Vessel heterogeneity of TIMI frame count and its relation to P-wave dispersion in patients with coronary slow flow
Introduction
Coronary slow flow (CSF) phenomenon, which is characterized by delayed coronary opacification in the absence of obstructive epicardial coronary artery disease, is a relatively common finding in patients undergoing routine coronary angiography and is often associated with chest pain (1,2). In some patients with CSF, blood flow may be heterogeneously distributed in the three coronary arteries, suggesting the heterogeneously distributed microvascular dysfunction in the myocardium (2-4). However, the vessel heterogeneity of Thrombolysis in Myocardial Infarction (TIMI) frame count (TFC) has not been fully elucidated.
P-wave dispersion (PWD) is defined as the difference between the longest and the shortest P-wave duration recorded from multiple different surface electrocardiogram (ECG) leads, and it has been reported to be associated with inhomogeneous and discontinuous propagation of sinus impulses (5), which are well known electrophysiologic characteristics of the atrium prone to fibrillation (5,6). Although it is plausible to hypothesize that PWD might be associated with the heterogeneously distributed microvascular dysfunction, and previous studies have demonstrated the abnormal PWD in patients with CSF (5,7), the correlation between TFC heterogeneity and PWD has never been evaluated.
In this study, we aimed to investigate the vessel heterogeneity of TFC in the three coronary arteries, and its relation to PWD, in patients with CSF and otherwise normal coronary arteries.
Materials and methods
Patient population and study protocol
The present study was conducted in the Department of Cardiology, the First Affiliated Hospital of Sun Yat-sen University. We enrolled 72 consecutive patients with angiographically documented CSF and 66 age- and gender-matched control subjects. All of the subjects had chest pain and were referred to our catheterization laboratory for coronary angiography, which showed normal epicardial coronary arteries and left ventricular systolic function. Diagnosis of CSF was based on TFC. Subjects with any of the following were excluded: valvular or congenital heart disease, atrial fibrillation (AF) or other arrhythmia that would interfere with ECG analysis, left ventricular hypertrophy, myocardial or pericardial disease, chronic obstructive pulmonary disease, or electrolyte abnormalities. Subjects taking antiarrhythmic, anti-ischemic, β-blocker, or calcium-channel blocker medications were also excluded from the study. Within 3 days before coronary angiography, standard 12-lead ECG was obtained.
The hospital Ethics Committee approved the study protocol, and each subject gave written informed consent to the study. This study (Prediction Value of Ecg on Coronary Slow-flow, PECS) is registered on Clinicaltrials.gov (NCT 02650557).
Coronary angiography and documentation of TFC
In all study participants, selective coronary angiography was performed using standard Judkins technique. Coronary arteries were visualized in left and right oblique planes with cranial and caudal angulations. Left ventriculography was performed in left and right anterior oblique views. Injection of contrast medium (Iopromide, Ultravist-370; Schering AG, Berlin, Germany) was carried out by an automatic injector, at a speed of 5 mL/s for left coronary artery and 3 mL/s for right coronary artery, and all angiograms were filmed at a speed of 30 frames/s.
Coronary flow was quantified objectively by two independent observers, who were blinded to the clinical details of the individual participants, using the TFC method, described by Gibson et al. (4,8). The first frame was defined as the frame in which concentrated dye occupies the full width of the proximal coronary artery lumen, touching both borders of the lumen, and indicates forward motion down the artery. The final frame counted is that in which the contrast first reaches the distal predefined landmark branch without the necessity for full opacification. Standard distal coronary landmarks are the distal bifurcation for the left anterior descending coronary artery (LAD), commonly referred as the “pitchfork” or “whale’s tail”, the distal bifurcation of the segment with the longest total distance for the left circumflex coronary artery (LCX), and the first branch of the posterolateral artery for the right coronary artery (RCA).
As the LAD is usually longer than the other major coronary arteries, the LAD frame count was divided by 1.7 to derive the corrected TFC as described earlier (8,9). The mean TFC for each participant was calculated by dividing the sum of the TFC of LAD (corrected), LCX and RCA by 3. Vessel heterogeneity of TFC in an individual patient was evaluated as coefficient of variation (CV), calculated by standard deviation of mean TFC of the three coronary arteries/mean TFC×100 for each patient.
All participants with a corrected TFC greater than 27 (two standard deviations from the normal published range) in at least one of the three epicardial coronary arteries were accepted as having CSF, while those whose TFC fell within the standard deviation of the published normal range were considered to have normal coronary flow (8).
Electrocardiography and PWD measurement
All standard 12-lead ECG were obtained at rest, simultaneously using a recorder (Hewlett Packard, Pagewriter) set at a 50-mm/s paper speed and 20 mm/mV amplitude with standard lead positions.
ECGs were manually measured by two blinded cardiologists having no information about the patients. The beginning of the P-wave was defined as the point where the initial deflection of the P-wave crossed the isoelectric line, and the end of the P-wave was defined as the point where the final deflection of the P-wave crossed the isoelectric line. The mean P-wave duration for at least three complexes was calculated in each lead. The difference between maximum and minimum P-wave duration (Pmax and Pmin) was calculated from the 12-lead ECG and defined as PWD. Intra-observer and interobserver coefficients of variation for P-wave variables were less than 5% and non-significant.
Statistical analysis
The statistical analysis was performed using SPSS 12.0 software. Data were expressed as mean ± SD unless otherwise specified. Continuous variables between groups were compared by Student’s t-test. Proportions were compared by the Fisher exact test when the expected frequency was <5, otherwise the chi-square test was applied. Pearson’s correlation analysis was used to test univariate relations. The κ statistic was used to calculate the magnitude and statistical significance of interobserver agreement between the interpretations of the angiography. Significance was considered to be achieved for two-tailed P values <0.05.
Results
Study population and clinical demographics
Patients’ clinical demographics in the CSF and control groups are indicated in Table 1. The two groups were similar for age, gender, cardiovascular risk factors, blood pressure, heart rate, left atrial diameter and left ventricular function.
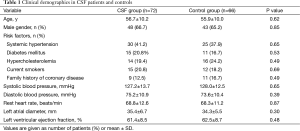
Full table
TFC and P-wave parameters
Comparisons of TFC and P-wave parameters between CSF patients and controls are presented in Table 2. The mean TFC and CV were both significantly higher in CSF patients than in controls (P<0.001 for both comparisons). The Pmax and PWD were found to be significantly higher in CSF patients than in controls (P<0.001 for both comparisons). In patients with CSF, TFC was heterogeneously distributed in the three coronary arteries, presenting with substantial variability.
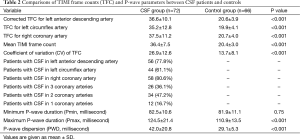
Full table
Correlations between P-wave parameters and TFC in CSF patients
Correlations between P-wave parameters and TFC data in CSF patients are presented in Table 3. P-wave parameters were not correlated to TFC for left anterior descending artery or TFC for right coronary artery, whereas Pmax and PWD were statistically correlated to TFC for left circumflex artery with a low P value.
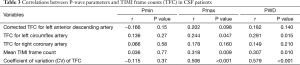
Full table
Both Pmax and PWD were mildly correlated to mean TFC, and were more significantly correlated to CV (Figure 1).
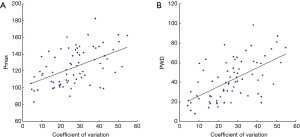
Discussion
This is the first study undertaken to investigate the vessel heterogeneity of coronary flow in the three coronary arteries, and its relation to P-wave duration and dispersion, in patients with CSF and otherwise normal coronary arteries. Our results indicated that CSF is not a phenomenon with homogeneous flow distribution in the three epicardial coronary arteries, suggesting that microvascular dysfunction is distributed heterogeneously in the different artery territories in this setting. Moreover, this vessel heterogeneity of blood flow was found to be correlated to P-wave duration and dispersion, a novel index predicting AF (10).
CSF phenomenon has been well recognized over the last three decades and was frequently observed in routine coronary angiography. Although the exact pathophysiological mechanisms still remain uncertain, CSF phenomenon is now recognized as a coronary microvascular disorder (11). Microvascular dysfunction is often distributed heterogeneously in the myocardium (12,13), and, in at least part of the patients with CSF, blood flow is not homogeneously distributed in the three coronary arteries (1-3). Although CSF is commonly diagnosed by simultaneously assessing TFC in the three coronary arteries, the vessel heterogeneity of TFC remains to be further elucidated. As flow reserve in a single coronary artery is usually used to assess the effect of some treatment (4,14), it is critically important to fully elucidate the vessel heterogeneity.
PWD, as well as Pmax, has been used to evaluate the intra-atrial and interatrial conduction times and the inhomogeneous propagation of sinus impulses, which has proven to be a sensitive and specific ECG predictor of AF in the various clinical settings (10,15). Since the intra-atrial and interatrial conduction times are apparently influenced by coronary blood flow and microcirculation in the atriums (15), it is plausible to speculate that PWD might be related to the impaired coronary flow, and to the heterogeneously distributed microvascular ischemia resulting from vessel heterogeneity in patients with CSF. In the present study, we found significantly higher Pmax and PWD in CSF patients than in controls, which are consistent with the previous reports (5,7). Moreover, we further found that in patients with CSF, both Pmax and PWD were mildly correlated to mean TFC, and were more significantly correlated to CV.
Although the clinical importance of this relationship remains unclear, considering the correlation between the presence of intra-atrial conduction abnormalities and the induction of paroxysmal AF (16), and our previous findings that AF patients have significantly higher TFC for all three coronary vessels compared to the control subjects (9), perhaps we can hypothesize that patients with CSF, especially those with substantial vessel heterogeneity in TFC, might be prone to AF. Prospective, long-term follow-up studies are warranted to confirm whether this vessel heterogeneity in TFC could translate into an increased incidence of AF in patients with CSF.
Study limitations
Our study was an observational assessment of daily clinical practice concerning CSF patients referred for diagnostic coronary angiography due to presence of angina or angina-like chest discomfort, bringing about important limitations. First of all, we did not further evaluate coronary physiology by using invasive measurements such as thermodilution method. The second limitation was that automated ECG measurements were not available and manual calculation of P-wave measurements may be of limited accuracy (17). However, several studies have demonstrated a low error of the measurement of PWD on paper printed ECGs (16,18), and the accuracy and reproducibility was acceptable in the present study, in which intra-observer and interobserver coefficients of variation for P-wave variables were less than 8% and statistically non-significant. Finally, we did not directly investigate the association of PWD and vessel heterogeneity in TFC with clinical outcomes and new onset AF in patients with CSF.
Conclusions
Coronary flow is heterogeneously distributed in three coronary arteries, and variability of TFC is increased in patients with CSF. The vessel heterogeneity in coronary flow might be intimately associated with PWD.
Acknowledgements
The authors thank the assistant of the statistical analysis from the Yilong Education, Inc., Sun Yat-sen University.
Funding: This study was supported by the grant from Guangdong Science and Technology Foundation (No. 2009B080701010, 2014A020212139) and Natural Science Foundation of Guangdong Province (No. 2015A030313160). The funders had no role in study design, data collection and analysis, decision to publish, or preparation of the manuscript.
Footnote
Conflicts of Interest: The authors have no conflicts of interest to declare.
References
- Beltrame JF. Defining the coronary slow flow phenomenon. Circ J 2012;76:818-20. [Crossref] [PubMed]
- Hawkins BM, Stavrakis S, Rousan TA, et al. Coronary slow flow--prevalence and clinical correlations. Circ J 2012;76:936-42. [Crossref] [PubMed]
- Luo C, Liu D, Wu G, et al. Effect of enhanced external counterpulsation on coronary slow flow and its relation with endothelial function and inflammation: a mid-term follow-up study. Cardiology 2012;122:260-8. [Crossref] [PubMed]
- Luo C, Wang L, Feng C, et al. Predictive value of coronary blood flow for future cardiovascular events in patients with atrial fibrillation. Int J Cardiol 2014;177:545-7. [Crossref] [PubMed]
- Dogan SM, Yildirim N, Gursurer M, et al. P-wave duration and dispersion in patients with coronary slow flow and its relationship with Thrombolysis in Myocardial Infarction frame count. J Electrocardiol 2008;41:55-9. [Crossref] [PubMed]
- Dilaveris PE, Gialafos EJ, Sideris SK, et al. Simple electrocardiographic markers for the prediction of paroxysmal idiopathic atrial fibrillation. Am Heart J 1998;135:733-8. [Crossref] [PubMed]
- Turkmen M, Barutcu I, Esen AM, et al. Effect of slow coronary flow on P-wave duration and dispersion. Angiology 2007;58:408-12. [Crossref] [PubMed]
- Gibson CM, Cannon CP, Daley WL, et al. TIMI frame count: a quantitative method of assessing coronary artery flow. Circulation 1996;93:879-88. [Crossref] [PubMed]
- Luo C, Wu X, Huang Z, et al. Documentation of impaired coronary blood flow by TIMI frame count method in patients with atrial fibrillation. Int J Cardiol 2013;167:1176-80. [Crossref] [PubMed]
- Dilaveris PE, Gialafos JE. P-wave dispersion: a novel predictor of paroxysmal atrial fibrillation. Ann Noninvasive Electrocardiol 2001;6:159-65. [Crossref] [PubMed]
- Beltrame JF, Limaye SB, Horowitz JD. The coronary slow flow phenomenon--a new coronary microvascular disorder. Cardiology 2002;97:197-202. [Crossref] [PubMed]
- Marroquin OC, Holubkov R, Edmundowicz D, et al. Heterogeneity of microvascular dysfunction in women with chest pain not attributable to coronary artery disease: implications for clinical practice. Am Heart J 2003;145:628-35. [Crossref] [PubMed]
- Johnson NP, Gould KL. Clinical evaluation of a new concept: resting myocardial perfusion heterogeneity quantified by markovian analysis of PET identifies coronary microvascular dysfunction and early atherosclerosis in 1,034 subjects. J Nucl Med 2005;46:1427-37. [PubMed]
- Caliskan M, Erdogan D, Gullu H, et al. Effects of atorvastatin on coronary flow reserve in patients with slow coronary flow. Clin Cardiol 2007;30:475-9. [Crossref] [PubMed]
- Altun A, Erdogan O, Tatli E, et al. Increased P wave dispersion: a new finding in patients with syndrome X. Can J Cardiol 2002;18:1207-10. [PubMed]
- Aytemir K, Ozer N, Atalar E, et al. P wave dispersion on 12-lead electrocardiography in patients with paroxysmal atrial fibrillation. Pacing Clin Electrophysiol 2000;23:1109-12. [Crossref] [PubMed]
- Dilaveris PE, Gialafos JE. P-wave duration and dispersion analysis: methodological considerations. Circulation 2001;103:E111-1. [Crossref] [PubMed]
- Dilaveris P, Batchvarov V, et al. Comparison of different methods for manual P wave duration measurement in 12-lead electrocardiograms. Pacing Clin Electrophysiol 1999;22:1532-8. [Crossref] [PubMed]