Skeletal muscle and related protein expression as prognostic factors in thymic squamous cell carcinoma
Introduction
Thymic epithelial tumors are rare malignancies that originate in the thymus. Among them, thymic carcinomas (TCs) are the most aggressive subtype and constitute just over 10% of such tumors (1). TCs have a 5-year survival rate of 51%, which is significantly lower than that of thymoma, which is 94% (2). Thymic squamous cell carcinoma (TSQCC) is a major subtype of TC, which includes 14 subtypes (3). Surgical resection is the only curative treatment for patients with TCs, and complete resection is the most important predictor of survival (4). Multimodal treatment, which includes chemotherapy, surgery, and radiotherapy, is considered for patients with advanced disease. TCs also show a high expression of programmed death-ligand 1 (PD-L1) (5), which has been correlated with a clinical response to programmed death-1 (PD-1) antibodies, as suggested in a phase II study (6). Several studies have assessed the predictors of favorable survival among patients with TCs, such as complete resection, pathological stage (4), and PD-L1 expression (7). However, the clinicopathological characteristics and prognostic factors of patients with TSQCC have not been elucidated well owing to the rarity of this disease.
Sarcopenia and its surrogate marker, the psoas muscle index (PMI), have recently received considerable attention in the field of oncology as a prognostic factor for various types of cancers, including gastric, esophageal, ovarian, and lung cancer (8,9). We previously reported that PMI is significantly associated with the survival of patients with lung squamous cell carcinoma (10). To date, however, no study has assessed the relationship between TSQCC and PMI. In our study, we also focused on the RNA-binding protein, fragile X-related 1 (FXR1). FXR1 has been found to play an essential role in normal myogenesis by editing transcription profiles as part of an RNA-induced silencing complex (11), and FXR1 is highly expressed in myocytes. Mutations or altered expressions of RNA-binding proteins can cause dysfunction in multiple biological and pathological processes, leading to diseases such as cancer (12). FXR1 overexpression in cancer is a candidate biomarker for poor survival in various types of cancers, including lung cancer, breast cancer, and oral squamous cell carcinoma (13-15). Therefore, we hypothesized that FXR1 expression was a prognostic factor in patients with TSQCC. However, the significance of FXR1 expression and its prognostic value in TSQCC are as yet unknown. Thus, the role of skeletal muscle mass in TSQCC progression and the relationship between FXR1 expression and the clinicopathological features of TSQCC are unclear. We therefore investigated the prognostic significance of PMI in patients with TSQCC, considering the status of FXR1 and PD-L1 using immunohistochemical analysis of TSQCC clinical samples obtained during surgical resection. We present the following article in accordance with the STROBE reporting checklist (available at https://jtd.amegroups.com/article/view/10.21037/jtd-22-385/rc).
Methods
Patients and samples
The study was conducted in accordance with the Declaration of Helsinki (as revised in 2013). The study was approved by the Institutional Review Board of Nagoya University Hospital (approval No. 2021-0236). Individual consent for this retrospective analysis was waived. In total, 215 patients underwent surgery for primary thymic epithelial tumors, including TCs and thymomas, at Nagoya University Hospital between January 2006 and December 2018. Of these, 34 patients (15.8%) whose resected specimens were histologically reviewed and confirmed to be TSQCC by a pathologist (H.T) were enrolled in the present study. We retrospectively analyzed these 34 patients to assess the relationship between PMI and clinicopathological characteristics, including FXR1 and PD-L1 expression, and the prognosis. Institutional databases and each patient’s medical records were retrospectively reviewed to determine the clinicopathological characteristics including age, sex, body mass index (BMI), serum albumin and cytokeratin fragment (CYFRA) levels, forced expiratory volume in one second (FEV1.0), diffusing capacity of the lungs for carbon monoxide (DLco), curability, prognosis, and pathological tumor-node-metastasis staging based on the Masaoka-Koga classification (16) and the TNM classification (17).
PMI measurement
All preoperative computed tomography (CT) images were evaluated 1–2 months before surgery. The PMI evaluation was performed as previously described (10). In brief, the cross-sectional area of the bilateral psoas muscle at the umbilical level during preoperative CT was measured by manual tracing using a picture archive and communication system. Previously, Hamaguchi et al. examined the sex distribution of PMI in 541 patients who underwent living-donor liver transplantation and found that the mean PMI was significantly higher in males than in females (8.85 vs. 5.77) (18). These mean PMI values were used to adjust the total psoas area for individual height using the following equation:
PMI (%) = cross-sectional area of the bilateral psoas muscle (cm2)/[height (m)2 ×8.85 (for males) or 5.77 (for females)].
Histopathology and immunohistochemistry
For the histopathological evaluation, we employed histology glass slides stained with hematoxylin and eosin. The histological type and differentiation were determined according to the World Health Organization classification (3). Differentiation was classified into the following three subgroups based on the presence or absence of keratinization, the degree of nuclear pleomorphism, and the extent of squamous cell maturation: well differentiated, moderately differentiated, and poorly differentiated. The representative slides are shown in Figure 1. For immunohistochemical analysis, unstained glass slides from preserved formalin-fixed paraffin-embedded blocks were available for each case. Each section was cut to a 4-µm thickness. Immunohistochemical staining for FXR1 (13) and PD-L1 evaluations (19) was performed as described in previous reports. The primary antibody used for FXR1 in the present study was rabbit polyclonal antihuman antibody (clone HPA018246; Sigma-Aldrich, Saint Louis, MO, USA). Cytoplasmic staining of FXR1 was scored using the Allred system with slight modifications, as previously reported (13). In brief, the staining index was considered to be the sum of the intensity score (0, no staining; 1+, weak; 2+, moderate; 3+, strong) (Figure 2A) and the distribution score (0, no staining; 0.1, 1–9% of cells stained; 0.5, 10–49% of cells stained; and 1 if >50% cells stained). The H score was determined by multiplying the intensity and the distribution scores, with a minimum score of 0 and a maximum score of 3. The median value of all H scores was set as the cutoff point to distinguish FXR1-high tumors from FXR1-low tumors in the present study. For PD-L1 staining, rabbit immunoglobulin G monoclonal antibody (clone SP142; Spring Bioscience, Pleasanton, CA) was used as a primary antibody. PD-L1 positivity was evaluated based on the intensity (Figure 2B) and distribution score as with FXR1. In the present study, a PD-L1 distribution rate of 50% or greater was defined as high, and all other cases were defined as low.
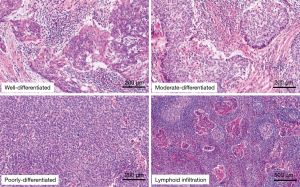
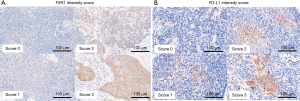
Statistical design and data analysis
The Mann-Whitney U test and Fisher’s exact test were used to compare the continuous and categorical variables between the groups, respectively. Overall survival (OS) and disease-free survival (DFS) were analyzed with the Kaplan–Meyer method, and the log-rank test was used to compare the survival curves. OS was defined as the time from surgery to death due to any cause. DFS was defined as the time from surgery to either the first disease relapse or death due to any cause. A univariate analysis of clinicopathological factors influencing OS and DFS using the log-rank test was performed. For all analyses, P value <0.05 were considered statistically significant. All statistical analyses were performed using the SPSS Statistics 25 software (IBM Corporation, Armonk, NY, USA).
Results
The clinical characteristics are summarized in Table 1. The patients included 25 males and 9 females, with a median age of 63 years (range, 38–79 years). The median PMI obtained using the preoperative CT images was 58.2% (interquartile range, 48.8–72.1%). The patients were divided into two groups: low PMI (<58.2%, n=17) and normal PMI (≥58.2%, n=17). The patients in the low PMI group were older than the patients in the normal PMI group (median age, 67 vs. 57 years; P=0.002). There were no significant differences in sex, BMI, serum albumin and CYFRA levels, FEV1.0, DLCO, and the pathological stage between the two groups. R0 resection was performed in 29 patients (75%), whereas R1 resection was performed in 5 patients (15%). Postoperative radiotherapy was administered to 11 patients (32%). No patient in the present study had autoimmune disorders, such as myasthenia gravis.
Table 1
Characteristics | Total (n=34) | Low PMI (n=17) | Normal PMI (n=17) | P value |
---|---|---|---|---|
PMI, %, median (range) | 58.2 (31.0–109.4) | 48.9 (31.0–58.1) | 71.6 (58.2–109.4) | <0.001 |
Age, years, median (range) | 63 (38–79) | 67 (54–79) | 57 (38–69) | 0.002 |
Sex | 0.50 | |||
Male | 25 (74%) | 13 (76%) | 12 (71%) | |
Female | 9 (26%) | 4 (24%) | 5 (29%) | |
BMI, kg/m2, median (range) | 22.7 (16.7–32.5) | 23.1 (18.9–26.2) | 22.0 (16.7–32.5) | 0.92 |
Serum albumin, g/dL, median (range) | 4.4 (3.6–4.9) | 4.3 (3.7–4.9) | 4.4 (3.6–4.9) | 0.38 |
CYFRA, median (range) | 2.7 (0.9–11.2) | 2.5 (1.1–9.3) | 2.9 (0.9–11.2) | 0.82 |
FEV1.0, % of predicted, median (range) | 93.7 (44.0–128.4) | 91.5 (44.0–128.4) | 97.9 (57.7–124.0) | 0.39 |
DLCO, % of predicted, median (range) | 106.4 (53.1–161.0) | 106.6 (53.1–161.0) | 103.0 (68.7–118.0) | 0.21 |
p-stage (Masaoka-Koga) | 0.47 | |||
I, II | 11 (32%) | 4 (24%) | 7 (41%) | |
III, IV | 23 (68%) | 13 (76%) | 10 (59%) | |
p-stage (TNM 8th) | 1 | |||
I, II | 15 (44%) | 7 (41%) | 8 (47%) | |
III, IV | 19 (56%) | 10 (59%) | 9 (53%) | |
Curability, R1 | 5 (15%) | 3 (18%) | 2 (12%) | 0.50 |
Postoperative radiotherapy, yes | 11 (32%) | 4 (24%) | 7 (41%) | 0.27 |
PMI, psoas muscle index; BMI, body mass index; CYFRA, cytokeratin fragment; FEV, forced expiratory volume; DLCO, diffusing capacity of the lung carbon monoxide; TNM, tumor-node-metastasis.
Histopathological features and IHC findings of TSQCC are summarized in Table 2. The TSQCC was classified as well differentiated in 7 patients, moderately differentiated in 23 patients, and poorly differentiated in 4 patients. Although fibrotic tissue was found around the tumor in many patients, abundant lymphoid infiltration around the tumor was observed in 8 patients (Figure 1). FXR1 was diffusely stained in the tumor cells’ cytoplasm. The FXR1 intensity score was high (2+–3+) in all but one patient. The distribution was also broad, with a median of 95% (range, 5–100%) (Table 2). The median H score for FXR1 was 3 (range, 0.1–3). Using this cutoff value, FXR1 expression was classified as high in 24 patients (71%) and low in 10 patients (29%). PD-L1 was diffusely stained in the tumor cells’ membrane, either partially or completely. The PD-L1 intensity score was also high (0–1+, 6%; 2+–3+, 94%) in many patients, and no staining was observed in two patients. The median PD-L1 distribution was 50% (range, 0–95%) (Table 2). PD-L1 expression was classified as high in 18 patients (53%) and low in 16 patients (47%). There were no significant differences regarding differentiation, lymphoid infiltration, and FXR1 and PD-L1 expression between the low and normal PMI groups (Figure 3).
Table 2
Characteristics | Total (n=34) | Low PMI (n=17) | Normal PMI (n=17) | P value |
---|---|---|---|---|
Differentiation | 0.33 | |||
Well | 7 | 5 | 2 | |
Moderate | 23 | 11 | 12 | |
Poor | 4 | 1 | 3 | |
Lymphoid infiltration | 1 | |||
Yes | 8 | 4 | 4 | |
No | 26 | 13 | 13 | |
FXR1 intensity | 0.66 | |||
0 | 0 | 0 | 0 | |
1+ | 1 | 1 | 0 | |
2+ | 5 | 3 | 2 | |
3+ | 28 | 13 | 15 | |
FXR1 distribution (%), median (range) | 95 (5–100) | 95 (5–100) | 90 (20–100) | 0.63 |
FXR1 H score, median (range) | 3 (0.1–3) | 3 (0.1–3) | 3 (1.5–3) | 0.54 |
FXR1 | 0.45 | |||
High | 24 (71%) | 11 (65%) | 13 (77%) | |
Low | 10 (29%) | 6 (35%) | 4 (24%) | |
PD-L1 intensity | 0.18 | |||
0 | 2 | 2 | 0 | |
1+ | 0 | 0 | 0 | |
2+ | 12 | 4 | 8 | |
3+ | 20 | 11 | 9 | |
PD-L1 distribution (%), median (range) | 50 (0–95) | 40 (0–90) | 50 (10–95) | 0.47 |
PD-L1 | 0.49 | |||
High | 18 (53%) | 8 (47%) | 10 (59%) | |
Low | 16 (47%) | 9 (53%) | 7 (41%) |
FXR1, fragile X-related 1; PD-L1, programmed cell death ligand 1; PMI, psoas muscle index.
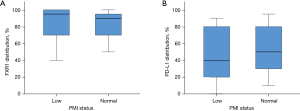
The median follow-up period for all cohorts was 60 months (range, 1–133 months). During the follow-up, tumor recurrence and death occurred in 13 patients (38%) and 6 patients (18%), respectively. Five patients died of the disease, and one patient died of other diseases. OS and DFS curves for all 34 patients are presented in Figure 4. The 5-year OS and 5-year DFS rates were 93% and 54%, respectively (Figure 4A,4B). PMI was associated with 5-year OS (low PMI vs. normal PMI: 86% vs. 100%; P=0.026) and was marginally associated with 5-year DFS (low PMI vs. normal PMI: 39% vs. 66%; P=0.09) (Figure 4C,4D). Table 3 shows the results of the univariate analysis with the log-rank test of the prognostic factors influencing OS and DFS. Although high PD-L1 expression represented a significantly better prognosis for OS, high PD-L1 expression was not statistically associated with DFS. In contrast, the male sex and earlier p-stage were significantly associated with better prognoses for DFS; however, these factors were not associated with OS. Other factors including CYFRA, differentiation, lymphoid infiltration, and FXR1 expression were not associated with OS or DFS. In a subgroup analysis stratified by p-stage (Masaoka-Koga), PMI status was a prognostic factor for 5-year OS in advanced TSQCC (low PMI vs. normal PMI: 82% vs. 100%; P=0.013), while PMI status was not associated with the prognosis for 5-year OS in early stage TSQCC (low PMI vs. normal PMI: 100% vs. 100%; P=0.81).
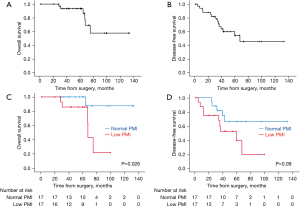
Table 3
Characteristics | Overall survival | Disease-free survival | |||
---|---|---|---|---|---|
5-year survival (%) | P value | 5-year survival (%) | P value | ||
Age (y), ≤65 vs. >65 | 94.7 vs. 90.9 | 0.32 | 47.9 vs. 63.5 | 0.89 | |
Sex, male vs. Female | 95.5 vs. 88.9 | 0.70 | 70.0 vs. 16.7 | 0.011 | |
CYFRA, ≤2.5 vs. >2.5 | 92.3 vs. 93.3 | 0.17 | 36.4 vs. 65.3 | 0.20 | |
PMI, low vs. Normal | 85.7 vs. 100.0 | 0.026 | 22.3 vs. 66.2 | 0.09 | |
Tumor size (mm), ≤50 vs. >50 | 100.0 vs. 88.2 | 0.43 | 64.5 vs. 44.7 | 0.10 | |
Lymph node metastasis, yes or no | 87.5 vs. 95.5 | 0.90 | 12.5 vs. 71.0 | 0.007 | |
Pathological stage (Masaoka-Koga), I–II vs. ≥III | 100.0 vs. 90.2 | 0.80 | 90.0 vs. 32.0 | 0.024 | |
Pathological stage (TNM 8th), I–II vs. ≥III | 100.0 vs. 88.9 | 0.69 | 80.8 vs. 34.8 | 0.017 | |
Curability, R0 vs. R1 | 92.1 vs. 100.0 | 0.19 | 64.4 vs. 20.0 | 0.18 | |
Postoperative radiotherapy, yes vs. No | 90.9 vs. 95.0 | 0.79 | 30.7 vs. 67.7 | 0.11 | |
Differentiation, well vs. Moderate/poor | 100.0 vs. 91.8 | 0.29 | 50.0 vs. 55.8 | 0.79 | |
Lymphoid infiltration, yes vs. No | 100.0 vs. 91.5 | 0.12 | 71.4 vs. 46.5 | 0.19 | |
FXR1, high vs. Low | 95.0 vs. 90.0 | 0.94 | 56.3 vs. 50.0 | 0.45 | |
PD-L1, high vs. Low | 100.0 vs. 85.1 | 0.018 | 58.4 vs. 40.4 | 0.43 |
CYFRA, cytokeratin fragment; PMI, psoas muscle index; FXR1, fragile X-related 1; PD-L1, programmed cell death ligand 1.
Discussion
In the present study, our findings suggest that PMI should be considered a potential prognostic factor in patients with TSQCC. Furthermore, FXR1 expression was widely observed regardless of PMI status. To the best of our knowledge, this study is the first to investigate the relationship between skeletal muscle mass and prognosis in patients with TSQCC. We also examined FXR1 expression in TSQCC using immunohistochemistry for the first time.
The number of studies on the prognosis for TCs has recently increased and includes large population-based studies (4). However, most current studies have included various subtypes in addition to TSQCC, such as basaloid carcinoma and mucoepidermoid carcinoma. Each histologic subtype has a special morphologic and oncologic behavior, which leads to discrepancies in the prognosis. Only a small number of studies on TSQCC have been published due to its low incidence, despite the fact that TSQCC is the most frequent subtype (20-23). Although sarcopenia and its surrogate marker, the PMI, have recently received considerable attention in the field of oncology as a prognostic factor for various types of cancers, there have been no studies till date assessing the relationship between TSQCC and PMI. We previously reported on the prognostic impact of skeletal muscle mass in lung squamous cell carcinoma but not lung adenocarcinoma, and we hypothesized that similar results would be obtained in patients with TSQCC (10). As expected, the present study demonstrated that PMI could be a prognostic factor for OS, although the results in terms of DFS were marginal. We have also shown that PMI is a strong prognostic factor, especially for patients with advanced TSQCC. Studies have investigated the relationship between inflammation/hypoalbuminemia and prognosis in TCs (24,25), and these factors might interact with PMI. However, we believe that skeletal muscle mass in patients with squamous cell carcinoma in the thymus as well as in the lung could reflect the tumor’s biological nature. However, the mechanism and interaction in the tumor microenvironment are as yet unknown. We therefore focused on the RNA-binding protein, FXR1, which is expressed in myocytes and plays an important role in myogenesis (11,13). In recent years, FXR1 expression has attracted attention as a prognostic factor in various malignant diseases including lung cancer; however, FXR1 expression in TSQCC has not been investigated. As observed in a previous report examining the significance of FXR1 in non-small cell lung cancer (13), we used immunohistochemistry in this study to evaluate the intensity and distribution of FXR1 expression. Consequently, the results showed strong intensity and a wide distribution regardless of PMI status in most cases. Although not directly indicating FXR1 as a prognostic factor in patients with TSQCC, this finding highlights the possibility of a relationship between the skeletal muscle mass and the microenvironment of TSQCC. Further evaluation of FXR1 might reveal this protein to be an interesting candidate target for cell-specific therapy.
The results of the present study have implications for the design of future clinical studies regarding TSQCC. It is expected that simply improving skeletal muscle mass could prolong long-term survival. It is important to accurately assess the quantity of skeletal muscle because it can be targeted for treatment before and after surgery with interventions including nutritional recommendations and rehabilitation (26,27). Exercise is generally known to be a non-pharmacological intervention that reduces fatigue and improves quality of life, lung function, muscle mass, physical performance, and the psychological status of patients with lung cancer (28,29). Furthermore, nutritional supplements, including branched-chain amino acids, vitamin D, whey protein, and hydroxymethylbutyrate-enriched milk, play an important role in preventing and improving sarcopenia (30). These interventions could therefore result in improved postoperative outcomes for patients with low PMI and TSQCC. Whether improvement in sarcopenia following these interventions can enhance the postoperative prognosis remains unclear due to the luck of the randomized control trial. Therefore, further studies are required to explore this possibility. Our finding that FXR1 is widely expressed in TSQCC makes it a potential new biomarker for molecular target therapy in the future. The role of skeletal muscle in the cancer microenvironment has not been well clarified, and further studies will help progress our understanding of the interaction between tumor cells and monocytes.
Our study has several limitations, the first of which is its small sample size. Our analysis might therefore have been too underpowered to identify differences in several values. In addition, we could not perform multivariate analyses due to the small number of patients and events. However, TC is a rare type of cancer, and studies limited to TSQCC in particular can be difficult to integrate in a single institution. Second, our retrospective study was performed at a single center with patients having a similar ethnic background. Data on FXR1 and PD-L1 were obtained from limited number of patients. Therefore, care should be taken in generalizing the interpretation of the results.
Conclusions
This study suggested that PMI was a potential prognostic factor in patients with TSQCC. In addition, FXR1 expression was widely observed regardless of PMI status in the patients with TSQCC. Skeletal muscle mass might play a role in the prognosis of TSQCC. Further studies on patients with TSQCC and an evaluation of FXR1 might reveal this protein to be an interesting candidate target for cell-specific therapy in the future.
Acknowledgments
Part of this work was presented at the Virtual 29th Meeting of the European Society of Thoracic Surgeons, June 20–22, 2021. The authors would like to thank Yuko Katayama from the Department of Pathology and Laboratory Medicine/Diagnostic Pathology of the Nagoya University Graduate School of Medicine for the technical assistance provided. The authors would also like to thank Enago (www.enago.jp) for the English language review.
Funding: None.
Footnote
Reporting Checklist: The authors have completed the STROBE reporting checklist. Available at https://jtd.amegroups.com/article/view/10.21037/jtd-22-385/rc
Data Sharing Statement: Available at https://jtd.amegroups.com/article/view/10.21037/jtd-22-385/dss
Peer Review File: Available at https://jtd.amegroups.com/article/view/10.21037/jtd-22-385/prf
Conflicts of Interest: All authors have completed the ICMJE uniform disclosure form (available at https://jtd.amegroups.com/article/view/10.21037/jtd-22-385/coif). TFCY serves as an unpaid editorial board member of Journal of Thoracic Disease from April 2022 to March 2024. The other authors have no conflicts of interest to declare.
Ethical Statement: The authors are accountable for all aspects of the work in ensuring that questions related to the accuracy or integrity of any part of the work are appropriately investigated and resolved. The study was approved by the Institutional Review Board of Nagoya University Hospital (approval No. 2021-0236) and conducted in accordance with the Declaration of Helsinki (as revised in 2013). Individual consent for this retrospective analysis was waived.
Open Access Statement: This is an Open Access article distributed in accordance with the Creative Commons Attribution-NonCommercial-NoDerivs 4.0 International License (CC BY-NC-ND 4.0), which permits the non-commercial replication and distribution of the article with the strict proviso that no changes or edits are made and the original work is properly cited (including links to both the formal publication through the relevant DOI and the license). See: https://creativecommons.org/licenses/by-nc-nd/4.0/.
References
- Ruffini E, Detterbeck F, Van Raemdonck D, et al. Thymic carcinoma: a cohort study of patients from the European society of thoracic surgeons database. J Thorac Oncol 2014;9:541-8. [Crossref] [PubMed]
- Kondo K, Monden Y. Therapy for thymic epithelial tumors: a clinical study of 1,320 patients from Japan. Ann Thorac Surg 2003;76:878-84; discussion 884-5. [Crossref] [PubMed]
- WHO Classification of Tumours Editorial Board. Thoracic tumours. Lyon: IARC, 2021.
- Hishida T, Nomura S, Yano M, et al. Long-term outcome and prognostic factors of surgically treated thymic carcinoma: results of 306 cases from a Japanese Nationwide Database Study. Eur J Cardiothorac Surg 2016;49:835-41. [Crossref] [PubMed]
- Weissferdt A, Fujimoto J, Kalhor N, et al. Expression of PD-1 and PD-L1 in thymic epithelial neoplasms. Mod Pathol 2017;30:826-33. [Crossref] [PubMed]
- Giaccone G, Kim C, Thompson J, et al. Pembrolizumab in patients with thymic carcinoma: a single-arm, single-centre, phase 2 study. Lancet Oncol 2018;19:347-55. [Crossref] [PubMed]
- Funaki S, Shintani Y, Fukui E, et al. The prognostic impact of programmed cell death 1 and its ligand and the correlation with epithelial-mesenchymal transition in thymic carcinoma. Cancer Med 2019;8:216-26. [Crossref] [PubMed]
- Park SY, Yoon JK, Lee SJ, et al. Prognostic value of preoperative total psoas muscle area on long-term outcome in surgically treated oesophageal cancer patients. Interact Cardiovasc Thorac Surg 2017;24:13-9. [Crossref] [PubMed]
- Sun C, Anraku M, Karasaki T, et al. Low truncal muscle area on chest computed tomography: a poor prognostic factor for the cure of early-stage non-small-cell lung cancer. Eur J Cardiothorac Surg 2019;55:414-20. [Crossref] [PubMed]
- Ozeki N, Kawaguchi K, Fukui T, et al. Psoas muscle mass in patients undergoing lung cancer surgery: a prognostic difference between squamous cell carcinoma and adenocarcinoma. Int J Clin Oncol 2020;25:876-84. [Crossref] [PubMed]
- Siomi MC, Siomi H, Sauer WH, et al. FXR1, an autosomal homolog of the fragile X mental retardation gene. EMBO J 1995;14:2401-8. [Crossref] [PubMed]
- Deng M, Wang N, Li Z, et al. FXR1 can bind with the CFIm25/CFIm68 complex and promote the progression of urothelial carcinoma of the bladder by stabilizing TRAF1 mRNA. Cell Death Dis 2022;13:170. [Crossref] [PubMed]
- Qian J, Hassanein M, Hoeksema MD, et al. The RNA binding protein FXR1 is a new driver in the 3q26-29 amplicon and predicts poor prognosis in human cancers. Proc Natl Acad Sci U S A 2015;112:3469-74. [Crossref] [PubMed]
- Qian J, Chen H, Ji X, et al. A 3q gene signature associated with triple negative breast cancer organ specific metastasis and response to neoadjuvant chemotherapy. Sci Rep 2017;7:45828. [Crossref] [PubMed]
- Meng Y, Li X. Expression and Prognosis Analysis of SUMOylation Regulators in Oral Squamous Cell Carcinoma Based on High-Throughput Sequencing. Front Genet 2021;12:671392. [Crossref] [PubMed]
- Koga K, Matsuno Y, Noguchi M, et al. A review of 79 thymomas: modification of staging system and reappraisal of conventional division into invasive and non-invasive thymoma. Pathol Int 1994;44:359-67. [Crossref] [PubMed]
- Brierley JD, Gospodarowicz MK, Wittekiind C, eds. UICC, TNM Classification of Malignant Tumours. 8th ed. New York, NY: Wiley, 2017.
- Hamaguchi Y, Kaido T, Okumura S, et al. Proposal for new diagnostic criteria for low skeletal muscle mass based on computed tomography imaging in Asian adults. Nutrition 2016;32:1200-5. [Crossref] [PubMed]
- Hakiri S, Fukui T, Mori S, et al. Clinicopathologic Features of Thymoma With the Expression of Programmed Death Ligand 1. Ann Thorac Surg 2019;107:418-24. [Crossref] [PubMed]
- Gao L, Wang C, Liu M, et al. Adjuvant chemotherapy improves survival outcomes after complete resection of thymic squamous cell carcinoma: a retrospective study of 116 patients. Interact Cardiovasc Thorac Surg 2021;33:550-6. [Crossref] [PubMed]
- Wu J, Wang Z, Jing C, et al. The incidence and prognosis of thymic squamous cell carcinoma: A Surveillance, Epidemiology, and End Results Program population-based study. Medicine (Baltimore) 2021;100:e25331. [Crossref] [PubMed]
- Yang X, Zhao K, Li C, et al. Thymic Squamous Cell Carcinoma: A Population-Based Surveillance, Epidemiology, and End Result Analysis. Front Oncol 2020;10:592023. [Crossref] [PubMed]
- Zhao Y, Zhao H, Hu D, et al. Surgical treatment and prognosis of thymic squamous cell carcinoma: a retrospective analysis of 105 cases. Ann Thorac Surg 2013;96:1019-24. [Crossref] [PubMed]
- Okuma Y, Ko R, Shukuya T, et al. Prognostic factors for patients with metastatic or recurrent thymic carcinoma receiving palliative-intent chemotherapy. Lung Cancer 2020;148:122-8. [Crossref] [PubMed]
- Yuan ZY, Gao SG, Mu JW, et al. Prognostic value of preoperative neutrophil-lymphocyte ratio is superior to platelet-lymphocyte ratio for survival in patients who underwent complete resection of thymic carcinoma. J Thorac Dis 2016;8:1487-96. [Crossref] [PubMed]
- Rosero ID, Ramírez-Vélez R, Lucia A, et al. Systematic Review and Meta-Analysis of Randomized, Controlled Trials on Preoperative Physical Exercise Interventions in Patients with Non-Small-Cell Lung Cancer. Cancers (Basel) 2019;11:944. [Crossref] [PubMed]
- Bauer J, Morley JE, Schols AMWJ, et al. Sarcopenia: A Time for Action. An SCWD Position Paper. J Cachexia Sarcopenia Muscle 2019;10:956-61. [Crossref] [PubMed]
- Avancini A, Sartori G, Gkountakos A, et al. Physical Activity and Exercise in Lung Cancer Care: Will Promises Be Fulfilled? Oncologist 2020;25:e555-69. [Crossref] [PubMed]
- Cavalheri V, Granger CL. Exercise training as part of lung cancer therapy. Respirology 2020;25:80-7. [Crossref] [PubMed]
- Chen LK, Woo J, Assantachai P, et al. Asian Working Group for Sarcopenia: 2019 Consensus Update on Sarcopenia Diagnosis and Treatment. J Am Med Dir Assoc 2020;21:300-307.e2. [Crossref] [PubMed]