Noninvasive and invasive positive pressure ventilation for acute respiratory failure in critically ill patients: a comparative cohort study
Introduction
Noninvasive positive pressure ventilation (NPPV) is an increasingly accepted method to manage selected cases of acute respiratory failure (1). Numerous studies and meta-analyses have been performed in order to establish the efficacy of NPPV in various subsets of patients (1-3). For patients suffering from acute respiratory failure due to exacerbations of chronic obstructive pulmonary disease (COPD) or congestive heart failure, as well as in immunosuppressed patients with pulmonary infiltrates, the addition of NPPV to standard care is associated with a marked reduction in intubation rate, complications, hospital length of stay and even mortality (3-7).
Only a limited number of studies investigated NPPV as an alternative to intubation in patients with acute respiratory failure (3,8-12). NPPV use resulted in fewer complications and lower readmission rates without changes in mortality (3).
Not all patients seem to fully benefit from NPPV. Compared with COPD exacerbations and congestive heart failure NPPV efficacy data show less beneficial outcomes for post-surgical patients and patients with asthma exacerbations, pneumonia, acute lung injury (ALI) or acute respiratory distress syndrome (ARDS) (13-17). Despite weaker recommendations and increased NPPV failure rates, still NPPV is regularly used for these indications (18).
NPPV failure, defined as the need for intubation after a trial of NPPV, is associated with various risk factors, including age, severity of illness scores, the presence of ARDS or community acquired pneumonia, acidosis (pH <7.25), persistent tachypnea, persistent hypercapnia, and poor neurologic scores (1,18-22). NPPV failure rates vary markedly from 5% to 60%, according to the etiology of respiratory failure and various other relevant factors (19). In a multicenter database study of critically ill patients a small increase in the success rate of NPPV was found over the last two decades (18). Early predictors of NPPV failure are relevant to select the appropriate patients in daily practice.
If successful, NPPV allows for the circumvention of many of the complications associated with mechanical ventilation, especially the occurrence of nosocomial infections (e.g., ventilator associated pneumonia), critical illness-associated weakness, pneumothorax or delirium (3).
Although several studies have indicated that patients failing NPPV have worse outcomes compared with patients with successful NPPV treatment, these outcomes are not compared with patients that were intubated without a prior trial of NPPV. Hence, very limited data is available on risks associated with NPPV failure resulting in (delayed) intubation. Few studies suggested that patients who fail NPPV and subsequently require endotracheal intubation experience significantly higher mortality and longer hospitalization than patients primarily intubated (8,23,24). More information to value the potential negative effects of NPPV failure in comparison to primary intubation would be of great value.
Therefore, the objective of this retrospective single-center study is to compare and contrast potential differences in clinical variables and outcomes in critically ill patients with acute respiratory failure who were either treated successfully or unsuccessfully with NPPV. We studied risk factors on ICU admission that were associated with NPPV failure on baseline, after 1 hour and as changes from baseline after 1 hour. To put these findings into perspective, clinical outcomes are compared to patients who were initially intubated without a trial of NPPV in order to assess the possible risks associated with NPPV failure and delayed intubation.
Methods
Study design and participants
We conducted a retrospective observational study using data from patients admitted to our 17-beds mixed medical-surgical ICU, Gelderse Vallei Hospital, in Ede, The Netherlands. All patients in the period of January 1st, 2013 to December 31st, 2014 diagnosed with acute hypoxemic or hypercapnic respiratory failure treated with NPPV were evaluated. Diagnostic criteria for respiratory failure were: hypoxemia [partial pressure of oxygen (PaO2) <9.0 kPa], pH ≤7.35 and/or partial pressure of carbon dioxide (PaCO2) ≥6.0 kPa, with dyspnoea, signs of increased respiratory work and a respiratory rate of >30 breaths/min. Patients were excluded from this study if they were aged <18 years, when they used NPPV chronically in the home care setting, in case of degenerative neuromuscular disease or obstructive sleep apnoea syndrome, if there was a no-intubation decision, or when NPPV was used during the weaning phase after detubation of invasive mechanical ventilation. If follow-up data were incomplete due to transfer to another hospital patients were excluded as well. In case of multiple admissions during the study period, only the first eligible admission was evaluated.
Subsequently, a comparable group of patients with acute respiratory failure who were primarily intubated was collected in order to match with the group of patients who failed NPPV. A sample of 40 patients was randomly selected from a total of 803 patients who were admitted to the intensive care during the study period for primary mechanical ventilation and acute respiratory failure meeting similar criteria for respiratory failure. Reasons not to apply NPPV in our ICU are unconsciousness, hemodynamic instability due to e.g., sepsis, pneumothorax, anatomical and/or subjective difficulty in airway access, recent facial or upper gastrointestinal surgery and gastrointestinal bleeding. Every 20th patient was selected, or the next patient in case a patient was found not eligible for inclusion. Only patients diagnosed with acute respiratory failure were included. Patients were not included when they needed post-operative ventilation or ventilation after cardiac arrest, seizures or intoxication. In case of incomplete data due to transfer to another hospital, patients were excluded from analysis.
Data collection and baseline measurements
The following variables were obtained from the Patient Data Management System (PDMS; iMDsoft MetaVision®, Tel Aviv, Israel) for all patients: age, gender, body mass index (BMI), Acute Physiology and Chronic Health Evaluation-II score (APACHE-II), Simplified Acute Physiology II score (SAPS-II), Sequential Organ Failure Assessment score (SOFA), the presence of severe sepsis on ICU admission, Glasgow Coma Score (GCS), Barthel score, time from hospital admission to ICU admission, time between ICU admission and start of ventilation, and after 1 hour of ventilation: urine production, vasopressor use (noradrenalin) and intravenous sedative use (propofol, midazolam, fentanyl, haloperidol or morphine). In addition, hemodynamic variables, respiratory variables, ventilatory variables and arterial blood gas values before start of ventilation and after 1 hour of ventilation, were collected. In addition, the primary diagnosis was recorded, categorized as cardiogenic pulmonary oedema, exacerbation COPD, pneumonia or ARDS. Cardiogenic pulmonary edema was defined as dyspnoea of sudden onset, typical findings of fluid overload on a chest X-ray, and widespread rales without a history suggesting pulmonary aspiration or infection. Exacerbation COPD was defined as an acute worsening of respiratory symptoms in patients with COPD, associated with a variable degree of physiological deterioration. Pneumonia was diagnosed according to the Centers for Disease Control and Prevention definitions (25). ARDS was defined according to the Berlin definition, including the level of positive end expiratory pressure (PEEP) used in ventilation ratio of arterial oxygen tension to fraction of inspired oxygen (PF-ratio) and chest radiography changes (26). Furthermore, comorbidities were documented, including: arterial hypertension (defined as the use of antihypertensive drugs or diuretics for hypertension), COPD according to the GOLD classification (27) or recorded by a pulmonologist, congestive heart failure according to the New York Heart Association classification (28), renal failure [defined as creatinine level >1.77 mmoL/mL (2 mg/dL)] (29) and immunodepression (defined as neutropenia <1,000/mm3 after bone marrow transplantation or anticancer chemotherapy, immunosuppressive therapy for solid organ transplantation, or connective tissue disorder requiring corticosteroid therapy of at least 20 mg/day for at least 3 weeks). In addition, the Charlson comorbidity index was determined for each patient (30).
Outcome parameters
The primary aim of the study was to identify clinical variables associated with NPPV failure. Secondary outcome parameters, including in-hospital mortality, length of (noninvasive) mechanical ventilation, length of ICU stay, length of hospital stay, incidence of ICU acquired pneumonia (defined as pneumonia diagnosed 3 days after ICU admission in order to exclude community acquired pneumonia) and incidence of organ failure after 48 hours (reflected by the SOFA score at 48 hours), were compared within three subgroups of patients: NPPV success, NPPV failure and primary intubation.
We defined NPPV success as no need for intubation within 48 hours. Clinical improvement with decrease in respiratory rate and heart rate, increase in pH and PaO2 and ability to wean the patient from NPPV were observed or anticipated. Need for intubation, according to our internal guidelines and/or clinical judgement, was diagnosed to the discretion of the attending physician.
Statistical analysis
Categorical variables were expressed as numbers and percentages. Baseline characteristics for frequencies were tested using chi-square tests. Continuous variables were presented as median and interquartile range (IQR, 25–75). In case data were not normally distributed according to the Shapiro-Wilks test, comparisons between independent groups’ data were analysed using the Mann-Whitney U-test. Delta values were computed for respiratory, laboratory and arterial values as variables after 1 hour minus baseline variables for the same patient and were expressed as means and standard deviations (SD). These values were tested with independent t-tests.
To evaluate the risk factors associated with NPPV failure, a multivariate logistic regression model was created. Variables that were associated with NPPV failure in univariate analysis (factors yielding P values <0.10) were entered. A forward selection process identified the final model containing no more than four predictor variables.
Secondary outcome parameters were adjusted according to baseline differences by using logistic regression analyses, employing NPPV failure as the reference category. Adjusted odds ratios (OR) and 95% CI were computed.
All tests of significance were two-sided and statistical significance was considered at P<0.05. Statistical analyses were performed using IBM SPSS Statistical Software, version 22.0.
Societal and ethical justification
The study was approved by the Institutional Review Board of Gelderse Vallei Hospital. The need for informed consent was waived because of the retrospective nature of the study using coded data obtained from routine care.
Results
During the study period, a total of 1,188 patients were admitted to the ICU for ventilation. Overall, 385 patients were treated with NPPV (32.4%). Of these, 133 NPPV patients were eligible for analysis and were included. A total of 803 patients were admitted to the ICU for invasive mechanical ventilation, without undergoing a trial of NPPV. A sample of 40 patients was randomly selected. Figure 1 shows the flowchart depicting the selection process and patients flow through the study and those patients excluded from analysis.
Baseline characteristics
Patient baseline characteristics are displayed in Table 1, stratified by NPPV success, NPPV failure and primary intubation. Compared to patients who were successfully treated with NPPV, those who failed NPPV and those who were primarily intubated had significantly higher severity of illness scores (APACHE-II, SAPS-II and SOFA-scores). Patients primarily intubated showed higher SOFA-scores compared to patients who failed NPPV (8 vs. 5, P=0.01). The primary diagnoses of patients who were successfully treated with NPPV and those who failed NPPV were not statistically different (P=0.45). Patients who were primarily intubated showed a higher percentage of ARDS/ALI compared to patients who failed NPPV (27.5% vs. 12.2%, P=0.001) and a lower percentage of cardiogenic pulmonary edema compared to patients successfully treated with NPPV (15.0% vs. 32.6%, P=0.04). No differences in comorbidities were detected among the three subsets of patients. The group that was successfully treated with NPPV showed a lower number of patients diagnosed with severe sepsis compared to patients with NPPV failure and patients primarily intubated (46.7% vs. 80.5% and 90.0% respectively, P<0.001). Patients who failed NPPV had significantly higher levels of plasma sodium and creatinine, and lower levels of plasma glucose, compared to patients with avoided intubation. Patients primarily intubated showed higher levels of lactate and albumin compared to the other groups. Patients treated successfully with NPPV showed significantly higher means of (peripheral) oxygen saturation, partial oxygen pressure and PF-ratio and lower fraction of inspired oxygen (FiO2) at the start of NPPV compared to patients who failed NPPV.
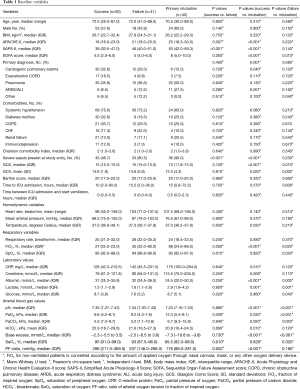
Full table
Table 2 shows the hemodynamic, respiratory and ventilatory variables after 1 hour of ventilation. Patients successfully treated with NPPV had significantly higher means of oxygen saturation and partial oxygen pressure after 1 hour of ventilation compared to both other groups. They also showed significantly lower rates of FiO2 and PF-ratio. The use of noradrenalin and sedative medications was significantly lower for patients treated successfully with NPPV compared to those who failed NPPV, P=0.05 and P=0.04, respectively. Patients primarily intubated showed the highest rates of noradrenalin and sedative medication use. Compared to patients who were primarily intubated, patients who failed NPPV had significantly lower ventilatory pressures.
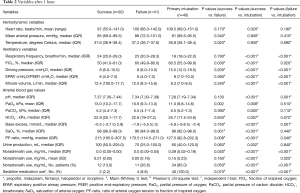
Full table
The delta values (Table 3) showed that patients treated successfully with NPPV had a significantly larger decline in partial carbon dioxide pressure. Contrarily, an increase of partial oxygen dioxide pressure was seen in patients who failed NPPV (P=0.04). In patients treated either successfully or unsuccessfully with NPPV an increase of PaO2 and oxygen saturation (SaO2) was shown, whereas only an increase in SaO2 was found in patients who were primarily intubated.
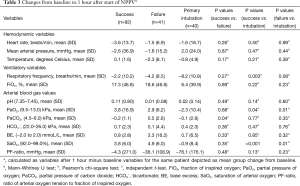
Full table
Predictors of NPPV failure
The baseline variables that were entered into the multivariate analysis included APACHE-II, SAPS-II and SOFA scores, the presence of sepsis at study entry, serum sodium, glucose, peripheral oxygen saturation (SpO2), PaO2, FiO2, SaO2 and PF-ratio. Multivariate logistic regression analysis identified four variables. Presence of sepsis at study entry was independently associated with NPPV failure, with an OR of 3.85 (95% CI: 1.38–10.76, P=0.01). Also SAPS-II (OR, 1.07, 95% CI: 1.02–1.12) and PF-ratio (OR, 0.995, 95% CI: 0.99–1.00) were identified as predictors of NPPV failure. Glucose was not significantly associated with NPPV failure, though showed a trend towards an association (OR, 0.83, 95% CI: 0.69–1.00, P=0.05).
Logistic analysis performed on variables recorded after 1 hour of ventilation showed that temperature (OR, 0.56, 95% CI: 0.34–0.91, P=0.02) and PF-ratio (OR, 0.99, 95% CI: 0.99–0.99, P=0.04) were independently associated with NPPV failure. Sedative medication only showed a trend towards an association with NPPV failure (OR, 10.16, 95% CI: 0.99–103.99, P=0.05).
As for the delta variables, only the delta value of PaCO2 was significantly different for patients with NPPV failure compared to NPPV success and was entered in a logistic regression model. This resulted in an OR of 1.32 (95% CI: 0.95–1.83), which did not show statistical significance (P=0.10).
Secondary outcome parameters
The (unadjusted) secondary outcomes are displayed in Table 4. The percentage of patients successfully treated with NPPV was 69.2%, with a survival rate of 96.7%, as depicted in Figure 1. Patients who failed NPPV showed a significantly lower survival rate of 65.9% (P<0.001). Patients intubated without a trial of NPPV showed a survival rate of 67.5%, not significantly different compared with the survival rate of patients who failed NPPV (P=0.88). Patients with NPPV success had significantly better outcomes compared with patients who failed NPPV and who were primarily intubated concerning ICU length of stay (P<0.001, P<0.001), incidence of ICU acquired pneumonia (P=0.005, P=0.040) and organ failure (P<0.001, P<0.001), respectively. Patients with NPPV failure showed shorter duration of noninvasive ventilation (median =7 days, IQR, 4–12) compared to patients with NPPV success (median =14, IQR, 6.3–135.0, P<0.001). Mortality rates were not significantly different among patients with NPPV failure ventilated noninvasively ≤7 hours (36.4%) versus >7 hours (46.2%, P=0.75). Hospital length of stay was not significantly different between NPPV success and failure (P=0.24), however a significant shorter hospital length of stay was found among NPPV success patients compared with the primary intubation group (P=0.02). Patients who failed NPPV compared with patients primarily intubated did not show any significant difference in the unadjusted outcome parameters length of ICU stay (P=0.51), length of hospital stay (0.37), rate of ICU acquired pneumonia (P=0.56), organ failure (P=0.27) and survival (P=0.88).
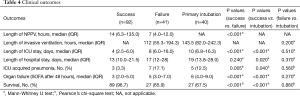
Full table
Table 5 depicts the OR of the outcome parameters of NPPV failure compared with NPPV success, adjusted for baseline differences. The adjusted OR show that NPPV failure is independently associated with a lower survival rate (OR, 0.10, 95% CI: 0.02–0.59, P=0.01) and a longer ICU length of stay (OR, 1.16, 95% CI: 1.04–1.30, P=0.01). Additionally, NPPV failure is associated with shorter length of noninvasive ventilation (OR, 0.91, 95% CI: 0.85–0.97, P=0.004). Length of hospital stay, organ failure and the incidence of ICU acquired pneumonia are not statistically different.
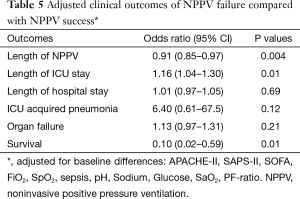
Full table
For patients who failed NPPV in comparison to patients who were primarily intubated, no differences in outcomes were found (Table 6).
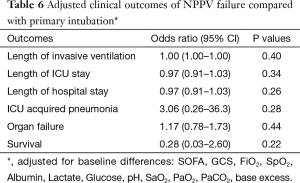
Full table
Discussion
This retrospective single-center study demonstrates that NPPV success, compared with NPPV failure, is independently associated with better survival and shorter ICU stay among patients admitted to the ICU for acute respiratory failure. These findings are consistent with prior research (18,20,31). In addition, a meta-analysis of 78 randomized trials supported the hypothesis that NPPV treatment improves survival in most acute settings when applied to treat or prevent acute respiratory failure (2).
Remarkably, our study found that patients with NPPV failure had predominantly similar adjusted outcomes compared to patients primarily intubated without a prior trial of NPPV. These outcomes could suggest there are no considerable risks involved due to delayed intubation after a NPPV trial. Length of noninvasive ventilation within 48 hours did not seem to affect mortality outcomes. An initial trial of NPPV therefore could be considered in patients with acute respiratory failure, since NPPV could be potentially beneficial and does not seem to result in worse outcome in case of failure of NPPV compared with primary intubation.
Previous studies addressing risks associated with NPPV failure show conflicting results. Our findings are in line with the results of subanalyses of two other trials, in which patients with NPPV failure did not show increased mortality compared to patients that were primarily intubated (8,31). On the other hand, Stefan and collaborators found that mortality rates in patients with COPD exacerbation who failed NPPV were substantially higher compared with patients who were primarily intubated (16.1% vs. 22.5%) (12). Similar results were observed by Chandra and coworkers (P<0.01) (24). An observational cohort study that evaluated the intubation-related complications in patients failing NPPV found increased odds of a composite of complications, which in turn was also associated with increased odds of death compared with patients intubated without a prior trial of NPPV (32). A large prospective trial is required to study risks in specific subgroups of patients, as it is likely that the effect with be different in patients with COPD and ARDS.
Logistic regression models of our study identified the presence of severe sepsis at study entry, a higher SAPS-II score, a lower PF-ratio and a lower plasma glucose at baseline as factors independently associated with NPPV failure (Table 4). These factors could be of predictive value in the selection process of patients eligible for NPPV. Particularly the presence of severe sepsis could be seen as a contra-indication for the start of NPPV, with an OR of NPPV failure of 3.85 (range, 1.38–10.76). Other studies have identified a large variety of predictors of NPPV failure. Antonelli also found that presence of sepsis at study entry was associated with increased NPPV failure rates (20). In numerous studies a higher SAPS-II score was related to NPPV failure, as well as APACHE-II and SOFA scores (19-21,23,24). A low baseline PF-ratio was found to be a risk factor of NPPV failure in several other studies (33,34).
Interestingly, NPPV failure was associated with significantly lower glucose levels in our study, which is in contrast to the results of Chakrabarti et al. They found that hyperglycemia upon presentation was associated with a poor outcome in patients with acute respiratory failure necessitating NPPV (35). A possible explanation of our findings may be that lower glucose levels represent lack of glycogen stores and more severe disease acuity with high consumption of glucose (36).
Our study did not identify several factors reported by other studies as independent predictors for NPPV failure, such as age, the presence of ARDS or community acquired pneumonia, low pH levels, increased respiratory rate, hypercapnia and poor neurologic scores, probably due to our local protocol excluding most of these patients from a NPPV trial (1,18-22).
The PF-ratio and use of sedative medication are predictors of NPPV failure after 1 hour of ventilation identified in our logistic regression analyses (Table 5). These factors could be useful in the decision-making process to continue NPPV to patients after 1 hour or not. The inability to improve the PF-ratio after 1 hour was also shown by Antonelli and collaborators to be an independent predictor of NPPV failure in patients with ARDS (37). In contrast to our findings, Muriel and coworkers did not find any apparent effect on outcome of monotherapy sedative medications. However, they did find that simultaneous use of analgesics and sedatives was associated with failure of NPPV (38).
Although temperature was also identified as a predictor, this variable is not considered as clinically relevant. Discrimination is limited as temperature differences measured in patients with NPPV success compared to NPPV failure are too small to be helpful in decision-making to interrupt NPPV.
The delta value of PaCO2 was significantly different for patients with NPPV failure compared to NPPV success in univariate analysis. In logistic regression analysis no significant predictive value was found (Table 6), although a deterioration of PaCO2 did show a trend towards association with NPPV failure (P=0.10). Anton and collaborators previously concluded that improvement of PaCO2 after 1 hour of NPPV was highly predictive for NPPV success in patients with COPD (39).
Our study has several strengths and limitations. First, many trials have been conducted to study outcomes of NPPV failure compared with NPPV success, as well as outcomes of NPPV with standard medical care or invasive ventilation. However, only few studies focused on outcomes in NPPV failure compared with immediate invasive ventilation.
The main limitation of this study is the retrospective nature of data collection. The groups of patients with NPPV and primary intubation are not fully comparable with respect to baseline variables. We addressed the imbalances by adjusting for significant baseline differences with respect to outcomes. Still, confounding by unknown factors and selection bias cannot be completely ruled out.
The small study population precludes subgroup analyses and extensive multivariate analysis due to the limited size of events (n=41). Non-significant trends could potentially have lead to significant results if larger number of patients were included. On the other hand, since this study investigated numerous variables with a 95% probability, factors could be found statistically significant by chance as a result of multiplicity.
Furthermore, we studied a heterogeneous population, since all patients with acute respiratory failure due to various causes were included. This may limit internal validity, however may increase external validity of results. Moreover, our study was conducted in a single experienced center with respect to practice of NPPV and this may limit the generalizability of findings. Since we investigated characteristics and outcomes of early NPPV failure (<48 hours), we cannot draw conclusions on possible “late” NPPV failure (>48 hours). Studies report an incidence of NPPV failure after 48 hours of around 10–20%, associated with a poor in-hospital prognosis (40). We did not address tolerance and patient comfort of the interfaces or the experience and skills of the staff involved, which are all key components to NPPV success (41). These factors were beyond the scope of our study.
Conclusions
The presence of severe sepsis at study entry, higher SAPS-II score, lower PF-ratio and lower plasma glucose at baseline were independently associated with NPPV failure. These factors could be helpful to select non-eligible patients for NPPV.
Patients with acute respiratory failure and NPPV failure have worse outcomes compared with NPPV success patients, however not worse than initially intubated patients. Therefore, an initial trial of NPPV may be considered in selected cases of acute respiratory failure patients in NPPV experienced medical centers, since NPPV could be potentially beneficial and does not seem to result in worse outcomes in case of NPPV failure compared with primary intubation. A prospective trial is warranted to confirm our findings.
Acknowledgements
None.
Footnote
Conflicts of Interest: The authors have no conflicts of interest to declare.
References
- Nava S, Hill N. Non-invasive ventilation in acute respiratory failure. Lancet 2009;374:250-9. [Crossref] [PubMed]
- Cabrini L, Landoni G, Oriani A, et al. Noninvasive ventilation and survival in acute care settings: a comprehensive systematic review and metaanalysis of randomized controlled trials. Crit Care Med 2015;43:880-8. [Crossref] [PubMed]
- Williams JW Jr, Cox CE, Hargett CW, et al. Noninvasive Positive-Pressure Ventilation (NPPV) for Acute Respiratory Failure [Internet]. Rockville (MD): Agency for Healthcare Research and Quality (US); 2012 Jul. Report No.: 12-EHC089-EF.
- Ram FS, Picot J, Lightowler J, et al. Non-invasive positive pressure ventilation for treatment of respiratory failure due to exacerbations of chronic obstructive pulmonary disease. Cochrane Database Syst Rev 2004.CD004104. [PubMed]
- Potts JM. Noninvasive positive pressure ventilation: Effect on mortality in acute cardiogenic pulmonary edema: A pragmatic metaanalysis. Pol Arch Med Wewn 2009;119:349-53. [PubMed]
- Weng CL, Zhao YT, Liu QH, et al. Meta-analysis: Noninvasive ventilation in acute cardiogenic pulmonary edema. Ann Intern Med 2010;152:590-600. [Crossref] [PubMed]
- Mehta S, Al-Hashim AH, Keenan SP. Noninvasive ventilation in patients with acute cardiogenic pulmonary edema. Respir Care 2009;54:186-95; discussion 195-7. [PubMed]
- Conti G, Antonelli M, Navalesi P, et al. Noninvasive vs. conventional mechanical ventilation in patients with chronic obstructive pulmonary disease after failure of medical treatment in the ward: a randomized trial. Intensive Care Med 2002;28:1701-7. [Crossref] [PubMed]
- Antonelli M, Conti G, Rocco M, et al. A comparison of noninvasive positive-pressure ventilation and conventional mechanical ventilation in patients with acute respiratory failure. N Engl J Med 1998;339:429-35. [Crossref] [PubMed]
- Honrubia T, García López FJ, Franco N, et al. Noninvasive vs conventional mechanical ventilation in acute respiratory failure: a multicenter, randomized controlled trial. Chest 2005;128:3916-24. [Crossref] [PubMed]
- Tsai CL, Lee WY, Delclos GL, et al. Comparative effectiveness of noninvasive ventilation vs invasive mechanical ventilation in chronic obstructive pulmonary disease patients with acute respiratory failure. J Hosp Med 2013;8:165-72. [Crossref] [PubMed]
- Stefan MS, Nathanson BH, Higgins TL, et al. Comparative Effectiveness of Noninvasive and Invasive Ventilation in Critically Ill Patients With Acute Exacerbation of Chronic Obstructive Pulmonary Disease. Crit Care Med 2015;43:1386-94. [Crossref] [PubMed]
- Agarwal R, Aggarwal AN, Gupta D. Role of noninvasive ventilation in acute lung injury/acute respiratory distress syndrome: a proportion meta-analysis. Respir Care 2010;55:1653-60. [PubMed]
- Agarwal R, Aggarwal AN, Gupta D, et al. Role of noninvasive positive-pressure ventilation in postextubation respiratory failure: a meta-analysis. Respir Care 2007;52:1472-9. [PubMed]
- Pallin M, Naughton MT. Noninvasive ventilation in acute asthma. J Crit Care 2014;29:586-93. [Crossref] [PubMed]
- Murad A, Li PZ, Dial S, et al. The role of noninvasive positive pressure ventilation in community-acquired pneumonia. J Crit Care 2015;30:49-54. [Crossref] [PubMed]
- Keenan SP, Sinuff T, Burns KE, et al. Clinical practice guidelines for the use of noninvasive positive-pressure ventilation and noninvasive continuous positive airway pressure in the acute care setting. CMAJ 2011;183:E195-214. [Crossref] [PubMed]
- Schnell D, Timsit JF, Darmon M, et al. Noninvasive mechanical ventilation in acute respiratory failure: trends in use and outcomes. Intensive Care Med 2014;40:582-91. [Crossref] [PubMed]
- Ozyilmaz E, Ugurlu AO, Nava S. Timing of noninvasive ventilation failure: causes, risk factors, and potential remedies. BMC Pulm Med 2014;14:19. [Crossref] [PubMed]
- Antonelli M, Conti G, Moro ML, et al. Predictors of failure of noninvasive positive pressure ventilation in patients with acute hypoxemic respiratory failure: a multi-center study. Intensive Care Med 2001;27:1718-28. [Crossref] [PubMed]
- Pacilli AM, Valentini I, Carbonara P, et al. Determinants of noninvasive ventilation outcomes during an episode of acute hypercapnic respiratory failure in chronic obstructive pulmonary disease: the effects of comorbidities and causes of respiratory failure. Biomed Res Int 2014;2014:976783.
- Hess DR. Noninvasive ventilation for acute respiratory failure. Respir Care 2013;58:950-72. [Crossref] [PubMed]
- Mosier JM, Sakles JC, Whitmore SP, et al. Failed noninvasive positive-pressure ventilation is associated with an increased risk of intubation-related complications. Ann Intensive Care 2015;5:4. [Crossref] [PubMed]
- Chandra D, Stamm JA, Taylor B, et al. Outcomes of noninvasive ventilation for acute exacerbations of chronic obstructive pulmonary disease in the United States, 1998-2008. Am J Respir Crit Care Med 2012;185:152-9. [PubMed]
- Horan TC, Andrus M, Dudeck MA. CDC/NHSN surveillance definition of health care-associated infection and criteria for specific types of infections in the acute care setting. Am J Infect Control 2008;36:309-32. [Crossref] [PubMed]
- ARDS Definition Task Force, Ranieri VM, Rubenfeld GD, et al. Acute respiratory distress syndrome: the Berlin Definition. JAMA 2012;307:2526-33. [PubMed]
- Pauwels RA, Buist AS, Ma P, et al. Global strategy for the diagnosis, management, and prevention of chronic obstructive pulmonary disease: National Heart, Lung, and Blood Institute and World Health Organization Global Initiative for Chronic Obstructive Lung Disease (GOLD): executive summary. Respir Care 2001;46:798-825. [PubMed]
- The Criteria Committee of the New York Heart Association. Nomenclature and criteria for diagnosis of diseases of the heart and blood vessels. Boston: Little Brown, 1964.
- Minimal dataset NICE. Nationale Intensive Care Evaluatie. Accessed Jul 15, 2015. Available online: www. stichting-nice.nl/datainbeeld/public
- Charlson ME, Pompei P, Ales KL, et al. A new method of classifying prognostic comorbidity in longitudinal studies: development and validation. J Chronic Dis 1987;40:373-83. [Crossref] [PubMed]
- Demoule A, Girou E, Richard JC, et al. Benefits and risks of success or failure of noninvasive ventilation. Intensive Care Med 2006;32:1756-65. [Crossref] [PubMed]
- Thille AW, Contou D, Fragnoli C, et al. Non-invasive ventilation for acute hypoxemic respiratory failure: intubation rate and risk factors. Crit Care 2013;17:R269. [Crossref] [PubMed]
- Agarwal R, Handa A, Aggarwal AN, et al. Outcomes of noninvasive ventilation in acute hypoxemic respiratory failure in a respiratory intensive care unit in north India. Respir Care 2009;54:1679-87. [PubMed]
- Carron M, Freo U, Zorzi M, Ori C. Predictors of failure of noninvasive ventilation in patients with severe community-acquired pneumonia. J Crit Care 2010;25:540.e9-14.
- Chakrabarti B, Angus RM, Agarwal S, et al. Hyperglycaemia as a predictor of outcome during non-invasive ventilation in decompensated COPD. Thorax 2009;64:857-62. [Crossref] [PubMed]
- de Jong MF, Beishuizen A, Spijkstra JJ, et al. Predicting a low cortisol response to adrenocorticotrophic hormone in the critically ill: a retrospective cohort study. Crit Care 2007;11:R61. [Crossref] [PubMed]
- Antonelli M, Conti G, Esquinas A, et al. A multiple-center survey on the use in clinical practice of noninvasive ventilation as a first-line intervention for acute respiratory distress syndrome. Crit Care Med 2007;35:18-25. [Crossref] [PubMed]
- Muriel A, Peñuelas O, Frutos-Vivar F, et al. Impact of sedation and analgesia during noninvasive positive pressure ventilation on outcome: a marginal structural model causal analysis. Intensive Care Med 2015;41:1586-600. [Crossref] [PubMed]
- Antón A, Güell R, Gómez J, et al. Predicting the result of noninvasive ventilation in severe acute exacerbations of patients with chronic airflow limitation. Chest 2000;117:828-33. [Crossref] [PubMed]
- Moretti M, Cilione C, Tampieri A, et al. Incidence and causes of non-invasive mechanical ventilation failure after initial success. Thorax 2000;55:819-25. [Crossref] [PubMed]
- Holanda MA, Reis RC, Winkeler GF, et al. Influence of total face, facial and nasal masks on short-term adverse effects during noninvasive ventilation. J Bras Pneumol 2009;35:164-73. [Crossref] [PubMed]