle and Diaphragm Weakness at Time of Liberation from Mechanical Ventilation in Medical Intensive Care Unit Patients. Am J Respir Crit Care Med 2017;195:57-66. [Crossref] [PubMed]
- Dres M, Goligher EC, Heunks LMA, et al. Critical illness-associated diaphragm weakness. Intensive Care Med 2017;43:1441-52. [Crossref] [PubMed]
- Béduneau G, Pham T, Schortgen F, et al. Epidemiology of Weaning Outcome according to a New Definition. The WIND Study. Am J Respir Crit Care Med 2017;195:772-83. [Crossref] [PubMed]
- Anzueto A, Peters JI, Tobin MJ, et al. Effects of prolonged controlled mechanical ventilation on diaphragmatic function in healthy adult baboons. Crit Care Med 1997;25:1187-90. [Crossref] [PubMed]
- Le Bourdelles G, Viires N, Boczkowski J, et al. Effects of mechanical ventilation on diaphragmatic contractile properties in rats. Am J Respir Crit Care Med 1994;149:1539-44. [Crossref] [PubMed]
- Goligher EC, Dres M, Patel BK, et al. Lung- and Diaphragm-Protective Ventilation. Am J Respir Crit Care Med 2020;202:950-61. [Crossref] [PubMed]
- Schellekens WJ, van Hees HW, Doorduin J, et al. Strategies to optimize respiratory muscle function in ICU patients. Crit Care 2016;20:103. [Crossref] [PubMed]
- Evans D, Shure D, Clark L, et al. Temporary transvenous diaphragm pacing vs. standard of care for weaning from mechanical ventilation: study protocol for a randomized trial. Trials 2019;20:60. [Crossref] [PubMed]
- Reynolds SC, Meyyappan R, Thakkar V, et al. Mitigation of Ventilator-induced Diaphragm Atrophy by Transvenous Phrenic Nerve Stimulation. Am J Respir Crit Care Med 2017;195:339-48. [Crossref] [PubMed]
- Masmoudi H, Coirault C, Demoule A, et al. Can phrenic stimulation protect the diaphragm from mechanical ventilation-induced damage? Eur Respir J 2013;42:280-3. [Crossref] [PubMed]
- Glenn WW, Hogan JF, Loke JS, et al. Ventilatory support by pacing of the conditioned diaphragm in quadriplegia. N Engl J Med 1984;310:1150-5. [Crossref] [PubMed]
- Le Pimpec-Barthes F, Gonzalez-Bermejo J, Hubsch JP, et al. Intrathoracic phrenic pacing: a 10-year experience in France. J Thorac Cardiovasc Surg 2011;142:378-83. [Crossref] [PubMed]
- Ataya A, Silverman EP, Bagchi A, et al. Temporary Transvenous Diaphragmatic Neurostimulation in Prolonged Mechanically Ventilated Patients: A Feasibility Trial (RESCUE 1). Crit Care Explor 2020;2:e0106. [Crossref] [PubMed]
- Spadaro S, Grasso S, Dres M, et al. Point of Care Ultrasound to Identify Diaphragmatic Dysfunction after Thoracic Surgery. Anesthesiology 2019;131:266-78. [Crossref] [PubMed]
- Bruni A, Garofalo E, Pasin L, et al. Diaphragmatic Dysfunction After Elective Cardiac Surgery: A Prospective Observational Study. J Cardiothorac Vasc Anesth 2020;34:3336-44. [Crossref] [PubMed]
- Etienne H, Gonzalez-Bermejo J, Dres M, et al. The terminal segment of the human phrenic nerve as a novel implantation site for diaphragm pacing electrodes: Anatomical and clinical description. Ann Anat 2022;239:151835. [Crossref] [PubMed]
- Masmoudi H, Persichini R, Cecchini J, et al. Corrective effect of diaphragm pacing on the decrease in cardiac output induced by positive pressure mechanical ventilation in anesthetized sheep. Respir Physiol Neurobiol 2017;236:23-8. [Crossref] [PubMed]
- Assouad J, Masmoudi H, Gonzalez-Bermejo J, et al. Diaphragm pacing after bilateral implantation of intradiaphragmatic phrenic stimulation electrodes through a transmediastinal endoscopic minimally invasive approach: pilot animal data. Eur J Cardiothorac Surg 2012;42:333-9. [Crossref] [PubMed]
- Hirschfeld S, Vieweg H, Schulz AP, et al. Threshold currents of platinum electrodes used for functional electrical stimulation of the phrenic nerves for treatment of central apnea. Pacing Clin Electrophysiol 2013;36:714-8. [Crossref] [PubMed]
- Morélot-Panzini C, Le Pimpec-Barthes F, Menegaux F, et al. Referred shoulder pain (C4 dermatome) can adversely impact diaphragm pacing with intramuscular electrodes. Eur Respir J 2015;45:1751-4. [Crossref] [PubMed]
- Reardon PM, Wong J, Fitzpatrick A, et al. Diaphragm function in acute respiratory failure and the potential role of phrenic nerve stimulation. Curr Opin Crit Care 2021;27:282-9. [Crossref] [PubMed]
- Martin AD, Joseph AM, Beaver TM, et al. Effect of intermittent phrenic nerve stimulation during cardiothoracic surgery on mitochondrial respiration in the human diaphragm. Crit Care Med 2014;42:e152-6. [Crossref] [PubMed]
- Ahn B, Beaver T, Martin T, et al. Phrenic nerve stimulation increases human diaphragm fiber force after cardiothoracic surgery. Am J Respir Crit Care Med 2014;190:837-9. [Crossref] [PubMed]
- Mankowski RT, Ahmed S, Beaver T, et al. Intraoperative hemidiaphragm electrical stimulation reduces oxidative stress and upregulates autophagy in surgery patients undergoing mechanical ventilation: exploratory study. J Transl Med 2016;14:305. [Crossref] [PubMed]
- Testelmans D, Nafteux P, Van Cromphaut S, et al. Feasibility of diaphragm pacing in patients after bilateral lung transplantation. Clin Transplant 2017; [Crossref] [PubMed]
- O'Rourke J, Soták M, Curley GF, et al. Initial Assessment of the Percutaneous Electrical Phrenic Nerve Stimulation System in Patients on Mechanical Ventilation. Crit Care Med 2020;48:e362-70. [Crossref] [PubMed]
- Keough-Delgado E, López-Rodríguez L, de Olaiz B, et al. Case Studies in Physiology: Physiological and clinical effects of temporary diaphragm pacing in two patients with ventilator-induced diaphragm dysfunction. J Appl Physiol (1985) 2021;130:1736-42. [Crossref] [PubMed]
- DiMarco AF, Onders RP, Kowalski KE, et al. Phrenic nerve pacing in a tetraplegic patient via intramuscular diaphragm electrodes. Am J Respir Crit Care Med 2002;166:1604-6. [Crossref] [PubMed]
- Tannenbaum J, Bennett BT. Russell and Burch's 3Rs then and now: the need for clarity in definition and purpose. J Am Assoc Lab Anim Sci 2015;54:120-32. [PubMed]