Reference values for resistance and compliance based on the single occlusion technique in healthy infants from Southeast China
Introduction
Respiratory disease is a major cause of morbidity and mortality in infants and imposes a significant impact on health care. With technical advance in respiratory detection, infant pulmonary function testing has become an important noninvasive method for assessing respiratory pathophysiology (1). It is critical to measure respiratory function for the treatment and study of pediatric lung disease (2). Unlike older children, infants cannot perform routine pulmonary function tests (such as forced expiratory volume at one second). Therefore, tidal breathing is the main pulmonary function testing method used in infants.
The single occlusion technique (SOT) is a noninvasive technique for measuring respiratory compliance (Crs) and resistance (Rrs). The SOT is an objective and easily applicable technique for assessing lung function in infants. It was first proposed by Olinsky et al. and later improved by Katier et al. and Gappa et al. (3,4). Crs and Rrs are two of the most widely used parameters in infant pulmonary function testing. The current guidelines describe the equipment, signal processing, data handling, and control, as well as the acceptance criteria for assessing the SOT in infants. However, in China, normal reference values are lacking for resistance and compliance using the SOT in infants; therefore, normal reference values need to be determined for the evaluation of respiratory development and for the diagnosis and treatment of respiratory diseases in infants.
Materials and methods
Study participants
The participants of this study were infants who visited the Children’s Hospital of FuDan University (Shanghai, China) from September 2013 to September 2014 for health examinations. For the purposes of our study, “infant” refers to a child aged <96 weeks. The infants were divided into four age groups: 1–24, 25–48, 49–72 and 73–96 weeks. The exclusion criteria included (I) gestational age of less than 37 weeks; (II) one or more symptoms of respiratory tract infections (e.g., fever ≥38 °C, cough, rhinorrhea, and vomiting) within 4 weeks; and (III) a history of congenital cardiopulmonary disease.
Measurements
Infant pulmonary function testing was performed using the Jaeger MasterScreen BabyBody system (Erich Jaeger, GmbH, Würzburg, Germany). The system was calibrated daily before the measurement. A silicone anesthesia facemask of the appropriate size covered the infants’ nose and mouth. The infants were sedated before the test. Oral chloral hydrate (100 mg/kg) was used for sedation. The infants were brought by their parents to the hospital on the day of the procedure after fasting for at least 4 hr, as per the arrangement of our conscious sedation unit, and had not slept for 3 hours before the study.
At the beginning of the test, the child breathed quietly and smoothly. When the infant’s end expiratory level was stable, the airway was briefly occluded at the end-inspiratory part of the tidal breath until an airway pressure plateau was observed, and the Hering-Breuer reflex was invoked to elicit relaxation of the respiratory system. The linear portion of the passive flow-volume curve was identified. From the intercepts on the flow and volume axes, the Crs and Rrs were calculated. For an overall understanding of the physiological status, flow-volume loops were collected to estimate the tidal volumes, the ratio of time to peak tidal expiratory flow over total expiratory time and the ratio of volume to peak expiratory flow over total expiratory volume. The data were required to meet guidelines of the European Respiratory Society (ERS)/American Thoracic Society (ATS) task force on infant lung function: (I) smooth expiration within 10% of the previous expiration; (II) the decay of the exhalation should be linear and smooth without glottic closure, early inspiration, or braking; (III) an occlusion plateau of 100 ms or longer; and (IV) standard deviation of the pressure plateau less than 0.01 kPa (4).
Informed consent was obtained from the parents or guardians of all children. This study was approved by the research ethics committee of Children’s Hospital of FuDan University (Shanghai, China). The clinical data in the study do not disclose the identity of the participants.
Statistical analysis
The statistical analyses were performed with the IBM SPSS Statistics version 20.0 (IBM Corporation, Armonk, NY, USA). Descriptive statistics were computed as the mean and standard deviation for continuous variables or as the median and 25th–75th percentiles in the event of a skewed distribution. For categorical variables, the descriptive statistics were computed as the absolute frequency and percent. The Mann-Whitney test was used for non-normally distributed continuous variables, and Student’s t test was used for normally distributed continuous variables. Regression analysis was used to develop the prediction equations of the parameters (i.e., Crs and Rrs). Age, weight, height, and body mass index (BMI) were a priori considered possible determinants. The associations of predictor variables with the dependent variables Crs and Rrs were examined by using single-variable models. The determinants that significantly improved (i.e., P<0.05) the fraction of explained variance of the dependent variables were determined through stepwise multivariable regression analysis. Interaction terms and several transformations of the predictors were composed and estimated in the regression models. The fit of the models was assessed with the check of normality of residuals. The coefficient of determination (R2), distribution of residuals, residual standard deviations, and homogeneity of variances were analyzed.
Results
Two hundred sixty-three infants underwent the SOT. The guardians of 19 infants refused to allow them to be sedated. The guardians of 17 infants refused to allow the children to undergo the test. Twenty-two infants failed to provide valid data. We succeeded in performing the measurements in 205 healthy infants from birth up to 96 weeks of life (112 boys, 93 girls). The baseline characteristics of the infants are displayed in Tables 1 and 2. All participants underwent lung function testing safely. The median age was 34 (range, 12–64) weeks, the mean length was 69.95±11.14 cm, and the mean weight was 8.34±2.79 kg. Length, weight, and BMI increased significantly with age (Table 1).
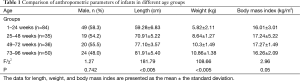
Full table
Lung function results of the different groups were compared (Table 2). The Rrs declined with age, whereas the Crs increased. The median Rrs was 5.04 kPa/L/s (3.73–6.82), and the mean Crs was 119.52±60.47 mL/kPa.
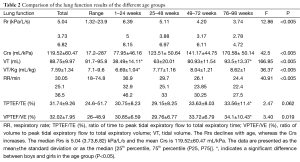
Full table
Univariate and multivariate regression equations for Crs and Rrs are presented in Tables 3 and 4, respectively. The Crs and Rrs in healthy infants from previous studies (5-10) are displayed in Table 5.
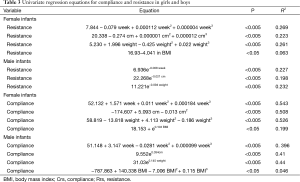
Full table
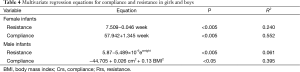
Full table
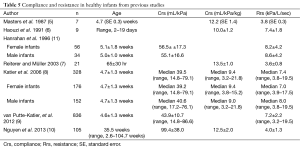
Full table
Discussion
Respiratory disease is a major cause of morbidity and mortality in infants and has a significant effect on health care (12-14). Like in adults, pulmonary function testing has been playing an important role in measurement of respiratory conditions in infants. Estimates of infant pulmonary function are being used increasingly in clinical and epidemiological studies (15). Guidelines regarding the methodology and equipment have been developed by the ATS and ERS Task Force (16), but there remains a shortage of reference equations for interpreting results. Infants cannot meet the demands of routine pulmonary function tests; therefore, the SOT can be used to reveal the condition of the respiratory physiology. Only small samples using this method to obtain reference values in infants have been described (8). To our knowledge, our study is the first to use the SOT to establish reference values for resistance and compliance in infants in Southeast China.
During the single breath occlusion technique, the airway is briefly occluded at the end of inspiration. The Hering-Breuer reflex leads to respiratory system relaxation during the occlusion. It is a relatively simple and useful method for the early detection of pulmonary diseases and for monitoring normal growth in infants. This simple technique allows measurement of the Crs and Rrs and the derivation of predictive equations for Crs and Rrs based on multiple regression analysis. In a study by Hanrahan et al. (11), passive respiratory mechanics were assessed using the SOT in 127 infants (55 boys and 72 girls) between 2 weeks and 18 months old. Respiratory compliance increased significantly with increasing infant length, whereas respiratory resistance (Rrs) declined (11). Their results were in line with those of Lanteri and Sly (17) and with the findings reported in the present study (Figures 1,2). Decreased Rrs was caused by the increased diameter of the airway and lung volume during childhood. Respiratory compliance increased gradually with the increase in the lung elastic fiber network. In our study, the dispersion degree of the resistance distribution decreased with the growth of the infants (Figure 1). There are two possible reasons. One is that, although nasal airway resistance contributes as much as 50% of the total airway resistance in newborns, the influence of nasal resistance on the total resistance decreases with the development of the upper airway. The other reason is that the variation in airway resistance is reduced by increased stability of the respiratory rate and respiratory rhythm with the development of the lung and airway (18).
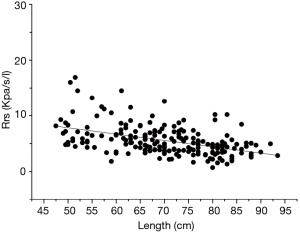
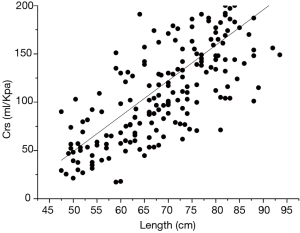
Sex differences in lung function are well recognized in children. Sex-specific normative equations are a standard feature of commercially available lung function equipment. Lanteri and Sly (17) published sex-specific equations for compliance and resistance, which were measured under general anesthesia in children aged 3 weeks to 15 years. In a longitudinal study by Turner et al. (19), lung function was initially lower in boys than in girls, but this difference reversed by 18 years of age. In a population-based birth cohort study by Belgrave et al. (20), boys had higher airway resistance than girls, and airway resistance increased significantly more in boys than in girls. However, in our study, we did not observe significant differences between boys and girls. These results were in line with those of studies by McKenzie et al. (21) and Zhang et al. (22). A larger sample size is needed to interpret this conflict in the future.
Reference equations for the SOT measured in infants are not widely available in the literature. The Crs and Rrs obtained in our study are equal to or somewhat lower than the values presented in previous studies. For Rrs, we found that age was an independent determinant in girls (explained 24% of variance), and weight was an independent determinant in boys (explained 6.1% of variance). For Crs, we found that age was independent determinant in girls (explained 55.2% of variance), and length and BMI were independent determinants in boys (explained 39.5% of variance). The mean values and prediction equations differs among previous studies, which is attributable to differences in sample size, age range of the participants, race, and other differences underlying the population (23).
The results of our study, as in most previous studies, were obtained with infants under sedation, which can occasionally result in mild respiratory depression with mild hypoxemia and a slight decrease in tidal volume (1). A comparison of infants during natural sleep with other groups of infants after sedation showed either an increased minute ventilation in the sedation group or a decreased respiratory rate in the sedation group (24). A study by Gelb et al. (25) found no evidence that sedation increased airway resistance. In the future, we will perform the SOT in infants during natural sleep and compare the differences between the results obtained with sedation and without sedation.
There are some limitations in the present study. First, like most other studies, our data were obtained from a cross-sectional survey, which limited our ability to explain short- or longer-term changes in infants. Further longitudinal studies are needed to track the secular trends, particularly during lung growth and development. Second, our results were obtained only from infants in Southeast China, so the sample was only weakly representative of all the infants in China. Further studies should compare our results with those from other areas in China. Third, we did not obtain other variables that affect lung function (such as birth length, birth weight, physical activity, parental lung function, socioeconomic status, and thoracic dimensions). More factors must be included in future studies to obtain more accurate results.
Our study established reference equations of resistance and compliance in Southeast Chinese infants aged 1–96 weeks old by using the SOT. Our results were based on recently collected data and accurately described the relationship between infant lung function and anthropometric variables. In the future, we will use the reference equations in epidemiological studies and in clinical practice.
Acknowledgements
None.
Footnote
Conflicts of Interest: The authors have no conflicts of interest to declare.
References
- Peterson-Carmichael SL, Rosenfeld M, Ascher SB, et al. Survey of clinical infant lung function testing practices. Pediatr Pulmonol 2014;49:126-31. [Crossref] [PubMed]
- Ren CL, Robinson P, Ranganathan S. Chloral hydrate sedation for infant pulmonary function testing. Pediatr Pulmonol 2014;49:1251-2. [Crossref] [PubMed]
- Katier N, Uiterwaal CS, de Jong BM, et al. Feasibility and variability of neonatal and infant lung function measurement using the single occlusion technique. Chest 2005;128:1822-9. [Crossref] [PubMed]
- Gappa M, Colin AA, Goetz I, et al. Passive respiratory mechanics: the occlusion techniques. Eur Respir J 2001;17:141-8. [Crossref] [PubMed]
- Masters IB, Seidenberg J, Hudson I, et al. Longitudinal study of lung mechanics in normal infants. Pediatr Pulmonol 1987;3:3-7. [Crossref] [PubMed]
- Haouzi P, Marchal F, Crance JP, et al. Respiratory mechanics in spontaneously breathing term and preterm neonates. Biol Neonate 1991;60:350-60. [Crossref] [PubMed]
- Reiterer F, Müller W. Assessment of the single-occlusion technique for measurements of respiratory mechanics and respiratory drive in healthy term neonates using a commercially available computerized pulmonary function testing system. Biol Neonate 2003;83:117-22. [Crossref] [PubMed]
- Katier N, Uiterwaal CS, de Jong BM, et al. Passive respiratory mechanics measured during natural sleep in healthy term neonates and infants up to 8 weeks of life. Pediatr Pulmonol 2006;41:1058-64. [Crossref] [PubMed]
- van Putte-Katier N, van der Gugten AC, Uiterwaal CS, et al. Early life lung function and respiratory outcome in the first year of life. Eur Respir J 2012;40:198-205. [Crossref] [PubMed]
- Nguyen TT, Hoo AF, Lum S, et al. New reference equations to improve interpretation of infant lung function. Pediatr Pulmonol 2013;48:370-80. [Crossref] [PubMed]
- Hanrahan JP, Brown RW, Carey VJ, et al. Passive respiratory mechanics in healthy infants. Effects of growth, gender, and smoking. Am J Respir Crit Care Med 1996;154:670-80. [Crossref] [PubMed]
- Sipahi EY. Experimental models of acute respiratory distress syndrome. J Transl Intern Med 2014;2:154-9. [Crossref]
- Yan J, Rao Q. Biomarkers in the diagnosis and prognostic assessment of acute respiratory distress syndrome. J Transl Intern Med 2014;2:160-3. [Crossref]
- Mohammad Y, Yassine F, Khadouj M. Comorbidities in 99 COPD patients: A case series from Syria. J Transl Intern Med 2015;3:167-70.
- Vogt B, Falkenberg C, Weiler N, et al. Pulmonary function testing in children and infants. Physiol Meas 2014;35:R59-90. [Crossref] [PubMed]
- Frey U, Stocks J, Coates A, et al. Specifications for equipment used for infant pulmonary function testing. ERS/ATS Task Force on Standards for Infant Respiratory Function Testing. European Respiratory Society/ American Thoracic Society. Eur Respir J 2000;16:731-40. [Crossref] [PubMed]
- Lanteri CJ, Sly PD. Changes in respiratory mechanics with age. J Appl Physiol (1985) 1993;74:369-78. [PubMed]
- Tabary A, Rassler B. Increased breathing resistance compromises the time course of rhythmical forearm movements—a pilot study. J Transl Intern Med 2015;3:161-6.
- Turner S, Fielding S, Mullane D, et al. A longitudinal study of lung function from 1 month to 18 years of age. Thorax 2014;69:1015-20. [Crossref] [PubMed]
- Belgrave DC, Buchan I, Bishop C, et al. Trajectories of lung function during childhood. Am J Respir Crit Care Med 2014;189:1101-9. [Crossref] [PubMed]
- McKenzie SA, Chan E, Dundas I, et al. Airway resistance measured by the interrupter technique: normative data for 2-10 year olds of three ethnicities. Arch Dis Child 2002;87:248-51. [Crossref] [PubMed]
- Zhang H, Xiao XM, Zheng S, et al. Study on the reference values of tidal flow-volume loop in 1002 normal Chinese children under 4 years old. The Journal of Clinical Pediatrics (Chinese) 2006;6:486-8.
- Quanjer PH, Stanojevic S, Cole TJ, et al. Multi-ethnic reference values for spirometry for the 3-95-yr age range: the global lung function 2012 equations. Eur Respir J 2012;40:1324-43. [Crossref] [PubMed]
- Rabbette PS, Dezateux CA, Fletcher ME, et al. Influence of sedation on the Hering-Breuer inflation reflex in healthy infants. Pediatr Pulmonol 1991;11:217-22. [Crossref] [PubMed]
- Gelb A, Southorn P, Rehder K, et al. Sedation and respiratory mechanics in man. Br J Anaesth 1983;55:809-15. [Crossref] [PubMed]