Pneumothorax/pneumomediastinum and pre-existing lung pathology in ventilated COVID-19 patients: a cohort study
Introduction
Pneumothorax (PTX), is an accumulation of free air within the virtual space between visceral and parietal pleura (1). Spontaneous PTX develops in seemingly healthy lung, while secondary PTX needs a lung affected by some underlying chronic pathology, such as bullous emphysema. The air either enters the pleural space through the chest wall (traumatic PTX) or “inside-out” from the alveoli through the injured visceral pleura into the intrapleural space. Artificial PTX was nearly the exclusive invasive therapy of tuberculosis for more than half a century (2). The hypothesis list of the possible mechanisms and the predisposing factors for the “spontaneous” free air accumulation is long (3-5). Pneumomediastinum (PM) is a different phenomenon, meaning the presence of extraluminal gas within the mediastinum. The true pathogenesis remains to be obscure in all but the obvious openings/wounds of the tracheobronchial wall and/or oesophagus (6,7).
PTX as a presenting phenomenon was described also in the 2003 severe acute respiratory syndrome (SARS) (8) and there is an increasing number of reports on developing PM/PTX among severe acute respiratory syndrome coronavirus disease 2019 (SARS-COVID-19) patients as well (9-15). The jury is still out on the question regarding the clinical relevance of the PM/PTX, which is reflected in the controversies surrounding the best clinical practice. The question in our non-interventional, observational, prospective, single centre study was whether pre-existing lung pathology increases the chance of developing PTX and PM among mechanically ventilated patients (16). We present the following article in accordance with the STROBE reporting checklist (available at https://jtd.amegroups.com/article/view/10.21037/jtd-22-817/rc).
Methods
Altogether 138 consecutive patients with polymerase chain reaction confirmed COVID-19 infection were treated at the Intensive Care Unit (ICU) of Petz Aladár University Teaching Hospital, Győr between 1st March 2020 and 1st February 2021. The study was conducted in accordance with the Declaration of Helsinki (as revised in 2013). The study was approved by the Regional Science and Research Ethics Committee of Petz Aladar University Teaching Hospital (No. 76-1-8/2021) and individual consent for this retrospective analysis was waived. Admission criteria to the ICU included PaO2 <65 mmHg or O2 saturation <92% on reservoir O2 bag, or hypotension [mean arterial pressure (MAP) <70 mmHg] or acidosis, or rapidly deteriorating clinical status due to the impaired gas exchange functions. Based on the currently valid evidence in adult respiratory distress syndrome (ARDS) ventilation strategy, we tried not to exceed the maximum end-inspiratory pressure of 30–35 mbar. Depending on lung compliance, allowing permissive hypercapnia, we set a tidal volume of 6 mL/kg. Basically, we used pressure-controlled ventilation or airway pressure release ventilation (17). Computer tomography (CT) was performed in 60/138 (43.48%) patients. Three independent observers evaluated pre-existing lung pathologies and pleural and mediastinal space abnormalities.
Electronic patient dataset provided checking anamnestic information. All records of the patients included were evaluated with the Hungarian patient-specific (Health Insurance Number coded) electronic database. The analysis was secured by full anonymization protocol. As far as the CT images are concerned, tissue free areas within the pleural space and mediastinal and/or pericardial collection with free air density have been graded. Bullae, pneumokele, fluid-free lung cysts less than 10% of the relevant lobe were categorised as stage I, destroyed lung, covering >50% of the ipsilateral hemithorax were categorised as stage III underlying destructive lung pathology. All positive cases between stages I and III were considered as stage II destructions. PTX less than 20% of ipsilateral lung surface was Grade 1 (Figure 1), while total PTX and those subtotal ones causing tension PTX were categorised as Grade 3. Any extension of free air collection between Grade 1 and 3 was categorised as Grade 2. The PM group was treated as a separate entity (Figure 2). Iatrogenic PTX was excluded from the study.
Statistical analysis
IBM SPSS 28 statistical software served data analysis. Descriptive analysis of the database was performed using the frequency function. Differentiating between the groups with or without previous lung pathology, Kruskal-Wallis test was used to determine whether there was a statistically significant difference in the development of PTX/PM between groups. Spearman correlation tested the connection between previous lung pathology and onset of PTX/PM. We performed logistic regression analysis to determine how the co-presence of age, sex, previous lung pathology, mechanical ventilation (MV)/non-invasive ventilation (NIV) variables affect PTX/PM as an outcome. Cox-regression analysis was executed to see how the presence of different previous lung pathology groups influence the days needed to develop PTX/PM in patients.
Results
The mean age of the 60 patients was 65.48 years, the median was 67.50 years (range, 31–85 years). The sex ratio was 75–25% in favour of men (Table 1). Table 2 summarises the PTX/PM cases compared to pre-existing lung status, stages of pathology and grade of PTX of the patients. Of the 60 patients, 44 were those who required controlled MV via endotracheal tube, whereas 16 pressure supported ventilation via full face mask (NIV) (Table 3). Altogether 13 patients developed PTX. Descriptive statistics by age showed that the mean age of men and women was similar (65.76 vs. 64.76 years). Pre-existing lung pathology was more prevalent in older patients, with an average age of 70.24 years, compared to 63.60 years for patients without lung pathology. The average age of the patients with controlled ventilation was 66.98 years, while the average age of the group with supported ventilation was 61.38 years. The difference in mean age narrowed when the onset of PTX/PM was examined: among those with injured visceral pleura integrity it was 66.31 years, compared to 65.26 years in the group without PTX/PM (Table 4). There was a male predominance in terms of existing lung pathology, with only 2 women for every 15 men, although this could be explained by the fact that there were more men in the study. PTX/PM developed in 27.3% of our patients. Among patients with pre-existing chronic lung pathology, 58.8% required controlled MV with intubation, and only 17.6% developed PTX/PM (Tables 5,6).
Table 1
Variables | Values |
---|---|
N | 60 |
Mean (years) | 65.48 |
Median (years) | 67.50 |
Mode (years) | 66 |
Standard deviation (years) | 10.881 |
Minimum (years) | 31 |
Maximum (years) | 85 |
Table 2
PTX vs. lung status | No pre-existing pathology |
Stage I, destroyed lung <10% | Stage II, destroyed lung 10–50% | Stage III, destroyed lung >50% | Total |
---|---|---|---|---|---|
No PTX | 33 | 3 | 1 | 10 | 47 |
Grade 1 PTX | 1 | 1 | 0 | 0 | 2 |
Grade 2 PTX | 4 | 0 | 0 | 1 | 5 |
Grade 3 PTX | 1 | 1 | 0 | 0 | 2 |
PM | 4 | 0 | 0 | 0 | 4 |
Total | 43 | 5 | 1 | 11 | 60 |
PTX, pneumothorax; PM, pneumomediastinum.
Table 3
Variables | Groups | Number | Percent (%) |
---|---|---|---|
Sex | Male | 45 | 75.0 |
Female | 15 | 25.0 | |
Pathology | Yes | 17 | 28.3 |
No | 43 | 71.7 | |
Ventilation | MV | 44 | 73.3 |
NIV | 16 | 26.7 | |
PTX/PM | Yes | 13 | 21.7 |
No | 47 | 78.3 | |
Survival | Exit | 41 | 68.3 |
Alive | 19 | 31.7 |
PTX, pneumothorax; PM, pneumomediastinum; MV, mechanical ventilation; NIV, non-invasive ventilation.
Table 4
Variables | Number | Mean (years) | Median (years) | Mode (years) | Std. deviation (years) | Minimum (years) | Maximum (years) |
---|---|---|---|---|---|---|---|
Male | 45 | 65.76 | 69.00 | 69 | 10.971 | 31 | 79 |
Female | 15 | 64.67 | 66.00 | 66 | 10.939 | 47 | 85 |
Pathology: yes | 17 | 70.24 | 70.00 | 66 | 6.897 | 54 | 79 |
Pathology: no | 43 | 63.60 | 66.00 | 66 | 11.638 | 31 | 85 |
Ventilation: MV | 44 | 66.98 | 68.50 | 66 | 9.559 | 42 | 85 |
Ventilation: NIV | 16 | 61.38 | 64.00 | 31 | 13.386 | 31 | 78 |
PTX + PM: yes | 13 | 66.31 | 70.00 | 31 | 15.966 | 31 | 85 |
PTX + PM: no | 47 | 65.26 | 67.00 | 66 | 9.225 | 44 | 79 |
MV, mechanical ventilation; NIV, non-invasive ventilation; Std., standard; PTX, pneumothorax; PM, pneumomediastinum.
Table 5
Pathology | Sex, n (%) | MV/NIV, n (%) | PTX/PM, n (%) | ||||||||
---|---|---|---|---|---|---|---|---|---|---|---|
Male | Female | Total | Yes | No | Total | Yes | No | Total | |||
Yes | 15 (88.2) | 2 (11.8) | 17 (100.0) | 10 (58.8) | 7 (41.2) | 17 (100.0) | 3 (17.6) | 14 (82.4) | 17 (100.0) | ||
No | 30 (69.8) | 13 (30.2) | 43 (100.0) | 34 (79.1) | 9 (20.9) | 43 (100.0) | 10 (23.3) | 33 (76.7) | 43 (100.0) |
MV, mechanical ventilation; NIV, non-invasive ventilation; PTX, pneumothorax; PM, pneumomediastinum.
Table 6
Ventilation | Sex, n (%) | PTX/PM, n (%) | |||||
---|---|---|---|---|---|---|---|
Male | Female | Total | Yes | No | Total | ||
MV | 32 (72.7) | 12 (27.3) | 44 (100.0) | 12 (27.3) | 32 (72.7) | 44 (100.0) | |
NIV | 13 (81.3) | 3 (18.8) | 16 (100.0) | 1 (6.3) | 15 (93.8) | 16 (100.0) |
PTX, pneumothorax; PM, pneumomediastinum; MV, mechanical ventilation; NIV, non-invasive ventilation.
In the subgroups of patients with and without underlying lung pathology, the occurrence of PTX or PM were 16.16% vs. 23.25% respectively. Survival rates for previously “healthy-lung” COVID-19 sufferers were 37.20% opposed to the 22.22% for stage III pre-existing lung pathology.
The results of the Kruskal-Wallis test showed that there was no statistically significant difference (P<0.05) between the different PTX stages [1, 2, 3] and the development of PM in patients with previous lung pathology (P=0.673). In other words, it did not matter which group the patient belonged to in terms of PTX development. Spearman correlation did not show a statistically significant association between pre-existing chronic lung pathology and the development of PTX stages and PM (r=−0.098, P=0.456). The Cox regression did not show a statistically significant result when examining how pre-existing lung pathology affect the time to onset of PTX (P=0.602) (Table 7).
Table 7
Statistical analysis | P | r | HR | 95% CI |
---|---|---|---|---|
Kruskal-Wallis Test | 0.673 | – | – | – |
Spearman correlation | 0.456 | −0.098 | – | – |
Cox regression | 0.602 | – | 0.808 | 0.364–1.797 |
HR, hazard ratio; CI, confidence interval.
Logistic regression did not yield statistically significant results for any of the variables when analysed together with age, sex, ventilation mode, and the size of lung pathology (Figure 3). The model included age, sex, MV/NIV ventilatory strategy, and chronic pre-existing lung pathology. The model produced was calculated by SPSS to reflect reality (P<0.001), and the predictive value of the model was 78.3%. The lowest odds ratio was obtained for the age variable however, the result is statistically not significant [odds ratio (OR) =1.001, 95% confidence interval (95% CI): 0.937–1.068, P=0.985]. The odds ratio for lung pathology was 1.209, but the results are also not significant (95% CI: 0.597–2.450, P=0.598). The odds ratio for sex was 1.356 with a confidence interval of 0.304–6.047, which is statistically not significant (P=0.689). For ventilation, we obtained the highest odds ratio of 5.221 with a confidence interval of 0.562–48.515 and the lowest significance value but it was also statistically not significant (P=0.146) (Figure 3).
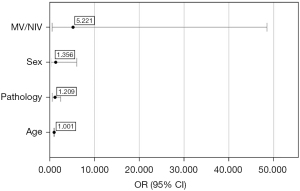
Discussion
Our hypothesis, that the increased number of PM and secondary PTX in ventilated COVID-19 patients has a relationship with pre-existing lung pathology was not confirmed.
While barotrauma induced PTX and PM is a well-known, but rare phenomenon in ventilated ARDS patients (18), a stark increase in PTX and PM have been observed from the beginning of the SARS-COVID-19 second pandemic wave, between March 2020 and March 2021 in Hungary (19). The COVID-19 PTXs observed by us and reported by others (20,21) seem to represent a distinctively specific pattern, a different situation. In an ordinary spontaneous PTX situation, the apex of the lung is expected to come down first, followed by the lateral surface. Lung parenchyma shrinkage due to viral inflammation consolidation rather that true intrapleural pressure compression is the main cause of PTX when it is circumferential (Grade 3 PTX). Our experience of lack of significant amount of air escape at the very time of drainage supports this hypothesis.
The lack of expected increase of frequency in PTX/PM among patients with pre-existing chronic lung parenchyma pathologies might be offered by two, mutually not exclusive explanations. The first, mechanical approach might be based on an increased resilience against pressure trauma as the dominance of the fibrotic elements. A similar pattern was recently identified in blunt liver trauma (22).
The second explanation is in a need of revisiting the mechanism leading to the PTX itself. Bullous part of visceral pleura is considered as a weak area, breaking point during ventilation. A reasonable explanation for the air escape is offered by the localised vascular necrosis of the external layer of the coat of the lung. The surgical finding of one of the resected bullae being rotten (23) supports this hypothesis. Venous micro thrombosis, capillary wall thickening is a proven consequence of COVID-19 infection, a phenomenon leading to wet necrosis, hence the cited rotten bulla.
Whatever is the mechanism of the intrapleural accumulation of the air, the lateral lung surface tends to be tethered to the parietal pleura in all but the Grade 3 PTX cases. The mediastinal face of the visceral pleura remains relatively free due to the permanent movement of the adjacent heart and great vessels providing space for developing circumscript free air containing regions. The vessel rich hilar region, with transmitted micro vibrations of blood flow is also working against the permanent juxtaposition needed for mediastinal-pleura symphysis. Microporous—necrotic and/or mechanical minimal ruptures are allowing air to dissect along the broncho vascular sheath. The theoretical explanations offered by Macklin (24) the air spreads along peribronchovascular sheets to the mediastinum. The barotrauma hypothesis is contradicted by the several spontaneous PTX cases without ventilation reported by other authors (25-31), and by our own biomechanical experiments (32,33): resistance of resected pleural bullae walls up to 1,938 cmH2O against pressure is another strong evidence against barotrauma caused PTX in ventilated patients (32).
Our study also has limitations. First, this is a study using data from a single institution, and the study population is small. Therefore, higher case number or data from more centres would be beneficial. Second, the treatment protocol of PTX/PM was expert opinion based and highly individualised instead of being strictly standardised. Third, as time passed by, the treatment policy underwent a significant shift towards the less invasive ventilatory strategies, causing undesired heterogeneity in the study population.
Conclusions
In conclusion, our results suggest, that the pre-existing diffuse lung pathologies do not increase the chances of onset of PTX/PM in ventilated COVID-19 patients. What is clear, PM and PTX in these cases are primarily consequences of the viral inflammation and consequent lung consolidation, rather than direct pressure injury. The evidence provided needs further confirmation in the alveolar barotrauma versus visceral pleura vulnerability debate sparked by the authors.
Acknowledgments
Funding: The project was supported by the TKP2021-NVA-06 and the 2020-4.1.1-TKP2020 grants, provided by the Hungarian Government and the European Union, and the Thematic Excellence Program 2020-National Excellence Subprogram; Biomedical Engineering Project (No. 2020-4.1.1-TKP2020).
Footnote
Reporting Checklist: The authors have completed the STROBE reporting checklist. Available at https://jtd.amegroups.com/article/view/10.21037/jtd-22-817/rc
Data Sharing Statement: Available at https://jtd.amegroups.com/article/view/10.21037/jtd-22-817/dss
Peer Review File: Available at https://jtd.amegroups.com/article/view/10.21037/jtd-22-817/prf
Conflicts of Interest: All authors have completed the ICMJE uniform disclosure form (available at https://jtd.amegroups.com/article/view/10.21037/jtd-22-817/coif). The authors have no conflicts of interest to declare.
Ethical Statement: The authors are accountable for all aspects of the work in ensuring that questions related to the accuracy or integrity of any part of the work are appropriately investigated and resolved. The study was conducted in accordance with the Declaration of Helsinki (as revised in 2013). The study was approved by the Regional Science and Research Ethics Committee of Petz Aladar University Teaching Hospital (No. 76-1-8/2021) and individual consent for this retrospective analysis was waived.
Open Access Statement: This is an Open Access article distributed in accordance with the Creative Commons Attribution-NonCommercial-NoDerivs 4.0 International License (CC BY-NC-ND 4.0), which permits the non-commercial replication and distribution of the article with the strict proviso that no changes or edits are made and the original work is properly cited (including links to both the formal publication through the relevant DOI and the license). See: https://creativecommons.org/licenses/by-nc-nd/4.0/.
References
- Schneider L, Reissman II. Idiopathic spontaneous pneumothorax: history of 100 unselected cases. Radiology 1945;44:485-8.
- Molnar TF. Tuberculosis: mother of thoracic surgery then and now, past and prospectives: a review. J Thorac Dis 2018;10:S2628-42. [Crossref] [PubMed]
- Papagiannis A, Lazaridis G, Zarogoulidis K, et al. Pneumothorax: an up to date "introduction". Ann Transl Med 2015;3:53. [Crossref] [PubMed]
- McKnight CL, Burns B. Pneumothorax. Treasure Island (FL): StatPearls Publishing; 2022.
- Noppen M, De Keukeleire T. Pneumothorax. Respiration 2008;76:121-7. [Crossref] [PubMed]
- Caceres M, Ali SZ, Braud R, et al. Spontaneous pneumomediastinum: a comparative study and review of the literature. Ann Thorac Surg 2008;86:962-6. [Crossref] [PubMed]
- Sahni S, Verma S, Grullon J, et al. Spontaneous pneumomediastinum: time for consensus. N Am J Med Sci 2013;5:460-4. [Crossref] [PubMed]
- Sihoe AD, Wong RH, Lee AT, et al. Severe acute respiratory syndrome complicated by spontaneous pneumothorax. Chest 2004;125:2345-51. [Crossref] [PubMed]
- Volpi S, Ali JM, Suleman A, et al. Pneumomediastinum in COVID-19 patients: a case series of a rare complication. Eur J Cardiothorac Surg 2020;58:646-7. [Crossref] [PubMed]
- Kangas-Dick A, Gazivoda V, Ibrahim M, et al. Clinical characteristics and outcome of pneumothorax in patients with COVID-19 pneumonia. Chest 2020;158:A2648. [Crossref] [PubMed]
- McGuinness G, Zhan C, Rosenberg N, et al. Increased Incidence of Barotrauma in Patients with COVID-19 on Invasive Mechanical Ventilation. Radiology 2020;297:E252-62. [Crossref] [PubMed]
- Wang XH, Duan J, Han X, et al. High incidence and mortality of pneumothorax in critically Ill patients with COVID-19. Heart Lung 2021;50:37-43. [Crossref] [PubMed]
- Salehi S, Abedi A, Balakrishnan S, et al. Coronavirus Disease 2019 (COVID-19): A Systematic Review of Imaging Findings in 919 Patients. AJR Am J Roentgenol 2020;215:87-93. [Crossref] [PubMed]
- Lacroix M, Graiess F, Monnier-Cholley L, et al. SARS-CoV-2 pulmonary infection revealed by subcutaneous emphysema and pneumomediastinum. Intensive Care Med 2020;46:1620-1. [Crossref] [PubMed]
- Rajdev K, Spanel AJ, McMillan S, et al. Pulmonary Barotrauma in COVID-19 Patients With ARDS on Invasive and Non-Invasive Positive Pressure Ventilation. J Intensive Care Med 2021;36:1013-7. [Crossref] [PubMed]
- Belletti A, Palumbo D, Zangrillo A, et al. Predictors of Pneumothorax/Pneumomediastinum in Mechanically Ventilated COVID-19 Patients. J Cardiothorac Vasc Anesth 2021;35:3642-51. [Crossref] [PubMed]
- Shrestha DB, Sedhai YR, Budhathoki P, et al. Pulmonary barotrauma in COVID-19: A systematic review and meta-analysis. Ann Med Surg (Lond) 2022;73:103221. [Crossref] [PubMed]
- Anzueto A, Frutos-Vivar F, Esteban A, et al. Incidence, risk factors and outcome of barotrauma in mechanically ventilated patients. Intensive Care Med 2004;30:612-9. [Crossref] [PubMed]
- Özdemir S, Bilgi DÖ, Köse S, et al. Pneumothorax in patients with coronavirus disease 2019 pneumonia with invasive mechanical ventilation. Interact Cardiovasc Thorac Surg 2021;32:351-5. [Crossref] [PubMed]
- Elhakim TS, Abdul HS, Pelaez Romero C, et al. Spontaneous pneumomediastinum, pneumothorax and subcutaneous emphysema in COVID-19 pneumonia: a rare case and literature review. BMJ Case Rep 2020;13:239489. [Crossref] [PubMed]
- Martinelli AW, Ingle T, Newman J, et al. COVID-19 and pneumothorax: a multicentre retrospective case series. Eur Respir J 2020;56:2002697. [Crossref] [PubMed]
- Simon G, Poór VS, Heckmann V, et al. The effect of steatosis and fibrosis on blunt force vulnerability of the liver. Int J Legal Med 2020;134:1067-72. [Crossref] [PubMed]
- Bellini R, Salandini MC, Cuttin S, et al. Spontaneous pneumothorax as unusual presenting symptom of COVID-19 pneumonia: surgical management and pathological findings. J Cardiothorac Surg 2020;15:310. [Crossref] [PubMed]
- Macklin CC. Transport of air along sheaths of pulmonic vessels from alveoli to mediastinum: clinical implications. Arch Intern Med (Chic) 1939;64:913-26.
- Gorospe L, Ayala-Carbonero A, Ureña-Vacas A, et al. Spontaneous Pneumomediastinum in Patients With COVID-19: A Case Series of Four Patients. Arch Bronconeumol 2020;56:754-6. (Engl Ed). [Crossref] [PubMed]
- Miró Ò, Llorens P, Jiménez S, et al. Frequency, Risk Factors, Clinical Characteristics, and Outcomes of Spontaneous Pneumothorax in Patients With Coronavirus Disease 2019: A Case-Control, Emergency Medicine-Based Multicenter Study. Chest 2021;159:1241-55. [Crossref] [PubMed]
- Mohan V, Tauseen RA. Spontaneous pneumomediastinum in COVID-19. BMJ Case Rep 2020;13:236519. [Crossref] [PubMed]
- Shan S, Guangming L, Wei L, et al. Spontaneous pneumomediastinum, pneumothorax and subcutaneous emphysema in COVID-19: case report and literature review. Rev Inst Med Trop Sao Paulo 2020;62:e76. [Crossref] [PubMed]
- Juárez-Lloclla JP, León-Jiménez F, Urquiaga-Calderón J, et al. Spontaneous Pneumopericardium and Pneumomediastinum in Twelve COVID-19 Patients. Arch Bronconeumol 2021;57:86-8. [Crossref] [PubMed]
- Rohailla S, Ahmed N, Gough K. SARS-CoV-2 infection associated with spontaneous pneumothorax. CMAJ 2020;192:E510. [Crossref] [PubMed]
- Wang W, Gao R, Zheng Y, et al. COVID-19 with spontaneous pneumothorax, pneumomediastinum and subcutaneous emphysema. J Travel Med 2020;27:taaa062. [Crossref] [PubMed]
- Zsoldos P, Simon G, Elo G, et al. Search for the cause of spontaneous pneumothorax: physics of an unresolved enigma. Interactive Cardiovascular and Thoracic Surgery 2017;25:ivx280.134.
- Yang HC, Jung S. Bullae formation hypothesis in primary spontaneous pneumothorax. J Thorac Dis 2020;12:2833-7. [Crossref] [PubMed]