LncRNA KCNQ1OT1 enhances the radioresistance of lung squamous cell carcinoma by targeting the miR-491-5p/TPX2-RNF2 axis
Introduction
Lung cancer is still a common malignant tumor worldwide, with high morbidity and mortality (1). Non-small cell lung cancer (NSCLC) accounts for 80–85% of lung cancer cases (2). According to histopathological classification, NSCLC can be further divided into lung adenocarcinoma, lung squamous cell carcinoma (LUSC), and large cell carcinoma (3). Currently, more than half of newly diagnosed cancer patients require radiation therapy, which can be combined with surgery, chemotherapy, or molecular targeted therapies (4). Radiation therapy has played a vital role in the treatment of patients with metastatic disease (5). It has been proven that the biological basis of radiation therapy is the effect of ionizing radiation on biological cells (6). Radiation-sensitive patients means that when radiation doses are limited to levels that maximize therapeutic effects, reduce normal tissue cell death, prevent excessive inflammation, and preserve stem cell populations, the irradiated normal tissues of patients can resist permanent damage and do not exhibit clinically relevant adverse effects (7). However, according to statistics, 60–70% of NSCLC patients have received radiotherapy, while the radiotherapy effect and prognosis of NSCLC are still poor due to the constraints of radiation resistance and other factors (8). It is worth noting that the exact mechanism of LUSC cell radioresistance has not been elucidated. Therefore, how to improve the radioresistance of LUSC cells has become a new approach and strategy for LUSC treatment.
Long non-coding RNAs (lncRNAs) are a class of linear RNA molecules that have no transcriptional function or protein coding potential, and are mainly produced by RNA polymerase II/I (9). LncRNAs have been confirmed to regulate gene expression through genomic imprinting, transcriptional regulation, and chromatin modification (10,11). Studies have also demonstrated that lncRNAs can be involved in cell proliferation, apoptosis, differentiation, chromatin remodeling, migration, and invasion, among other processes (12,13). At present, lncRNAs have been proven to be crucial in almost all diseases, such as tumors (12), nervous system disorders (14), and cardiovascular system diseases (15). Therefore, lncRNAs can be applied as potential diagnostic markers and new drug targets for diseases. Recent research revealed that lncRNA KCNQ1OT1 was closely associated with the processes of multiple diseases including cancers (16-18), ischemia reperfusion (19), and diabetic nephropathy (20), among others. Interestingly, It was discovered that lncRNA KCNQ1OT1 was upregulated in lung cancer compared with the normal tissues and the upregulation of lncRNA KCNQ1OT1 reduced the survival rate of NSCLC patients (18). Besides, lncRNA KCNQ1OT1 has also been revealed to regulate the cisplatin resistance of cancer (21). However, the role and mechanism of lncRNA KCNQ1OT1 in radiation therapy for LUSC remain unclear.
MicroRNAs (miRNAs) are a class of small non-coding RNAs that can mediate mRNA transcription and degradation through binding to the complementary 3'-untranslated region (3'-UTR) (22,23). Research has shown that miRNAs are involved in cell proliferation, cell cycle, apoptosis, oncogenesis, and differentiation (24,25). In cancer tissues, miRNAs play the roles of oncogenes or tumor suppressor genes (26). In the diagnosis and therapy of clinical tumors, miRNAs can be used as early diagnostic indicators, effective prognostic indicators, and new treatment targets for lung cancer (27,28). Recent research has demonstrated that lncRNAs can competitively bind miRNAs with the targeted regulatory genes to induce cancer cell progression (29). However, the potential miRNAs and target genes of lncRNA KCNQ1OT1 have not been explored in radiation therapy for LUSC.
In this study, we generated radioresistant cells (SK-MES-1R and NCI-H226R cells) through X-ray irradiation, and further verified the correlation of lncRNA KCNQ1OT1 with the radioresistance of LUSC cells. Moreover, we disclosed the potential role and regulatory mechanism of lncRNA KCNQ1OT1 in the radioresistance of radioresistant LUSC cells. We present the following article in accordance with the MDAR reporting checklist (available at https://jtd.amegroups.com/article/view/10.21037/jtd-22-1261/rc).
Methods
Cell lines
LUSC cell lines (SK-MES-1 and NCI-H226) and HEK293 cells were purchased from American Type Culture Collection (Manassas, USA). SK-MES-1 cells were incubated in minimum Eagle’s medium (MEM, Gibco; Thermo Fisher Scientific, Inc.; Shanghai, China), NCI-H226 cells were grown in RPMI-1640 medium (Gibco), and HEK293 cells were cultured in Dulbecco’s modified Eagle’s medium (DMEM, Life Technologies; Thermo Fisher Scientific, Inc.; Shanghai, China). All the media were supplemented with 10% fetal bovine serum (FBS, HyClone; Logan, USA) and all cells were incubated at 37 ℃ with 5% CO2.
X-ray irradiation
SK-MES-1 and NCI-H226 cells were cultured to achieve 90% confluence and then exposed to 0, 2, 4, 6, 8, and 10 Gy 6 Mv X-rays at room temperature with a radiation distance of 100 cm and a radiation area of 10 cm × 10 cm for 4 h. After cell passage, the procedure was repeated. The surviving cells were the radioresistant cells (SK-MES-1R and NCI-H226R). After 8 passages, cells were used for the subsequent experiments.
Cell transfection
Control, lncRNA KCNQ1OT1 siRNAs (si#1 and si#2), miR-491-5p mimics, and anti-miR-491-5p were obtained from Shanghai Integrated Biotech Solutions Co., Ltd. (Shanghai, China). SK-MES-1R and NCI-H226R cells were transfected with above recombinants at a concentration of 40 nM using Lipofectamine 3000 (Invitrogen; Thermo Fisher Scientific, Inc.; Shanghai, China) in line with the experimental instructions.
Clonogenic assay
The 4 kinds of cell lines were inoculated in 6-well plates with 200, 200, 400, 800, 1,600, 2,000, and 4,000 cells per well, respectively. Then, cells were exposed to 0, 2, 4, 6, 8, and 10 Gy 6 Mv X-rays. After 14 days, 4% paraformaldehyde was applied to fix the cells and Wright-Giemsa (Shanghai yuan Mu Biotechnology Co., Ltd.; Shanghai, China) was adopted to stain the cells. Cell clones were observed and counted with at least 50 cells. Based on previous research (30), the surviving fraction was also counted.
RT-qPCR analysis
According to the kit instructions, total RNA was extracted by the TRIzol method (Invitrogen), and cDNA was obtained by reverse transcription using the BestarTM qPCR RT kit (DBI Bioscience, Shanghai, China). The levels of lncRNA KCNQ1OT1 and miR-491-5p in cells were examined by RT-qPCR analysis with SYBR green master mix (Thermo Fisher Scientific). Primer sequences are exhibited in Table 1.
Table 1
ID | Sequence (5'-3') |
---|---|
GAPDH | Forward: TGTTCGTCATGGGTGTGAAC |
GAPDH | Reverse: ATGGCATGGACTGTGGTCAT |
LncRNA KCNQ1OT1 | Reverse: GACCTGGCAGTCTCAAAAGC |
LncRNA KCNQ1OT1 | Forward: CACTGGGGCAGTCACCTAAT |
U6 | Forward: CTTCGGCAGCACATATAC |
U6 | Reverse: GAACGCTTCACGAATTTGC |
miR-491-5p | Forward: GGAGTGGGGAACCCTTCC |
miR-491-5p | Reverse: GTGCAGGGTCCGAGGT |
LncRNA, Long non-coding RNA; GAPDH, glyceraldehyde-3-phosphate dehydrogenase; RT-qPCR, real-time quantitative polymerase chain reaction.
MTT assay
The treated SK-MES-1R and NCI-H226R cells (4,000 cells/well) were administered in 96-well plates and cultured at 37 ℃ for 0, 12, 24, 48, and 72 h. At the set time point, 20 µL MTT reagent (cat. no. M5655) was added to each well and the cells were incubated for an additional 4 h. After dissolution with dimethyl sulfoxide (DMSO, cat. no. D8418), the 96-well plates were placed under an automatic microplate reader to detect the optical density (OD) at 490 nm.
Colony formation assay
After digestion and counting, the treated SK-MES-1R and NCI-H226R cells were seeded in 6-well plates with 400 cells/well. After incubation for 12 days, cells were fixed using methanol for 10 min and dyed with crystal violet for 10 min. After washing, the cells were air dried, and the number of clones was taken and counted.
EdU staining
Cell proliferation was monitored by the EdU assay kit (Life Technologies). EdU solution (10 µM) was added to the cells in 24-well plates, and cells were cultured for 2 h at 37 ℃. After fixation using 4% formaldehyde for 20 min, the EdU-stained cells were examined. Next, the cells were treated with Hoechst 33342 for 20 min, and the results were visualized under a fluorescence microscope (Olympus, Tokyo, Japan).
Flow cytometry
The treated SK-MES-1R and NCI-H226R cells were collected and washed using phosphate-buffered saline (PBS). The cell suspension was then added with Annexin-V-fluorescein isothiocyanate (FITC) and propidium iodide (PI) (BD Biosciences, San Jose, USA) for 15 min. Apoptotic cells were determined using a FACS Calibur Flow cytometer (BD Bioscience).
Western blot
The treated SK-MES-1R and NCI-H226R cells were harvested, and total proteins were extracted using RIPA buffer. The protein concentration was determined by the BCA method (Beyotime Biotechnology, China). Protein samples (40 µg) in each group were subjected to electrophoresis for 2 h and transmembrane treatment for 1 h, and then sealed in 5% non-fat milk for 2 h. Subsequently, the membranes were cultivated with primary antibodies at 4 ℃ overnight, followed by the cultivation with horseradish peroxidase (HRP)-conjugated secondary antibodies (1:1,000, cat. no. ab6802, Abcam, Shanghai, China) for 1 h. The immunochemical detection was conducted using the ECL system (Thermo Fisher Scientific). The primary antibodies were Bax (1:1,000, cat. no. 5023, Cell Signaling Technology, Shanghai, China), Bcl2 (1:1,000, cat. no. ab196495, Abcam), cleaved caspase-3 (1:1,000, cat. no. 9664, Cell Signaling Technology), Xenopus kinesin-like protein 2 (TPX2; 1:1,000, cat. no. ab32795, Abcam), RING finger protein 2 (RNF2; 1:1,000, cat. no. ab101273, Abcam), and β-actin (1:5,000, cat. no. ab179467, Abcam).
Anti-AGO2 radioimmunoprecipitation (RIP) assay
As reported by a previous study (31), we also used the Magna RIP RNA-Binding Protein Immunoprecipitation kit (Millipore, Billerica, USA) to conduct the anti-AGO2 RIP assay. Extracts of the treated SK-MES-1R and NCI-H226R cells in RIP buffer were incubated with normal rabbit IgG (Proteintech Group, Inc.; Wuhan, China) and AGO2 antibodies (Cell Signaling Technology), which were combined with magnetic beads. We isolated the immunoprecipitated RNAs and examined genes using RT-qPCR.
Luciferase assay
We constructed the wild-type and mutant lncRNA KCNQ1OT1, TPX2, and RNF2 with potential miR-491-5p binding sites using the pMIR-REPORT plasmids (Promega Biotechnology Co., Ltd.; Beijing, China). HEK293T cells (1×105 cells/well) were inoculated in a 24-well plate and co-transfected with luciferase plasmids, miR-491-5p mimics, and miRNA control for 48 h. Luciferase activity was confirmed using a dual luciferase reporter assay system (Promega).
Statistical analysis
Measurement data was presented as mean ± standard deviation (SD) from 3 replications. The statistical significance was confirmed using SPSS software 21.0 (SPSS Inc., Chicago, USA) with Student’s t-test. P<0.05 indicated a significant difference. All experiments were independently repeated in triplicate and all experimental data were biologically repeated in triplicate.
Results
LncRNA KCNQ1OT1 was highly expressed in radioresistant LUSC cells
To explore the possible relationship between LUSC cell radiosensitivity and lncRNA KCNQ1OT1, the parental LUSC cells (SK-MES-1 and NCI-H226) and the radioresistant LUSC cells (SK-MES-1R and NCI-H226R) were exposed to different levels of X-ray irradiation. The results from the clonogenic assay showed that SK-MES-1R and NCI-H226R cells displayed greater resistance to X-ray exposure than their parental cells (Figure 1A,1B). Subsequently, our data from RT-qPCR demonstrated that lncRNA KCNQ1OT1 expression was notably increased in SK-MES-1R and NCI-H226R cells relative to their respective parental cells (Figure 1C). Furthermore, we demonstrated that lncRNA KCNQ1OT1 was significantly expressed in the cytoplasm of SK-MES-1R and NCI-H226R cells, as well as in the nucleus (Figure 1D,1E). Consequently, we validated that lncRNA KCNQ1OT1 was prominently upregulated in the SK-MES-1R and NCI-H226R cells, especially in the nucleus.
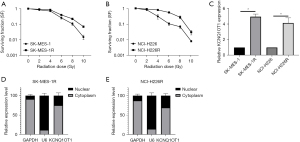
Knockdown of lncRNA KCNQ1OT1 significantly repressed the proliferation of radioresistant LUSC cells
To further elucidate the impact of lncRNA KCNQ1OT1 on radioresistant LUSC cells, lncRNA KCNQ1OT1 expression was knocked down by siRNAs in SK-MES-1R, NCI-H226R, and the parental cells. As presented in Figure 2A, lncRNA KCNQ1OT1 was significantly downregulated in the knockdown group versus the control group, indicating the effective transfection of siRNAs in each group. Next, cell proliferation was examined by conducting the MTT assay, colony formation assay, and EdU staining. The MTT results demonstrated that the proliferation capacities of SK-MES-1R and NCI-H226R cells were dramatically weakened in the knockdown group compared to the control group (Figure 2B,2C). Also, the experimental results of the colony formation assay showed that silencing lncRNA KCNQ1OT1 resulted in a significant reduction in the proliferation of SK-MES-1R and NCI-H226R cells (Figure 2D). Similarly, the results of EdU staining also revealed that the proliferation of SK-MES-1R and NCI-H226R cells could be significantly inhibited by lncRNA KCNQ1OT1 knockdown (Figure 2E). On the whole, we demonstrated that silencing lncRNA KCNQ1OT1 has a significant inhibitory effect on radioresistant LUSC cell proliferation.
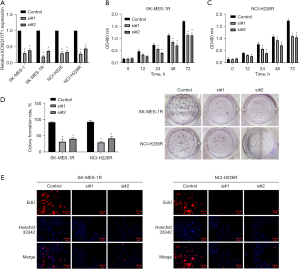
Silencing of lncRNA KCNQ1OT1 suppressed radioresistance and induced the apoptosis of radioresistant LUSC cells
Furthermore, we adopted MTT assay to assess the role of lncRNA KCNQ1OT1 silencing in the radioresistance of SK-MES-1R and NCI-H226R cells. The results showed that silencing of lncRNA KCNQ1OT1 significantly repressed cell growth in SK-MES-1R and NCI-H226R cells, and X-ray irradiation could also lead to a decrease in the survival rate of SK-MES-1R and NCI-H226R cells (Figure 3A,3B). Additionally, the flow cytometry data showed that silencing of lncRNA KCNQ1OT1 could significantly increase the number of apoptotic cells after treatment with 4 Gy X-ray irradiation (Figure 3C,3D). Meanwhile, we also found that in X-ray-treated SK-MES-1R and NCI-H226R cells, the levels of Bax and cleaved caspase-3 were dramatically elevated, and the level of Bcl2 was markedly lowered in the lncRNA KCNQ1OT1 silencing group compared to the control group (Figure 3E,3F). Overall, we uncovered that lncRNA KCNQ1OT1 knockdown markedly reduced the radioresistance of radioresistant SK-MES-1R and NCI-H226R cells.
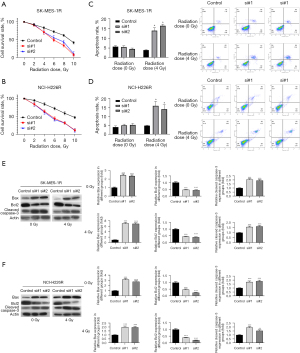
MiR-491-5p was a regulatory target of lncRNA KCNQ1OT1
More and more evidence has shown that lncRNAs may play the role of miRNA sponges, regulating the binding of miRNAs to the target mRNAs (32,33). We used a bioinformatics tool (LncBase Predicted v.2) to predict the target miRNAs of lncRNA KCNQ1OT1, and screened 4 potential target miRNAs including miR-491-5p, miR-133b, miR-15a, and miR-7. Firstly, we discovered that only miR-491-5p was prominently downregulated in SK-MES-1R and NCI-H226R cells compared with their respective parental cells (Figure 4A,4B). Secondly, we performed the anti-AGO2 RIP assay to monitor whether lncRNA KCNQ1OT1 could directly interact with these 4 miRNAs. The data indicated that lncRNA KCNQ1OT1 could be specifically enriched in miR-491-5p-overexpressed SK-MES-1R and NCI-H226R cells (Figure 4C,4D). In addition, we constructed the wild-type and mutant lncRNA KCNQ1OT1 plasmids, which were co-transfected into HEK-293 cells. The results uncovered that the luciferase activity was substantially reduced in HEK-293 cells with the co-transfection of miR-491-5p and wild-type lncRNA KCNQ1OT1, while the luciferase activity was not affected in the lncRNA KCNQ1OT1 mutant co-transfection group (Figure 4E). We also found that silencing of lncRNA KCNQ1OT1 could notably upregulate miR-491-5p in SK-MES-1R and NCI-H226R cells (Figure 4F). As a whole, miR-491-5p was an inhibitory target of lncRNA KCNQ1OT1.
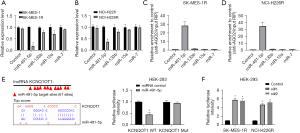
MiR-491-5p inhibited proliferation and downregulated TPX2 and RNF2 in radioresistant LUSC cells
We further predicted the target genes of miR-491-5p using bioinformatics analysis, and discovered that TPX2 and RNF2 might be the potential target genes of miR-491-5p. The luciferase assay showed that miR-491-5p reduced only the luciferase activity of wild-type TPX2, while the luciferase activity of mutant TPX2 was not responsive to miR-491-5p overexpression (Figure 5A). We also demonstrated that miR-491-5p significantly attenuated the luciferase activity of wild-type RNF2, but did not affect the luciferase activity of mutant RNF2 (Figure 5B). Furthermore, the colony formation assay elucidated that overexpression of miR-491-5p led to the inhibition of proliferation in SK-MES-1R and NCI-H226R cells (Figure 5C). Similarly, inhibition of miR-491-5p could result in the enhancement of proliferation in SK-MES-1R and NCI-H226R cells (Figure 5D). Western blotting analysis demonstrated that overexpression of miR-491-5p significantly lowered TPX2 and RNF2 expression, and inhibition of miR-491-5p markedly elevated TPX2 and RNF2 expression in SK-MES-1R and NCI-H226R cells (Figure 5E,5F). Overall, we demonstrated that miR-491-5p could significantly prevent proliferation and reduce TPX2 and RNF2 expression in SK-MES-1R and NCI-H226R cells.
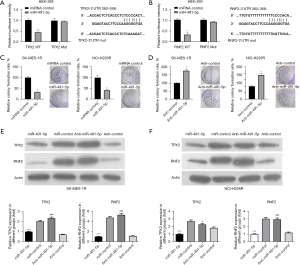
Knockdown of lncRNA KCNQ1OT1 markedly prevented radioresistant LUSC cell proliferation by targeting miR-491-5p to regulate TPX2 and RNF2
Subsequently, we performed rescue experiments to explore the impact of lncRNA KCNQ1OT1/miR-491-5p on the proliferation or TPX2 and RNF2 expression in radioresistant LUSC cells. LncRNA KCNQ1OT1 siRNAs and anti-miR-491-5p were adopted to transfect SK-MES-1R and NCI-H226R cells. The colony formation assay revealed that lncRNA KCNQ1OT1 knockdown dramatically repressed proliferation, and inhibition of miR-491-5p markedly accelerated proliferation. Meanwhile, co-transfection of lncRNA KCNQ1OT1 siRNAs and anti-miR-491-5p offset this effect in SK-MES-1R and NCI-H226R cells (Figure 6A). Western blot results demonstrated that lncRNA KCNQ1OT1 knockdown significantly downregulated TPX2 and RNF2 expression, inhibition of miR-491-5p notably upregulated TPX2 and RNF2 expression, and co-transfection of lncRNA KCNQ1OT1 siRNAs and anti-miR-491-5p further enhanced the expression of TPX2 and RNF2 in SK-MES-1R and NCI-H226R cells (Figure 6B). Collectively, these data demonstrated that lncRNA KCNQ1OT1 knockdown prevented the proliferation of SK-MES-1R and NCI-H226R cells by miR-491-5p to regulate TPX2 and RNF2.
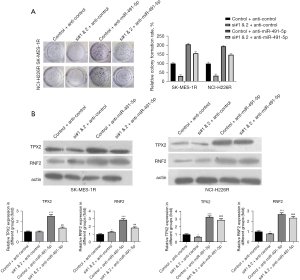
Discussion
Lung cancer has been considered to be one of the biggest contributors to cancer-related deaths worldwide (34). As a vital subtype of lung cancer, the incidence of LUSC is also increasing yearly (35). At present, radiotherapy has become one of the crucial methods of LUSC treatment because it can inhibit tumor growth and induce cell apoptosis. Increasing the radiosensitivity of tumor cells has become the most effective strategy for LUSC radiotherapy (36). However, the progression of LUSC is a multifactorial and multi-step process, and the carcinogenesis mechanism is still not very clear. At present, there is no effective biological target to increase radiosensitivity. In recent years, more and more evidence has confirmed that lncRNAs can participate in tumor progression through multiple pathways (37,38). Over the past decade, significant efforts have been made to bring ncRNA-based therapies to clinical use, some of which have received FDA approval. However, trial results to date have been contradictory, with some studies reporting effective results and others showing limited efficacy or toxicity (39,40). More important, studies have found that lncRNAs are involved in regulating the radiosensitivity of tumors (41,42). For instance, LINC02532 contributes to the radiosensitivity of clear cell renal cell carcinoma (43). LINC00958 could suppress radiosensitivity in colorectal cancer (44), and lncRNAs, such as PVT1 (45) and TUG1 (46), can also enhance radiosensitivity by promoting the apoptosis of tumor cells. Therefore, investigating the mechanism of lncRNAs in LUSC can lay the foundation for the improvement of radiosensitivity.
According to the literature, lncRNA KCNQ1OT1 has also been proven to be involved in the progression of multiple diseases, such as osteolysis (47), fracture healing (48), myocardial ischemia/reperfusion injury (49), atrial fibrillation (50), and diabetic cardiomyopathy (51). Furthermore, research has shown that lncRNA KCNQ1OT1 is also related to the progression of acute promyelocytic leukemia (52), ovarian cancer (17), bladder cancer (53), and NSCLC (18). Moreover, studies demonstrated that lncRNA KCNQ1OT1 was involved in oxaliplatin-resistant colon cancer (16), methotrexate-resistant colorectal cancer (54), and cisplatin-resistant tongue cancer (21). In our study, we established radioresistant cells (SK-MES-1R and NCI-H226R cells) through X-ray irradiation. LncRNA KCNQ1OT1 was notably upregulated in SK-MES-1R and NCI-H226R cells. Moreover, we verified that knockdown of lncRNA KCNQ1OT1 has a significant inhibitory effect on the proliferation and radioresistance of radioresistant LUSC cells. Knockdown of lncRNA KCNQ1OT1 also had a significant promotive effect on the apoptosis of radioresistant LUSC cells. Therefore, we demonstrated that lncRNA KCNQ1OT1 could dramatically enhance the radioresistance and induce the malignant behaviors of LUSC.
MiRNAs are a group of crucial regulatory factors in cancers, and are closely related to the progression of NSCLC (55). Studies have discovered that lncRNAs can serve as “sponges” of miRNAs to reduce miRNA abundance, thus alleviating the inhibition effect of miRNAs on the downstream target genes (56,57). Research has shown that the lncRNA/miRNA/mRNA axis can play vital roles in regulating the biological behaviors and radiosensitivity of various cancer cells, such as hepatocellular carcinoma (58), pancreatic cancer (59), lung adenocarcinoma (60), nasopharyngeal carcinoma (61), and ovarian cancer (62), among others. We also predicted the target miRNAs of lncRNA KCNQ1OT1 through a bioinformatics tool, and miR-491-5p, miR-133b, miR-15a, and miR-7 were screened as the potential target miRNAs of lncRNA KCNQ1OT1. Several studies have confirmed that miR-491-5p can function as a tumor suppressor in many types of cancers, such as osteosarcoma (63), colorectal cancer (64), gastric cancer (65), prostate cancer (66), nasopharyngeal carcinoma (67), and NSCLC (68). After experimental verification, we also discovered that miR-491-5p was a regulatory target of lncRNA KCNQ1OT1, and miR-491-5p could also be markedly upregulated by lncRNA KCNQ1OT1 knockdown.
Furthermore, through bioinformatics and experimental validation, we proved that TPX2 and RNF2 might be the potential target genes of miR-491-5p. As a microtubule-associated protein, TPX2 is essential for microtubule formation and can regulate many crucial biological processes (69,70). The aberrant expression of TPX2 has a vital relationship with the progression of human malignant tumors (71,72). RNF2, a member of the polycomb gene family, is a ubiquitin ligase with ring structure (73). Recent research has also confirmed that RNF2 is highly expressed in human malignant tumors, and is associated with the proliferation, invasion, and prognosis of tumors (74,75). Another study showed that silencing of RNF2 could promote the radiosensitivity of NSCLC (76). In our study, we also revealed that miR-491-5p could markedly downregulate TPX2 and RNF2 expression in radioresistant LUSC cells. In addition, knockdown of lncRNA KCNQ1OT1 could also markedly inhibit the proliferation of LUSC through miR-491-5p to regulate TPX2 and RNF2.
Conclusions
We demonstrated that lncRNA KCNQ1OT1 could markedly induce the radioresistance of LUSC by directly targeting miR-491-5p to reduce TPX2 and RNF2 expression. Therefore, we uncovered that the lncRNA KCNQ1OT1/miR-491-5p/TPX2 or RNF2 axis is correlated with the radioresistance of LUSC cells. In the future, this conclusion will be further validated in clinical and in vivo samples.
Acknowledgments
Funding: This work was supported by the Natural Science Foundation of Hebei Province (No. H2019206291).
Footnote
Reporting Checklist: The authors have completed the MDAR reporting checklist. Available at https://jtd.amegroups.com/article/view/10.21037/jtd-22-1261/rc
Data Sharing Statement: Available at https://jtd.amegroups.com/article/view/10.21037/jtd-22-1261/dss
Conflicts of Interest: All authors have completed the ICMJE uniform disclosure form (available at https://jtd.amegroups.com/article/view/10.21037/jtd-22-1261/coif). The authors have no conflicts of interest to declare.
Ethical Statement: The authors are accountable for all aspects of the work in ensuring that questions related to the accuracy or integrity of any part of the work are appropriately investigated and resolved.
Open Access Statement: This is an Open Access article distributed in accordance with the Creative Commons Attribution-NonCommercial-NoDerivs 4.0 International License (CC BY-NC-ND 4.0), which permits the non-commercial replication and distribution of the article with the strict proviso that no changes or edits are made and the original work is properly cited (including links to both the formal publication through the relevant DOI and the license). See: https://creativecommons.org/licenses/by-nc-nd/4.0/.
References
- Nooreldeen R, Bach H. Current and Future Development in Lung Cancer Diagnosis. Int J Mol Sci 2021;22:8661. [Crossref] [PubMed]
- Skřičková J, Kadlec B, Venclíček O. Non-small cell lung cancer. Vnitr Lek Winter;63:861-74.
- Akhurst T. Staging of Non-Small-Cell Lung Cancer. PET Clin 2018;13:1-10. [Crossref] [PubMed]
- Mesko S, Gomez D. Proton Therapy in Non-small Cell Lung Cancer. Curr Treat Options Oncol 2018;19:76. [Crossref] [PubMed]
- Miranda AF, Howard JM, McLaughlin M, et al. Metastasis-directed radiation therapy after radical cystectomy for bladder cancer. Urol Oncol 2021;39:790.e1-7. [Crossref] [PubMed]
- Kris MG, Gaspar LE, Chaft JE, et al. Adjuvant Systemic Therapy and Adjuvant Radiation Therapy for Stage I to IIIA Completely Resected Non-Small-Cell Lung Cancers: American Society of Clinical Oncology/Cancer Care Ontario Clinical Practice Guideline Update. J Clin Oncol 2017;35:2960-74. [Crossref] [PubMed]
- Habash M, Bohorquez LC, Kyriakou E, et al. Clinical and Functional Assays of Radiosensitivity and Radiation-Induced Second Cancer. Cancers (Basel) 2017;9:147. [Crossref] [PubMed]
- Timmerman RD, Hu C, Michalski JM, et al. Long-term Results of Stereotactic Body Radiation Therapy in Medically Inoperable Stage I Non-Small Cell Lung Cancer. JAMA Oncol 2018;4:1287-8. [Crossref] [PubMed]
- Dahariya S, Paddibhatla I, Kumar S, et al. Long non-coding RNA: Classification, biogenesis and functions in blood cells. Mol Immunol 2019;112:82-92. [Crossref] [PubMed]
- Li LJ, Leng RX, Fan YG, et al. Translation of noncoding RNAs: Focus on lncRNAs, pri-miRNAs, and circRNAs. Exp Cell Res 2017;361:1-8. [Crossref] [PubMed]
- Wu T, Du Y. LncRNAs: From Basic Research to Medical Application. Int J Biol Sci 2017;13:295-307. [Crossref] [PubMed]
- Mitobe Y, Takayama KI, Horie-Inoue K, et al. Prostate cancer-associated lncRNAs. Cancer Lett 2018;418:159-66. [Crossref] [PubMed]
- Ashrafizadeh M, Rabiee N, Kumar AP, et al. Long noncoding RNAs (lncRNAs) in pancreatic cancer progression. Drug Discov Today 2022;27:2181-98. [Crossref] [PubMed]
- Chen Z, Wu H, Zhang M. Long non-coding RNA: An underlying bridge linking neuroinflammation and central nervous system diseases. Neurochem Int 2021;148:105101. [Crossref] [PubMed]
- Correia CCM, Rodrigues LF, de Avila Pelozin BR, et al. Long Non-Coding RNAs in Cardiovascular Diseases: Potential Function as Biomarkers and Therapeutic Targets of Exercise Training. Noncoding RNA 2021;7:65. [Crossref] [PubMed]
- Li Y, Li C, Li D, et al. lncRNA KCNQ1OT1 enhances the chemoresistance of oxaliplatin in colon cancer by targeting the miR-34a/ATG4B pathway. Onco Targets Ther 2019;12:2649-60. [Crossref] [PubMed]
- Luo ZP, Jin H. Effects of LncRNA KCNQ1OT1 on proliferation and migration of ovarian cancer cells by Wnt/β-catenin. Eur Rev Med Pharmacol Sci 2019;23:8788-94. [PubMed]
- Zheng L, Zhang FX, Wang LL, et al. LncRNA KCNQ1OT1 is overexpressed in non-small cell lung cancer and its expression level is related to clinicopathology. Eur Rev Med Pharmacol Sci 2019;23:6944-50. [PubMed]
- Xu X, Huang CY, Oka SI. LncRNA KCNQ1OT1 promotes Atg12-mediated autophagy via inhibiting miR-26a-5p in ischemia reperfusion. Int J Cardiol 2021;339:132-3. [Crossref] [PubMed]
- Zhao L, Chen H, Wu L, et al. LncRNA KCNQ1OT1 promotes the development of diabetic nephropathy by regulating miR-93-5p/ROCK2 axis. Diabetol Metab Syndr 2021;13:108. [Crossref] [PubMed]
- Zhang S, Ma H, Zhang D, et al. LncRNA KCNQ1OT1 regulates proliferation and cisplatin resistance in tongue cancer via miR-211-5p mediated Ezrin/Fak/Src signaling. Cell Death Dis 2018;9:742. [Crossref] [PubMed]
- Cui J, Zhou B, Ross SA, et al. Nutrition, microRNAs, and Human Health. Adv Nutr 2017;8:105-12. [Crossref] [PubMed]
- Li Y, Liu R, Li J, et al. Isoflurane promotes proliferation and invasion of cervical carcinoma cells via downregulation of miR-375 expression. Eur J Gynaecol Oncol 2019;40:368-72.
- Alwani A, Baj-Krzyworzeka M. MiRNAs - targets in cancer therapy Postepy Biochem 2021;67:259-67. [Crossref] [PubMed]
- Fathi M, Ghafouri-Fard S, Abak A, et al. Emerging roles of miRNAs in the development of pancreatic cancer. Biomed Pharmacother 2021;141:111914. [Crossref] [PubMed]
- Kanwal R, Plaga AR, Liu X, et al. MicroRNAs in prostate cancer: Functional role as biomarkers. Cancer Lett 2017;407:9-20. [Crossref] [PubMed]
- Castro D, Moreira M, Gouveia AM, et al. MicroRNAs in lung cancer. Oncotarget 2017;8:81679-85. [Crossref] [PubMed]
- Lelli D, Pedone C, Majeed M, et al. Curcumin and Lung Cancer: the Role of microRNAs. Curr Pharm Des 2017;23:3440-4. [Crossref] [PubMed]
- Xue ST, Zheng B, Cao SQ, et al. Long non-coding RNA LINC00680 functions as a ceRNA to promote esophageal squamous cell carcinoma progression through the miR-423-5p/PAK6 axis. Mol Cancer 2022;21:69. [Crossref] [PubMed]
- Matsui T, Nuryadi E, Komatsu S, et al. Robustness of Clonogenic Assays as a Biomarker for Cancer Cell Radiosensitivity. Int J Mol Sci 2019;20:4148. [Crossref] [PubMed]
- Wang H, Huo X, Yang XR, et al. STAT3-mediated upregulation of lncRNA HOXD-AS1 as a ceRNA facilitates liver cancer metastasis by regulating SOX4. Mol Cancer 2017;16:136. [Crossref] [PubMed]
- Liz J, Esteller M. lncRNAs and microRNAs with a role in cancer development. Biochim Biophys Acta 2016;1859:169-76. [Crossref] [PubMed]
- Xue M, Zhuo Y, Shan B. MicroRNAs, Long Noncoding RNAs, and Their Functions in Human Disease. Methods Mol Biol 2017;1617:1-25. [Crossref] [PubMed]
- O'Dowd E, Mackenzie J, Balata H. Lung cancer for the non-respiratory physician. Clin Med (Lond) 2021;21:e578-83. [Crossref] [PubMed]
- Coudray N, Ocampo PS, Sakellaropoulos T, et al. Classification and mutation prediction from non-small cell lung cancer histopathology images using deep learning. Nat Med 2018;24:1559-67. [Crossref] [PubMed]
- Huang S, Zhan Z, Li L, et al. LINC00958-MYC positive feedback loop modulates resistance of head and neck squamous cell carcinoma cells to chemo- and radiotherapy in vitro. Onco Targets Ther 2019;12:5989-6000. [Crossref] [PubMed]
- Jarroux J, Morillon A, Pinskaya M. History, Discovery, and Classification of lncRNAs. Adv Exp Med Biol 2017;1008:1-46. [Crossref] [PubMed]
- Huang X, Zhi X, Gao Y, et al. LncRNAs in pancreatic cancer. Oncotarget 2016;7:57379-90. [Crossref] [PubMed]
- Winkle M, El-Daly SM, Fabbri M, et al. Noncoding RNA therapeutics - challenges and potential solutions. Nat Rev Drug Discov 2021;20:629-51. [Crossref] [PubMed]
- Ning B, Yu D, Yu AM. Advances and challenges in studying noncoding RNA regulation of drug metabolism and development of RNA therapeutics. Biochem Pharmacol 2019;169:113638. [Crossref] [PubMed]
- Wang C, Shao S, Deng L, et al. Retraction Note to: LncRNA SNHG12 regulates the radiosensitivity of cervical cancer through the miR-148a/CDK1 pathway. Cancer Cell Int 2022;22:210. [Crossref] [PubMed]
- Yu C, Liang Y, Jin Y, et al. LncRNA GAS5 enhances radiosensitivity of hepatocellular carcinoma and restricts tumor growth and metastasis by miR-144-5p/ATF2. Am J Transl Res 2021;13:10896-907. [PubMed]
- Zhou X, Zeng B, Li Y, et al. LINC02532 Contributes to Radiosensitivity in Clear Cell Renal Cell Carcinoma through the miR-654-5p/YY1 Axis. Molecules 2021;26:7040. [Crossref] [PubMed]
- Liang H, Zhao Q, Zhu Z, et al. Long noncoding RNA LINC00958 suppresses apoptosis and radiosensitivity of colorectal cancer through targeting miR-422a. Cancer Cell Int 2021;21:477. [Crossref] [PubMed]
- Wu D, Li Y, Zhang H, et al. Knockdown of Lncrna PVT1 Enhances Radiosensitivity in Non-Small Cell Lung Cancer by Sponging Mir-195. Cell Physiol Biochem 2017;42:2453-66. [Crossref] [PubMed]
- Jiang H, Hu X, Zhang H, et al. Down-regulation of LncRNA TUG1 enhances radiosensitivity in bladder cancer via suppressing HMGB1 expression. Radiat Oncol 2017;12:65. [Crossref] [PubMed]
- Gao X, Ge J, Li W, et al. LncRNA KCNQ1OT1 ameliorates particle-induced osteolysis through inducing macrophage polarization by inhibiting miR-21a-5p. Biol Chem 2018;399:375-86. [Crossref] [PubMed]
- Gu H, Li Z, Lv XF, et al. LncRNA KCNQ1OT1 delayed fracture healing through the Wnt/β-catenin pathway. Eur Rev Med Pharmacol Sci 2019;23:4575-83. [PubMed]
- Li X, Dai Y, Yan S, et al. Down-regulation of lncRNA KCNQ1OT1 protects against myocardial ischemia/reperfusion injury following acute myocardial infarction. Biochem Biophys Res Commun 2017;491:1026-33. [Crossref] [PubMed]
- Dai W, Chao X, Jiang Z, et al. lncRNA KCNQ1OT1 may function as a competitive endogenous RNA in atrial fibrillation by sponging miR-223-3p. Mol Med Rep 2021;24:870. [Crossref] [PubMed]
- Zhao SF, Ye YX, Xu JD, et al. Long non-coding RNA KCNQ1OT1 increases the expression of PDCD4 by targeting miR-181a-5p, contributing to cardiomyocyte apoptosis in diabetic cardiomyopathy. Acta Diabetol 2021;58:1251-67. [Crossref] [PubMed]
- Tang D, Luo Y, Jiang Y, et al. LncRNA KCNQ1OT1 activated by c-Myc promotes cell proliferation via interacting with FUS to stabilize MAP3K1 in acute promyelocytic leukemia. Cell Death Dis 2021;12:795. [Crossref] [PubMed]
- Wang J, Zhang H, Situ J, et al. KCNQ1OT1 aggravates cell proliferation and migration in bladder cancer through modulating miR-145-5p/PCBP2 axis. Cancer Cell Int 2019;19:325. [Crossref] [PubMed]
- Xian D, Zhao Y. LncRNA KCNQ1OT1 enhanced the methotrexate resistance of colorectal cancer cells by regulating miR-760/PPP1R1B via the cAMP signalling pathway. J Cell Mol Med 2019;23:3808-23. [Crossref] [PubMed]
- Florczuk M, Szpechcinski A, Chorostowska-Wynimko J. miRNAs as Biomarkers and Therapeutic Targets in Non-Small Cell Lung Cancer: Current Perspectives. Target Oncol 2017;12:179-200. [Crossref] [PubMed]
- Huang Y. The novel regulatory role of lncRNA-miRNA-mRNA axis in cardiovascular diseases. J Cell Mol Med 2018;22:5768-75. [Crossref] [PubMed]
- Paraskevopoulou MD, Hatzigeorgiou AG. Analyzing MiRNA-LncRNA Interactions. Methods Mol Biol 2016;1402:271-86. [Crossref] [PubMed]
- Chen X, Zhang N. Downregulation of lncRNA NEAT1_2 radiosensitizes hepatocellular carcinoma cells through regulation of miR-101-3p/WEE1 axis. Cell Biol Int 2019;43:44-55. [Crossref] [PubMed]
- Sun Y, Wang P, Yang W, et al. The role of lncRNA MSC-AS1/miR-29b-3p axis-mediated CDK14 modulation in pancreatic cancer proliferation and Gemcitabine-induced apoptosis. Cancer Biol Ther 2019;20:729-39. [Crossref] [PubMed]
- Yang QS, Li B, Xu G, et al. Long noncoding RNA LINC00483/microRNA-144 regulates radiosensitivity and epithelial-mesenchymal transition in lung adenocarcinoma by interacting with HOXA10. J Cell Physiol 2019;234:11805-21. [Crossref] [PubMed]
- Yi L, Ouyang L, Wang S, et al. Long noncoding RNA PTPRG-AS1 acts as a microRNA-194-3p sponge to regulate radiosensitivity and metastasis of nasopharyngeal carcinoma cells via PRC1. J Cell Physiol 2019;234:19088-102. [Crossref] [PubMed]
- Zhao X, Tang DY, Zuo X, et al. Identification of lncRNA-miRNA-mRNA regulatory network associated with epithelial ovarian cancer cisplatin-resistant. J Cell Physiol 2019;234:19886-94. [Crossref] [PubMed]
- Chen T, Li Y, Cao W, et al. miR-491-5p inhibits osteosarcoma cell proliferation by targeting PKM2. Oncol Lett 2018;16:6472-8. [Crossref] [PubMed]
- Lu L, Cai M, Peng M, et al. miR-491-5p functions as a tumor suppressor by targeting IGF2 in colorectal cancer. Cancer Manag Res 2019;11:1805-16. [Crossref] [PubMed]
- Sun R, Liu Z, Tong D, et al. miR-491-5p, mediated by Foxi1, functions as a tumor suppressor by targeting Wnt3a/β-catenin signaling in the development of gastric cancer. Cell Death Dis 2017;8:e2714. [Crossref] [PubMed]
- Xu Y, Hou R, Lu Q, et al. MiR-491-5p negatively regulates cell proliferation and motility by targeting PDGFRA in prostate cancer. Am J Cancer Res 2017;7:2545-53. [PubMed]
- Zhang Q, Li Q, Xu T, et al. miR-491-5p suppresses cell growth and invasion by targeting Notch3 in nasopharyngeal carcinoma. Oncol Rep 2016;35:3541-7. [Crossref] [PubMed]
- Gong F, Ren P, Zhang Y, et al. MicroRNAs-491-5p suppresses cell proliferation and invasion by inhibiting IGF2BP1 in non-small cell lung cancer. Am J Transl Res 2016;8:485-95. [PubMed]
- Garrido G, Vernos I. Non-centrosomal TPX2-Dependent Regulation of the Aurora A Kinase: Functional Implications for Healthy and Pathological Cell Division. Front Oncol 2016;6:88. [Crossref] [PubMed]
- Koike Y, Yin C, Sato Y, et al. TPX2 is a prognostic marker and promotes cell proliferation in neuroblastoma. Oncol Lett 2022;23:136. [Crossref] [PubMed]
- Zou J, Huang RY, Jiang FN, et al. Overexpression of TPX2 is associated with progression and prognosis of prostate cancer. Oncol Lett 2018;16:2823-32. [Crossref] [PubMed]
- Tomii C, Inokuchi M, Takagi Y, et al. TPX2 expression is associated with poor survival in gastric cancer. World J Surg Oncol 2017;15:14. [Crossref] [PubMed]
- An R, Cheng L, Chen L, et al. Plk1 interacts with RNF2 and promotes its ubiquitin-dependent degradation. Oncol Rep 2018;39:2358-64. [Crossref] [PubMed]
- Wei M, Jiao D, Han D, et al. Knockdown of RNF2 induces cell cycle arrest and apoptosis in prostate cancer cells through the upregulation of TXNIP. Oncotarget 2017;8:5323-38. [Crossref] [PubMed]
- Zhang J, Sun Z, Han Y, et al. Rnf2 knockdown reduces cell viability and promotes cell cycle arrest in gastric cancer cells. Oncol Lett 2017;13:3817-22. [Crossref] [PubMed]
- Yang J, Yu F, Guan J, et al. Knockdown of RNF2 enhances the radiosensitivity of squamous cell carcinoma in lung. Biochem Cell Biol 2019;97:589-99. [Crossref] [PubMed]
(English Language Editor: C. Betlazar-Maseh)