Preventive and therapeutic effects of green tea on lung cancer: a narrative review of evidence from clinical and basic research
Introduction
Tea is one of the most popular beverages worldwide (1). It is made from the leaves of Camellia sinensis. According to the handling methods after the leaves are picked, tea can be categorized into 3 types: green tea, black tea, and oolong tea (2). The handling methods for green tea involve steaming or pan-frying tea leaves, while black tea involves rolling and fermenting (3). The steaming and pan-frying prevent the oxidation of polyphenols, while rolling and fermenting do not. Therefore, green tea has a much higher amount of polyphenols than does black tea (4). Catechin is the primary type of polyphenol and has numerous health-promoting properties (4). In addition, other chemical constituents of green tea, such as amino acids, vitamins, and trace elements, also have health-promoting properties. During the past year, numerous preclinical and clinical studies indicated that green tea exerts preventive effects on various types of cancer, including lung cancer, gastric cancer, and prostate cancer (5). Here, we reviewed the association between green tea consumption and lung cancer. We present the following article in accordance with the Narrative Review reporting checklist (available at https://jtd.amegroups.com/article/view/10.21037/jtd-22-1791/rc).
Methods
To perform this narrative review, we searched the PubMed database to screen scientific literature concerning lung cancer and green tea. All clinical trials, observational studies, animal studies and basic researches published between January 1st, 1980 to August 1st, 2022 were considered. The search was performed till August 1st, 2022. The following search terms were used: (“green tea” or polyphenol or “Epigallocatechin-3-gallate” or egcg or “epigallocatechin gallate”[nm] or “Catechin”[nm]) and (“lung cancer” or “Lung Neoplasms”[mesh]). We only considered research articles written in English. Three authors (ZD Hu, YJ Wang, L Zhang) read the potential studies and wrote the draft. The search strategy was summarized in Table 1.
Table 1
Items | Specification |
---|---|
Date of search | August 1st, 2022 |
Databases and other sources searched | PubMed |
Search terms used | (“green tea” or polyphenol or “Epigallocatechin-3-gallate” or egcg or “epigallocatechin gallate”[nm] or “Catechin”[nm]) and (“lung cancer” or “Lung Neoplasms”[mesh]) |
Timeframe | From January 1st, 1980 to August 1st, 2022 |
Inclusion and exclusion criteria | Clinical trials, observational studies, animal studies and basic researches were included. Only articles published in English were included |
Selection process | Three authors selected studies together |
Overview of findings
Green tea consumption and lung cancer risk
The first study to investigate lung cancer risk and green tea consumption was published in 1988 (6). In that prospective cohort study, tea consumption was surprisingly associated with increased lung cancer risk (6). However, the subsequent cohort and case-control studies indicated that green tea consumption decreased lung cancer risk (7-9). A meta-analysis indicated that consumption of green tea, but not black tea, was associated with decreased lung cancer risk [risk ratio (RR) 0.78; 95% confidence interval (CI): 0.60–1.00] (10). However, subgroup analyses did not support the association between green tea consumption and lung cancer risk. Notably, the estimated RR of green tea is <1.00, but its 95% CI is 1.00, indicating that green tea’s effect on lung cancer risk is not statistically significant. This may be because only 12 studies investigated green tea in this meta-analysis, and considerable heterogeneity was observed (I2=78.5%). Therefore, further studies are needed to address the association between tea consumption and lung cancer risk.
In 2014, an updated meta-analysis was published (2). By pooling 26 case-control studies and 12 cohort controls, this meta-analysis revealed that tea consumption decreased lung cancer risk, with an RR of 0.78 (95% CI: 0.70–0.87). In subgroup analysis, both green tea and black tea were associated with decreased lung cancer risk. However, the study design (case-control or cohort) and the sex of the participants can affect the strength of the association between tea consumption and lung cancer risk. In a most recently published meta-analysis (11), tea consumption was proven to be associated with decreased lung cancer risk (odds ratio 0.80; 95% CI: 0.73–0.87). Consumption of 7.5 g of green tea a day can significantly decrease lung cancer risk, as demonstrated by both case-control studies and cohort studies. In 2020, a meta-analysis performed by Cochrane group analyzed the relationship between green tea and cancer (12). They concluded that green tea may have a small preventive effect for lung cancer, but there is possible risk of bias due to case-control studies (12).
The above-mentioned meta-analyses, which pooled the results of both case-control and cohort studies, indicate that green tea consumption can decrease lung cancer risk. These meta-analyses also revealed that great heterogeneity exists across the available studies. Indeed, the participants in available studies varied. For example, some studies included only nonsmokers (13) or heavy smokers (14). In addition, the confounding factors adjusted in each eligible study are variable. These design characteristics are possible sources of heterogeneity among available studies. The association between lung cancer risk and tea consumption may be affected by confounding factors, such as smoking status, gender, dietary habit, and economic status. For example, in a recently published cohort study with a large sample size (n=455,981) (15), green tea consumption was found to only decrease lung cancer risk in smokers and excessive alcohol consumers. Therefore, further studies with large sample sizes are needed to address the clinical factors affecting the association between tea consumption and lung cancer risk.
Notably, the majority of eligible studies categorize participants into several groups with a prespecified threshold. This design characteristic may complicate the clinical explanation of the pooled results of eligible studies with different categories. Under this condition, a dose-response meta-analysis is a good choice for pooling the findings of available studies. Indeed, one dose-response meta-analysis revealed a nonlinear relationship between green tea consumption and lung cancer risk (16), with individuals who consume 7 cups/day of green tea having a significantly decreased risk of lung cancer.
Theabrownin
Theabrownin is an active pigment of green tea that can determine the color and taste of green tea. In vitro studies indicated that it could promote apoptosis (17-19), induce cell cycle arrest (20), and inhibit migration (18), clone formation (19), and proliferation (17,19,20) of lung cancer cell lines in a dose-dependent manner. In vivo studies indicated that it could inhibit the growth of tumors in xenograft zebrafish (18) or mice (20). The expressions of several genes were dysregulated after theabrownin treatment, such as p53 (17), Bax (17,18), TOPO 1/2 (17), and Bcl-2 (17,18), suggesting that the antitumor effect of theabrownin is associated with these molecules. It is hypothesized that the apoptosis-inducing effect of theabrownins is mediated by the p53-mediated caspase-dependent pathway (17,18). Theabrownin-induced cell cycle arrest may be mediated by C-myc and its downstream molecules (20). Further studies are needed to validate this hypothesis.
Autophagy is a crucial physiological procedure that enhances cell survival. It is involved in the emergence and development of cancer (21). The formation of double-membrane vesicles (termed autophagosomes) is an initial step of autophagy. The autophagosome captures the cytosolic cargo and transports it to the lysosomes, where the cargo is degraded. The degradation product is subsequently recycled back to the cytoplasm. This procedure is known as autophagic flux (22). In vitro studies indicated that theabrownin can suppress PI3K/Akt/mTOR pathway and thus promote autophagic flux, leading to the accumulation of autophagosomes in lung cancer cells (19). Inhibition of autophagy partially impairs the antiproliferative and proapoptotic activities of theabrownin (19), indicating that the antitumor effect of theabrownin is partially mediated by autophagy.
(−)-Epigallocatechin gallate (EGCG)
Proliferation and apoptosis
As mentioned above, catechin is the primary type of polyphenol and has anticancer properties. Green tea has 4 types of catechin including EGCG, (−)-epigallocatechin (EGC), (−)-epicatechin gallate (ECG), and (−)-epicatechin (EC) (23-25). In vitro studies indicated that EGCG could inhibit proliferation (24,26-28) and promote the apoptosis (26,29) of lung cancer cells, indicating its antitumor activity. In vivo studies also found that EGCG could inhibit the growth of lung cancer (26,30-32). With long noncoding RNA (lncRNA) microarray or next-generation sequencing (NGS), 960 differentially expressed lncRNAs, 1,434 mRNAs, and >100 microRNAs were identified in EGCG-treated lung cancer cells (28,33). Bioinformatic analysis indicates that many pathways are involved in the antitumor activity of EGCG (28), especially MAPK pathway (33).
There are many mechanisms involved in the antitumor activity of EGCG. First, EGCG suppresses the activity of lung cancer stem cells (LCSCs). LCSCs represent a minor but significant population of lung cancer cells that have the ability of multipotent differentiation and self-renewal. LCSCs are thus important for lung cancer metastasis and chemotherapy resistance (34). The activation of the canonical Wnt/β-catenin pathway is critically involved in the maintenance of LCSCs (35). EGCG inactivates the canonical Wnt/β-catenin pathway by decreasing GSK-3β phosphorylation, expression of β-catenin, and its downstream target gene C-Myc (36). These effects may be partially mediated by clock circadian regulator (CLOCK), a protein that regulates circadian rhythm (31). In addition, EGCG demethylates the promoter of Wnt inhibitory factor-1 (WIF-1) and increases its expression (37). Because WIF-1 is an inhibitor of Wnt signaling through direct binding to Wnt (38), increased WIF-1 expression can inactivate the Wnt/β-catenin pathway. However, it remains unknown whether WIF-1 mediates the stemness inhibitive effect of EGCG. In addition to the Wnt/β-catenin pathway, some other molecules can also mediate the inhibitive effective activity of EGCG on stemness, such as AXL receptor tyrosine kinase (AXL) (39) and miR-485-5p (30). EGCG upregulates the expression of miR-485-5p in lung cancer cells and inhibits the stemness by targeting retinoid X receptor-α (RXRα) (30) or CD44 (26).
Second, EGCG regulates the function of lung cancer cells through microRNAs (40). The expression of some microRNAs in lung cancer cells can be altered by EGCG (40), such as miR-210 (41) let-7a-1 (42), and let-7g (42). Green tea catechins upregulate the expression and let-7a-1 and let-7g, resulting in the decreased expression of 2 oncogenes, C-MYC and LIN-28 (42). In addition, EGCG directly binds to hypoxia-inducible factors alpha (HIFα), preventing HIFα from degradation and thus increasing its activity (41). HIFα upregulates the expression of miR-210 by binding to the hypoxia-response element (HRE) in the miR-210 promoter (41). Increased miR-210 promotes apoptosis and inhibits the proliferation of lung cancer cells (41). However, the target of miR-210 that mediates this procedure remains unknown. In addition, EGCG has been found to decrease the expression of miR-98 and thus increase the sensitivity of lung cancer cells to cisplatin (43,44).
Third, EGCG promotes lung cancer cell apoptosis by targeting the p53 pathway. P53 is a crucial tumor suppressor, and decreased p53 is frequently observed in the development of various cancers (45). Its activation is regulated by multiple posttranslational modifications, such as ubiquitination, phosphorylation, and acetylation (46). Phosphorylated p53 has increased activity and promotes the expression of its target genes (46). EGCG has been shown to induce the expression of p53 (47,48), inhibit the interaction between p53 and mouse double minute 2 (MDM2), and thus inhibit the p53 ubiquitination mediated by MDM2 (49). Furthermore, EGCG increases the p53 Ser15 and Ser20 phosphorylation and enhances its transcriptional activity (47,49).
Fourth, EGCG can inhibit 2 receptor tyrosine kinases, the epidermal growth factor receptor (EGFR) and C-Met, by inhibiting the phosphorylation of mTOR, STAT3, EGFR (Y1068), C-Met, AKT, S6, ERK1/2, and p38 (29,50); the expression and nuclear localization of EGFR; and its downstream target, cyclin D (50).
In addition, several other pathways also mediate the antiproliferation and proapoptosis of lung cancer. For example, EGCG was found to upregulate Ku70 acetylation and downregulate the interaction of Bax-Ku70 (32,51). Because the interaction of Ku70 and Bax is essential for cell survival, the separation of Ku70 and Bax can lead to lung cancer cell apoptosis (52). EGCG can directly interact with Ras-GAP SH3 domain-binding protein 1 (G3BP1) and suppress the binding of G3BP1 and Ras-GAP (53). The interaction of EGCG and G3BP1 can lead to the inactivation of Ras downstream targets (e.g., MEK and ERK), decreasing the proliferation of cancer cells (53). Both in vivo and in vitro studies have found that treatment of EGCG causes the generation of intracellular reactive oxygen species (ROS) and mitochondrial ROS (54). EGCG-induced ROS causes DNA oxidative damage and apoptosis (54). EGCG also inhibits lung cancer cell metastasis by suppressing matrix metalloproteinase 2 (MMP2) expression via the JNK pathway (55). In addition, telomerase (56), Bcl-2 (48), and B-cell lymphoma-extra large (Bcl-xl) (24,32,51) also mediate the inhibitive effect of EGCG on lung cancer proliferation and apoptosis.
Epithelial-mesenchymal transition (EMT)
EMT is characterized by a loss of epithelial phenotype and a gain of mesenchymal fibroblastic phenotype in polarized epithelial cells (57). This process can increase the metastatic and invasive potential of a cancer cell. Some studies indicated that ECGC could inhibit the EMT process of lung cancer cell lines. Transforming growth factor-β (TGF-β) is the primary mediator of EMT in lung cancer cells. Several in vitro studies indicated that EGCG could inhibit the EMT induced by TGF-β in lung cancer cell lines (58,59). Specifically, EGCG inhibits the expression of mesenchymal markers such as vimentin (59-61) and N-cadherin (59) while increasing the expression of epithelial marker E-cadherin (58,59) at the transcriptional level. The inhibitive effect of EGCG on EMT decreases the migration and invasion of lung cancer cells (58-61). There are some molecular mechanisms underlying the EMT-inhibitive effect of EGCG. For example, EGCG inhibits the phosphorylation of Smad2 (58) and ERK1/2 (58) or the expression of transcription factors including Snail (58,59), ZEB1 (58), Twist (58), and Slug (58,59,61). In addition, EGCG inhibits the expression of p300 and CBP, thus decreasing their ability to acetylate Smad2 and Smad3 (59). Because the nucleus translocation of acetylated Smad2 and Smad3 is a critical step in TGF-β-induced expression of EMT-related genes (62), the inhibitive effect of EGCG on Smad2 and Smad3 acetylation downregulates the expression of EMT target genes.
Angiogenesis
Angiogenesis is necessary for tumor cell growth, and suppressing angiogenesis is a treatment approach for various solid cancers. Hypoxia occurs in the growing tumor tissue and can promote the expression and release of hypoxia-inducible factors-1α (HIF-1α) and HIF-2α from cancer cells (63). HIF-1α and HIF-2α upregulate the expression of vascular endothelial growth factor (VEGF), which binds to the VEGF receptors (VEGFR) in endothelial cells and enhances angiogenesis (64). EGCG has been proven to inhibit the expression of HIF-1α in lung cancer in vitro (65) and in vivo (66). In in vitro studies, EGCG decreases the expression of HIF-1α induced by nicotine (60), human papillomavirus (HPV)-16 oncoprotein (66), or insulin-like growth factor 1 (IGF-1) (67). In addition, EGCG upregulates the expression of endostatin, a potent endogenous angiogenesis inhibitor (65). Decreased HIF-1α impairs the production of VEGF in the lung cancer cell and thus inhibits angiogenesis (65-67). In addition to HIF-1α/VEGF pathway, EGCG can rebalance angiopoietin-1 and angiopoietin-2 (68) and induce vascular normalization (69), thus relieving the hypoxia status of lung cancer cell and improving the efficacy of the chemotherapeutic agent (68,69).
Sensitizing lung cancer cells to cisplatin
Cisplatin is a conventional chemotherapy agent for patients with advanced non-small cell lung cancer (NSCLC), but cisplatin resistance occurs in some patients (70). The mechanisms underlying cisplatin resistance are complex, and many pathways or molecules are involved. Copper transporter 1 (CTR1, also known as SLC31A1) is a copper influx transporter that promotes cisplatin internalization in cancer cells. The decreased expression of CTR1 is associated with cisplatin resistance.
EGCG can upregulate the expression of CTR1 and thus enhance the cisplatin sensitivity in vivo and in vitro (44). Specifically, EGCG has been shown to increase the expression of NEAT1 by increasing ROS generation (71). NEAT1 decreases the expression of miR-98 (44). Because CTR1 is a target of miR-98 in lung cancer cells, decreased miR-98 downregulates the expression of CTR1 and sensitizes lung cancer cells to cisplatin (44). In addition, decreased miR-98 results in increased p53, which activates the p53-dependent apoptosis pathway and enhances the effect of cisplatin (43).
In addition to the ROS-NEAT1-CTR1 axis, an additional 3 pathways have been reported to be involved in the sensitizing of lung cancer cells to cisplatin. First, proteomic analysis revealed that hepatoma-derived growth factor (HDGF) mediates the anticancer effects of EGCG (72). EGCG enhances cisplatin-induced apoptosis by suppressing HDGF (72). Second, EGCG can downregulate the expression of 2 TAM receptors (Tyro3, Axl, and MerTK), Axl and Typo3 (73). Because the TAM receptors are critically involved in the progression of lung cancer (74), the decreased expression of Axl and Typo3 may cause cisplatin resistance. Third, by inhibiting the activity of DNA methyltransferase (DNMT) and histone deacetylase, EGCG upregulates the expression of some tumor suppressors (e.g., GAS1, TIMP4, ICAM1, and WISP2) and can partially reverse cisplatin resistance (75).
Sensitizing lung cancer cells to tyrosine kinase inhibitors (TKIs)
Activating EGFR pathway plays a critical role in the development and occurrence of lung cancer. EGFR is a member of the receptor tyrosine kinase (RTKs), and TKIs, such as gefitinib and erlotinib, have been recommended as the first-line treatment in NSCLC patients with the EGFR mutation. However, acquired resistance occurs after long-term exposure to gefitinib (76). EMT is a possible mechanism underlying TKI resistance. In gefitinib-resistant lung cancer cells, EMT can be caused by activation of the PI3K/Akt/mTOR pathway. An EGCG derivate can sensitize the chemosensitivity of gefitinib by inhibiting the phosphorylation of PI3K and Akt (77). In addition to EGFR, EGCG can also decrease the expression of C-MET, another type of RTK (78).
Conclusions
As shown in Figure 1, accumulating observational studies support that green tea consumption can decrease lung cancer risk. In vitro studies also demonstrated that EGCG and theabrownin can inhibit the growth, EMT, stemness, and angiogenesis of lung cancers and promote apoptosis. In a lung cancer animal model, EGCG also showed anticancer activity. In a phase I trial of patients with locally advanced stage III NSCLC who received chemotherapy (cisplatin and etoposide) and radiation, oral administration of EGCG did not cause dose-limiting toxicities and could reduce the pain score of patients (79). Interestingly, dramatic regression of esophagitis to grade 0–1 was observed in >90% of the patients (79). These results indicate that EGCG is safe and effective in improving lung cancer patients’ quality. Notably, most of the clinical evidence comes from observational studies, and little evidence based on randomized clinical trial. Further studies with high level of evidence are needed to investigate the long-term efficacy and safety of oral administration of EGCG in lung cancer patients. In addition, some studies also revealed that the derivates of EGCG also exert anti-lung cancer activity (25,80,81). The anti-lung cancer activity of these derivates needs to be investigated by further studies.
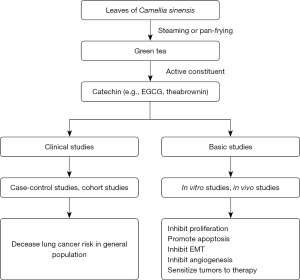
Acknowledgments
Funding: This work is supported by the National Natural Science Foundation of China (No. 81860501) and the Natural and Science Foundation of Inner Mongolia Autonomous Region for Distinguished Young Scholars (No. 2020JQ07).
Footnote
Reporting Checklist: The authors have completed the Narrative Review reporting checklist. Available at https://jtd.amegroups.com/article/view/10.21037/jtd-22-1791/rc
Conflicts of Interest: All authors have completed the ICMJE uniform disclosure form (available at https://jtd.amegroups.com/article/view/10.21037/jtd-22-1791/coif). The authors have no conflicts of interest to declare.
Ethical Statement: The authors are accountable for all aspects of the work in ensuring that questions related to the accuracy or integrity of any part of the work are appropriately investigated and resolved.
Open Access Statement: This is an Open Access article distributed in accordance with the Creative Commons Attribution-NonCommercial-NoDerivs 4.0 International License (CC BY-NC-ND 4.0), which permits the non-commercial replication and distribution of the article with the strict proviso that no changes or edits are made and the original work is properly cited (including links to both the formal publication through the relevant DOI and the license). See: https://creativecommons.org/licenses/by-nc-nd/4.0/.
References
- Graham HN. Green tea composition, consumption, and polyphenol chemistry. Prev Med 1992;21:334-50. [Crossref] [PubMed]
- Wang L, Zhang X, Liu J, et al. Tea consumption and lung cancer risk: a meta-analysis of case-control and cohort studies. Nutrition 2014;30:1122-7. [Crossref] [PubMed]
- Kim TL, Jeong GH, Yang JW, et al. Tea Consumption and Risk of Cancer: An Umbrella Review and Meta-Analysis of Observational Studies. Adv Nutr 2020;11:1437-52. [Crossref] [PubMed]
- Musial C, Kuban-Jankowska A, Gorska-Ponikowska M. Beneficial Properties of Green Tea Catechins. Int J Mol Sci 2020;21:1744. [Crossref] [PubMed]
- Yuan JM. Cancer prevention by green tea: evidence from epidemiologic studies. Am J Clin Nutr 2013;98:1676S-81S. [Crossref] [PubMed]
- Kinlen LJ, Willows AN, Goldblatt P, et al. Tea consumption and cancer. Br J Cancer 1988;58:397-401. [Crossref] [PubMed]
- Hirvonen T, Virtamo J, Korhonen P, et al. Flavonol and flavone intake and the risk of cancer in male smokers (Finland). Cancer Causes Control 2001;12:789-96. [Crossref] [PubMed]
- Zheng W, Doyle TJ, Kushi LH, et al. Tea consumption and cancer incidence in a prospective cohort study of postmenopausal women. Am J Epidemiol 1996;144:175-82. [Crossref] [PubMed]
- Axelsson G, Liljeqvist T, Andersson L, et al. Dietary factors and lung cancer among men in west Sweden. Int J Epidemiol 1996;25:32-9. [Crossref] [PubMed]
- Tang N, Wu Y, Zhou B, et al. Green tea, black tea consumption and risk of lung cancer: a meta-analysis. Lung Cancer 2009;65:274-83. [Crossref] [PubMed]
- Guo Z, Jiang M, Luo W, et al. Association of Lung Cancer and Tea-Drinking Habits of Different Subgroup Populations: Meta-Analysis of Case-Control Studies and Cohort Studies. Iran J Public Health 2019;48:1566-76.
- Filippini T, Malavolti M, Borrelli F, et al. Green tea (Camellia sinensis) for the prevention of cancer. Cochrane Database Syst Rev 2020;3:CD005004. [Crossref] [PubMed]
- Liang D, Wang J, Li D, et al. Lung Cancer in Never-Smokers: A Multicenter Case-Control Study in North China. Front Oncol 2019;9:1354. [Crossref] [PubMed]
- Gnagnarella P, Maisonneuve P, Bellomi M, et al. Red meat, Mediterranean diet and lung cancer risk among heavy smokers in the COSMOS screening study. Ann Oncol 2013;24:2606-11. [Crossref] [PubMed]
- Li X, Yu C, Guo Y, et al. Association between tea consumption and risk of cancer: a prospective cohort study of 0.5 million Chinese adults. Eur J Epidemiol 2019;34:753-63. [Crossref] [PubMed]
- Wang Y, Yu X, Wu Y, et al. Coffee and tea consumption and risk of lung cancer: a dose-response analysis of observational studies. Lung Cancer 2012;78:169-70. [Crossref] [PubMed]
- Wu F, Zhou L, Jin W, et al. Anti-Proliferative and Apoptosis-Inducing Effect of Theabrownin against Non-small Cell Lung Adenocarcinoma A549 Cells. Front Pharmacol 2016;7:465. [Crossref] [PubMed]
- Xiao X, Guo L, Dai W, et al. Green tea-derived theabrownin suppresses human non-small cell lung carcinoma in xenograft model through activation of not only p53 signaling but also MAPK/JNK signaling pathway. J Ethnopharmacol 2022;291:115167. [Crossref] [PubMed]
- Wang Y, Yuan Y, Wang C, et al. Theabrownins Produced via Chemical Oxidation of Tea Polyphenols Inhibit Human Lung Cancer Cells in vivo and in vitro by Suppressing the PI3K/AKT/mTOR Pathway Activation and Promoting Autophagy. Front Nutr 2022;9:858261. [Crossref] [PubMed]
- Zhou L, Wu F, Jin W, et al. Theabrownin Inhibits Cell Cycle Progression and Tumor Growth of Lung Carcinoma through c-myc-Related Mechanism. Front Pharmacol 2017;8:75. [Crossref] [PubMed]
- Singh SS, Vats S, Chia AY, et al. Dual role of autophagy in hallmarks of cancer. Oncogene 2018;37:1142-58. [Crossref] [PubMed]
- Zhang XJ, Chen S, Huang KX, et al. Why should autophagic flux be assessed? Acta Pharmacol Sin 2013;34:595-9. [Crossref] [PubMed]
- Suganuma M, Takahashi A, Watanabe T, et al. Biophysical Approach to Mechanisms of Cancer Prevention and Treatment with Green Tea Catechins. Molecules 2016;21:1566. [Crossref] [PubMed]
- Sonoda JI, Ikeda R, Baba Y, et al. Green tea catechin, epigallocatechin-3-gallate, attenuates the cell viability of human non-small-cell lung cancer A549 cells via reducing Bcl-xL expression. Exp Ther Med 2014;8:59-63. [Crossref] [PubMed]
- Wang J, Sun P, Wang Q, et al. (-)-Epigallocatechin-3-gallate derivatives combined with cisplatin exhibit synergistic inhibitory effects on non-small-cell lung cancer cells. Cancer Cell Int 2019;19:266. [Crossref] [PubMed]
- Jiang P, Xu C, Chen L, et al. EGCG inhibits CSC-like properties through targeting miR-485/CD44 axis in A549-cisplatin resistant cells. Mol Carcinog 2018;57:1835-44. [Crossref] [PubMed]
- Pan T, Han D, Xu Y, et al. LC-MS Based Metabolomics Study of the Effects of EGCG on A549 Cells. Front Pharmacol 2021;12:732716. [Crossref] [PubMed]
- Hu DL, Wang G, Yu J, et al. Epigallocatechin-3-gallate modulates long non-coding RNA and mRNA expression profiles in lung cancer cells. Mol Med Rep 2019;19:1509-20. [Crossref] [PubMed]
- Minnelli C, Cianfruglia L, Laudadio E, et al. Effect of Epigallocatechin-3-Gallate on EGFR Signaling and Migration in Non-Small Cell Lung Cancer. Int J Mol Sci 2021;22:11833. [Crossref] [PubMed]
- Jiang P, Xu C, Chen L, et al. Epigallocatechin-3-gallate inhibited cancer stem cell-like properties by targeting hsa-mir-485-5p/RXRα in lung cancer. J Cell Biochem 2018;119:8623-35. [Crossref] [PubMed]
- Jiang P, Xu C, Zhang P, et al. Epigallocatechin-3-gallate inhibits self-renewal ability of lung cancer stem-like cells through inhibition of CLOCK. Int J Mol Med 2020;46:2216-24. [Crossref] [PubMed]
- Li JJ, Gu QH, Li M, et al. Role of Ku70 and Bax in epigallocatechin-3-gallate-induced apoptosis of A549 cells in vivo. Oncol Lett 2013;5:101-6. [Crossref] [PubMed]
- Bhardwaj V, Mandal AKA. Next-Generation Sequencing Reveals the Role of Epigallocatechin-3-Gallate in Regulating Putative Novel and Known microRNAs Which Target the MAPK Pathway in Non-Small-Cell Lung Cancer A549 Cells. Molecules 2019;24:368. [Crossref] [PubMed]
- Heng WS, Gosens R, Kruyt FAE. Lung cancer stem cells: origin, features, maintenance mechanisms and therapeutic targeting. Biochem Pharmacol 2019;160:121-33. [Crossref] [PubMed]
- Zhang Y, Wang X. Targeting the Wnt/β-catenin signaling pathway in cancer. J Hematol Oncol 2020;13:165. [Crossref] [PubMed]
- Zhu J, Jiang Y, Yang X, et al. Wnt/β-catenin pathway mediates (-)-Epigallocatechin-3-gallate (EGCG) inhibition of lung cancer stem cells. Biochem Biophys Res Commun 2017;482:15-21. [Crossref] [PubMed]
- Gao Z, Xu Z, Hung MS, et al. Promoter demethylation of WIF-1 by epigallocatechin-3-gallate in lung cancer cells. Anticancer Res 2009;29:2025-30.
- Kerekes K, Bányai L, Trexler M, et al. Structure, function and disease relevance of Wnt inhibitory factor 1, a secreted protein controlling the Wnt and hedgehog pathways. Growth Factors 2019;37:29-52. [Crossref] [PubMed]
- Namiki K, Wongsirisin P, Yokoyama S, et al. (-)-Epigallocatechin gallate inhibits stemness and tumourigenicity stimulated by AXL receptor tyrosine kinase in human lung cancer cells. Sci Rep 2020;10:2444. [Crossref] [PubMed]
- Zhou H, Chen JX, Yang CS, et al. Gene regulation mediated by microRNAs in response to green tea polyphenol EGCG in mouse lung cancer. BMC Genomics 2014;15:S3. [Crossref] [PubMed]
- Wang H, Bian S, Yang CS. Green tea polyphenol EGCG suppresses lung cancer cell growth through upregulating miR-210 expression caused by stabilizing HIF-1α. Carcinogenesis 2011;32:1881-9. [Crossref] [PubMed]
- Zhong Z, Dong Z, Yang L, et al. Inhibition of proliferation of human lung cancer cells by green tea catechins is mediated by upregulation of let-7. Exp Ther Med 2012;4:267-72. [Crossref] [PubMed]
- Zhou DH, Wang X, Feng Q. EGCG enhances the efficacy of cisplatin by downregulating hsa-miR-98-5p in NSCLC A549 cells. Nutr Cancer 2014;66:636-44. [Crossref] [PubMed]
- Jiang P, Wu X, Wang X, et al. NEAT1 upregulates EGCG-induced CTR1 to enhance cisplatin sensitivity in lung cancer cells. Oncotarget 2016;7:43337-51. [Crossref] [PubMed]
- Rizzotto D, Englmaier L, Villunger A. At a Crossroads to Cancer: How p53-Induced Cell Fate Decisions Secure Genome Integrity. Int J Mol Sci 2021;22:10883. [Crossref] [PubMed]
- Gadepalli VS, Deb SP, Deb S, et al. Lung cancer stem cells, p53 mutations and MDM2. Subcell Biochem 2014;85:359-70. [Crossref] [PubMed]
- Yamauchi R, Sasaki K, Yoshida K. Identification of epigallocatechin-3-gallate in green tea polyphenols as a potent inducer of p53-dependent apoptosis in the human lung cancer cell line A549. Toxicol In Vitro 2009;23:834-9. [Crossref] [PubMed]
- Hessien M, El-Gendy S, Donia T, et al. Growth inhibition of human non-small lung cancer cells h460 by green tea and ginger polyphenols. Anticancer Agents Med Chem 2012;12:383-90. [Crossref] [PubMed]
- Jin L, Li C, Xu Y, et al. Epigallocatechin gallate promotes p53 accumulation and activity via the inhibition of MDM2-mediated p53 ubiquitination in human lung cancer cells. Oncol Rep 2013;29:1983-90. [Crossref] [PubMed]
- Ma YC, Li C, Gao F, et al. Epigallocatechin gallate inhibits the growth of human lung cancer by directly targeting the EGFR signaling pathway. Oncol Rep 2014;31:1343-9. [Crossref] [PubMed]
- Li M, Li JJ, Gu QH, et al. EGCG induces lung cancer A549 cell apoptosis by regulating Ku70 acetylation. Oncol Rep 2016;35:2339-47. [Crossref] [PubMed]
- Hada M, Subramanian C, Andrews PC, et al. Cytosolic Ku70 regulates Bax-mediated cell death. Tumour Biol 2016;37:13903-14. [Crossref] [PubMed]
- Shim JH, Su ZY, Chae JI, et al. Epigallocatechin gallate suppresses lung cancer cell growth through Ras-GTPase-activating protein SH3 domain-binding protein 1. Cancer Prev Res (Phila) 2010;3:670-9. [Crossref] [PubMed]
- Li GX, Chen YK, Hou Z, et al. Pro-oxidative activities and dose-response relationship of (-)-epigallocatechin-3-gallate in the inhibition of lung cancer cell growth: a comparative study in vivo and in vitro. Carcinogenesis 2010;31:902-10. [Crossref] [PubMed]
- Deng YT, Lin JK. EGCG inhibits the invasion of highly invasive CL1-5 lung cancer cells through suppressing MMP-2 expression via JNK signaling and induces G2/M arrest. J Agric Food Chem 2011;59:13318-27. [Crossref] [PubMed]
- Sadava D, Whitlock E, Kane SE. The green tea polyphenol, epigallocatechin-3-gallate inhibits telomerase and induces apoptosis in drug-resistant lung cancer cells. Biochem Biophys Res Commun 2007;360:233-7. [Crossref] [PubMed]
- Lamouille S, Xu J, Derynck R. Molecular mechanisms of epithelial-mesenchymal transition. Nat Rev Mol Cell Biol 2014;15:178-96. [Crossref] [PubMed]
- Liu LC, Tsao TC, Hsu SR, et al. EGCG inhibits transforming growth factor-β-mediated epithelial-to-mesenchymal transition via the inhibition of Smad2 and Erk1/2 signaling pathways in nonsmall cell lung cancer cells. J Agric Food Chem 2012;60:9863-73. [Crossref] [PubMed]
- Ko H, So Y, Jeon H, et al. TGF-β1-induced epithelial-mesenchymal transition and acetylation of Smad2 and Smad3 are negatively regulated by EGCG in human A549 lung cancer cells. Cancer Lett 2013;335:205-13. [Crossref] [PubMed]
- Shi J, Liu F, Zhang W, et al. Epigallocatechin-3-gallate inhibits nicotine-induced migration and invasion by the suppression of angiogenesis and epithelial-mesenchymal transition in non-small cell lung cancer cells. Oncol Rep 2015;33:2972-80. [Crossref] [PubMed]
- Takahashi A, Watanabe T, Mondal A, et al. Mechanism-based inhibition of cancer metastasis with (-)-epigallocatechin gallate. Biochem Biophys Res Commun 2014;443:1-6. [Crossref] [PubMed]
- Simonsson M, Kanduri M, Grönroos E, et al. The DNA binding activities of Smad2 and Smad3 are regulated by coactivator-mediated acetylation. J Biol Chem 2006;281:39870-80. [Crossref] [PubMed]
- Stratigos M, Matikas A, Voutsina A, et al. Targeting angiogenesis in small cell lung cancer. Transl Lung Cancer Res 2016;5:389-400. [Crossref] [PubMed]
- Villaruz LC, Socinski MA. The role of anti-angiogenesis in non-small-cell lung cancer: an update. Curr Oncol Rep 2015;17:26. [Crossref] [PubMed]
- Sakamoto Y, Terashita N, Muraguchi T, et al. Effects of epigallocatechin-3-gallate (EGCG) on A549 lung cancer tumor growth and angiogenesis. Biosci Biotechnol Biochem 2013;77:1799-803. [Crossref] [PubMed]
- He L, Zhang E, Shi J, et al. (-)-Epigallocatechin-3-gallate inhibits human papillomavirus (HPV)-16 oncoprotein-induced angiogenesis in non-small cell lung cancer cells by targeting HIF-1α. Cancer Chemother Pharmacol 2013;71:713-25. [Crossref] [PubMed]
- Li X, Feng Y, Liu J, et al. Epigallocatechin-3-gallate inhibits IGF-I-stimulated lung cancer angiogenesis through downregulation of HIF-1α and VEGF expression. J Nutrigenet Nutrigenomics 2013;6:169-78. [Crossref] [PubMed]
- Deng PB, Hu CP, Xiong Z, et al. Treatment with EGCG in NSCLC leads to decreasing interstitial fluid pressure and hypoxia to improve chemotherapy efficacy through rebalance of Ang-1 and Ang-2. Chin J Nat Med 2013;11:245-53. [Crossref] [PubMed]
- Deng P, Hu C, Xiong Z, et al. Epigallocatechin-3-gallate-induced vascular normalization in A549-cell xenograft-bearing nude mice: therapeutic efficacy in combination with chemotherapy. Cancer Manag Res 2019;11:2425-39. [Crossref] [PubMed]
- MacDonagh L, Gallagher MF, Ffrench B, et al. MicroRNA expression profiling and biomarker validation in treatment-naïve and drug resistant non-small cell lung cancer. Transl Lung Cancer Res 2021;10:1773-91. [Crossref] [PubMed]
- Chen A, Jiang P, Zeb F, et al. EGCG regulates CTR1 expression through its pro-oxidative property in non-small-cell lung cancer cells. J Cell Physiol 2020;235:7970-81. [Crossref] [PubMed]
- Flores-Pérez A, Marchat LA, Sánchez LL, et al. Differential proteomic analysis reveals that EGCG inhibits HDGF and activates apoptosis to increase the sensitivity of non-small cells lung cancer to chemotherapy. Proteomics Clin Appl 2016;10:172-82. [Crossref] [PubMed]
- Kim KC, Lee C. Reversal of Cisplatin resistance by epigallocatechin gallate is mediated by downregulation of axl and tyro 3 expression in human lung cancer cells. Korean J Physiol Pharmacol 2014;18:61-6. [Crossref] [PubMed]
- Sang YB, Kim JH, Kim CG, et al. The Development of AXL Inhibitors in Lung Cancer: Recent Progress and Challenges. Front Oncol 2022;12:811247. [Crossref] [PubMed]
- Zhang Y, Wang X, Han L, et al. Green tea polyphenol EGCG reverse cisplatin resistance of A549/DDP cell line through candidate genes demethylation. Biomed Pharmacother 2015;69:285-90. [Crossref] [PubMed]
- Dai L, Wang W, Li W, et al. Effect of Gefitinib Combined with Chemotherapy in Patients with Advanced NSCLC: A Retrospective Cohort Study. Int J Gen Med 2022;15:637-44. [Crossref] [PubMed]
- Liu J, Zhong T, Yi P, et al. A new epigallocatechin gallate derivative isolated from Anhua dark tea sensitizes the chemosensitivity of gefitinib via the suppression of PI3K/mTOR and epithelial-mesenchymal transition. Fitoterapia 2020;143:104590. [Crossref] [PubMed]
- Milligan SA, Burke P, Coleman DT, et al. The green tea polyphenol EGCG potentiates the antiproliferative activity of c-Met and epidermal growth factor receptor inhibitors in non-small cell lung cancer cells. Clin Cancer Res 2009;15:4885-94. [Crossref] [PubMed]
- Zhao H, Zhu W, Xie P, et al. A phase I study of concurrent chemotherapy and thoracic radiotherapy with oral epigallocatechin-3-gallate protection in patients with locally advanced stage III non-small-cell lung cancer. Radiother Oncol 2014;110:132-6. [Crossref] [PubMed]
- Sun XL, Xiang ZM, Xie YR, et al. Dimeric-(-)-epigallocatechin-3-gallate inhibits the proliferation of lung cancer cells by inhibiting the EGFR signaling pathway. Chem Biol Interact 2022;365:110084. [Crossref] [PubMed]
- Wang Y, Shen XJ, Su FW, et al. Novel Perbutyrylated Glucose Derivatives of (-)-Epigallocatechin-3-Gallate Inhibit Cancer Cells Proliferation by Decreasing Phosphorylation of the EGFR: Synthesis, Cytotoxicity, and Molecular Docking. Molecules 2021;26:4361. [Crossref] [PubMed]