Bufalin inhibits epithelial-mesenchymal transition and increases radiosensitivity of non-small cell lung cancer via inhibition of the Src signaling
Highlight box
Key findings
• Bufalin was found to inhibit EMT-related effects via Src-related signaling pathways to enhance radiosensitivity.
What is known and what is new?
• EMT can enhance cell invasion and metastasis and induce tumor cells’ resistance to radiation; Src has been shown to have a critical role in the regulation of the resolution and initiation of EMT programs; bufalin inhibition of STAT3 activity, Src is an upstream activator of STAT3.
• Bufalin enhances the radiosensitivity of NSCLC cells; bufalin inhibits EMT and abrogates radiation-induced EMT; Src mediates bufalin action in cell mobility and EMT.
What is the implication, and what should change now?
• The interaction of Src and STAT3 with bufalin should be addressed by future research; assessment efficacy of bufalin in preclinical and clinical context.
Introduction
Non-small cell lung cancer (NSCLC) accounts for approximately 80% of all lung cancer cases, of which about 25% are diagnosed as locally advanced disease (III stage NSCLC) (1). Radiotherapy is currently considered the best treatment option for advanced lung tumors. Although the tumor can be locally controlled, metastasis after radiation therapy remains the main cause of death (2).
Epithelial-mesenchymal transition (EMT) is a biological process that promotes the malignant behavior of tumor cells after radiation (3-5). The main characteristics of EMT include the transformation of cell morphology from epithelial type to interstitial type, down-regulation of E-cadherin (epithelial marker), and up-regulation of N-cadherin and vimentin (interstitial cell markers). Inhibition of E-cadherin expression has a key role during the process of EMT. The major transcription factors involved in inhibiting E-cadherin expression and inducing EMT include Snail, Slug, and Twist (6). Src signaling have been reported to regulate the resolution and initiation of EMT programs (7). Recent studies have shown that radiation can induce EMT to lead to acquired radiation resistance (3,8,9). However, the underlying mechanisms of these effects remain unknown. Understanding the underlying mechanism of EMT in radiation resistance may help to create more effective anti-NSCLC therapeutic drugs.
Bufalin is an effective anti-cancer agent that has various biological activities. Its structure is similar to digitalis toxin (10-12). Some studies have demonstrated that bufalin exerts significant anti-tumor activity by inhibiting cell proliferation and angiogenesis and inducing programmed cell death (13-15). More recently, studies have discovered that bufalin can inhibit the invasion and metastasis of hepatocellular carcinoma by regulating the PI3K/Akt/Mtor/HIF-1α axis (16,17). The aim of this study was to explore the effect of bufalin on the EMT and radiosensitivity in NSCLC and the underlying molecular mechanism. We found that bufalin inhibits EMT and increases radiosensitivity in NSCLC cells. We also showed that Src signaling is a new major target of bufalin, which reduced p-Src and largely abrogated Src activation triggered by radiation. We present the following article in accordance with the MDAR reporting checklist (available at https://jtd.amegroups.com/article/view/10.21037/jtd-22-1859/rc).
Methods
Reagents and plasmids
Bufalin [high-performance liquid chromatography (HPLC) ≥98%] was obtained from the National Standard Network (Beijing, China) and dissolved in dimethyl sulfoxide (DMSO) to a 10 mM concentration and then stored at −20 ℃. Final concentrations of bufalin were diluted using culture medium (DMSO <3%, 0–200 nM).
Lentiviral short hairpin RNA (shRNA) was generated by inserting shRNA targeting sequence (GACAGACCTGTCCTTCAAGAA) into the pLKO.1 vector.
Antibodies
The antibodies used in this study were purchased from Cell Signaling Technology (CST; Danvers, MA, USA), including anti-Src (#2123), anti-p-Y416-Src (#2102), anti-STAT3 (#12640), anti-p-STAT3 (#9145), anti-E-cadherin (#3195), anti-snail (#3879), anti-snail2 (#9585), anti-vimentin (#5741), anti-N-cadherin (#13116), anti-Vinculin (#4650), anti-GAPDH (#5174), and anti-β-actin (#3700).
Cell culture
We acquired 3 human NSCLC cell lines, H1299, A549, and Hcc827, from the American Type Culture Collection (ATCC; Manassas, VA, USA). The cells were cultured in Dulbecco’s modified Eagle medium (DMEM) or Roswell Park Memorial Institute (RPMI) 1640 medium (Gibco, Grand Island, NY, USA) supplemented with 10% fetal bovine serum (FBS; Gibco) and 1% antibiotics in a humidified atmosphere containing 5% CO2/95% air at 37 ℃.
Irradiation
Cells were incubated with or without bufalin (at a dose of 0–100 nM) for 24 hours. Then, 6 MV X-rays at an exposure rate of 4 Gy/min were used to irradiate the cells at room temperature; the radiation field was 15 cm × 15 cm with the aid of a local irradiation apparatus.
Cell migration and invasion assays
A wound-healing assay was used to measure cell migration. Briefly, NSCLC cells were grown to 90% confluency in the medium containing bufalin (0, 25, 50, or 100 µM). A sterile 1,000 µL pipette tip was then used to scratch 3 separate wounds through the cells. Images of the scratches were obtained using an inverted microscope at ×10 magnification at 0 and 24 hours. Cell migration was quantified and expressed as an average percentage of closure of the scratch area.
The cell invasion assay was evaluated using transwell Matrigel invasion chambers (#3422; Corning Costar Corporation, Cambridge, MA, USA) coated with 100 µL of 1:6 diluted Matrigel. The number of invading cells on the filters was counted in 5 random fields per filter at a 100× magnification in triplicate wells for each group.
Flow cytometry
Flow cytometry was used to analyze the effect of treatment of cell cycle and cell apoptosis. Briefly, cells (4×105/well) in culture medium were seeded in a 6-well plate, then incubated at 37 ℃ for 24 hours. Cells were then washed with cold phosphate-buffered saline (PBS) and resuspended in a binding buffer and then incubated with a fresh medium containing various concentrations of bufalin (0 to 100 nM). Apoptotic cells were detected with ANXA5-FITC/PI Detection Kit (Becton, Dickinson, and Co. BD Biosciences, San Jose, CA, USA) and flow cytometry. Distribution of cell cycle was accessed by flow cytometry according to manufacturer’s instruction.
Clonogenic survival assays
The clonogenic survival assays were performed as previously described (9). For clonogenic survival assay, cells were cultured in a 6-well plate at 1,000 cells per well for 2 weeks. Cells were then stained with crystal violet, and the colony numbers were counted.
Western blot
Cells were lysed in a lysis buffer. The protein concentrations were determined using a bicinchoninic acid (BCA) assay kit (Bio-Rad Laboratories, Inc., Hercules, CA, USA). Equal amounts (20–25 µg) of protein lysate were then resolved on sodium dodecyl sulfate-polyacrylamide gel electrophoresis (SDS-PAGE), transferred to polyvinylidene fluoride (PVDF) membranes (Millipore, Burlington, MA, USA), and immunoblotted as formerly described (15).
Immunofluorescence
Cells were seeded onto coverslips in 6-well plates. Following treatment with radiation or radiation plus bufalin, the cells were fixed with ice-cold methanol for 20 minutes and incubated with 0.1% Triton X-100 in PBS for 10 minutes. After pre-blocking with 2% bovine serum albumin (BSA)/PBS for 45 minutes, the cells were incubated with primary antibodies overnight at 4 ℃ and then with secondary antibodies conjugated with Alexa-Fluor-488 or Alexa-Fluor-594 (Thermo Fisher, Waltham, MA, USA). The nuclei were stained with 4',6-diamidino-2-phenylindole (DAPI).
Statistical analysis
All quantitative data were presented as mean ± standard deviation (SD). Student’s t-test was used to compare the group differences. A P value ≤0.05 was considered statistically significant.
Results
Bufalin decreased cell growth and viability in NSCLC
Previous studies have shown that bufalin can inhibit cell survival and growth of NSCLC (A549 and/or H460 cell lines) (18-20). Another report also demonstrated that bufalin could overcome epidermal growth factor receptor (EGFR)-inhibitor resistance in NSCLC cell lines (21). To further determine whether bufalin inhibits cell proliferation and survival in more NSCLC cell lines, we treated 3 NSCLC cell lines (A549, H1299, and HCC827) with bufalin for 24, 48, and 72 hours, respectively. Cell proliferation was determined with the Cell Counting Kit-8 (CCK-8) and colony formation assays. As shown in Figure 1A, bufalin significantly inhibited the proliferation of all 3 cell lines in a dose- and time-dependent manner. The IC50 value was nearly 30 nM after 24 hours of bufalin treatment. Colony formation assay further revealed that the number of the colony was considerably reduced by bufalin treatment in a dose-dependent fashion (Figure 1B,1C).
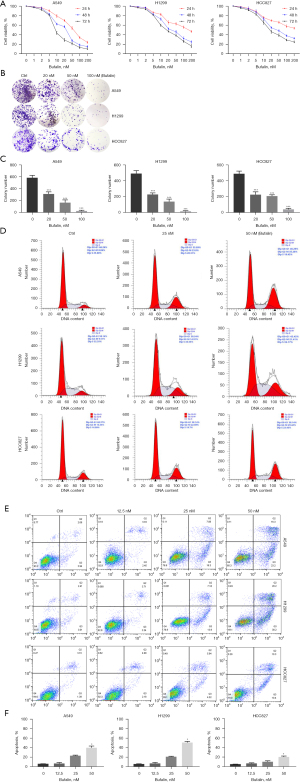
A previous study suggested that bufalin induces cell cycle arrest in malignant cells, but the effect was different among different cell types (22). We next examined the effect of bufalin on the cell cycle in NSCLC cells. Flow cytometry analysis showed that the percentage of cells in the G2-M phase increased in A549, H1299, and Hcc827 cells after treatment with bufalin (50 nM at 48 h) (Figure 1D). Moreover, compared with the control group, the proportion of apoptosis in the 50 nM bufalin treatment group was significantly increased (Figures 1E,1F). These findings suggest that bufalin inhibits NSCLC tumor growth by inducing G2-M cell cycle arrest and promoting programmed cell death.
Bufalin enhances the radiosensitivity of NSCLC cells
Radiotherapy is a critical component in the treatment strategy for NSCLC patients. We next investigated the effect of bufalin on radiosensitivity in NSCLC cells. The A549, H1299, and HCC827 cells were treated with bufalin in combination with and without radiation and radiation alone. After incubation of 24 hours, cell viability was assayed and showed that radiation (2/4/6 Gy) reduced cell survival in a dose-dependent manner. However, the inhibitor effect of a bufalin and radiation was significantly stronger than radiation or bufalin alone (Figure 2A). We also found that the combinational treatment decreased colony formation was more pronounced when compared to radiation alone (Figure 2B). These data revealed that bufalin and radiotherapy have a synergistic effect in NSCLC.
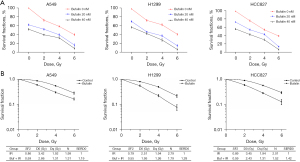
Bufalin inhibits EMT and abrogates radiation-induced EMT
A previous study has suggested that EMT can enhance cell invasion and metastasis and induce tumor cells' resistance to radiation, chemotherapy, and immunotherapy (23). Our results showed that bufalin treatment led to a decrease in N-cadherin, vimentin, Snail, and Slug protein levels and an increase in E-cadherin expression in a dose-dependent manner when compared to untreated cells (Figure 3A and Figure S1A).
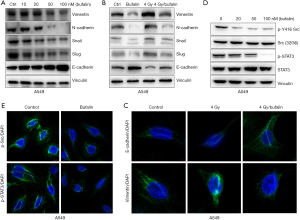
Next, we determined whether bufalin overrides radiation-induced EMT in A549 and H1299 cell lines. As expected, radiation decreased E-cadherin and upregulated N-cadherin, vimentin, Snail, and Slug. However, additional bufalin treatment completely abrogated the changes in these proteins induced by radiation (Figure 3B and Figure S1B). Immunofluorescence analysis further showed that bufalin overrode radiation effects on E-cadherin and vimentin (Figure 3C and Figure S1C). These findings suggested that bufalin enhancing radiosensitivity could be due to inhibition of EMT induced by radiation in NSCLC.
Bufalin inhibits the Src signaling
Src has been shown to have a critical role in the regulation of the resolution and initiation of EMT programs as well as cell migration and invasion (7,24). In addition, previous studies demonstrated bufalin inhibition of STAT3 activity (25,26). As Src is an upstream activator of STAT3 (27), we next investigated whether bufalin inhibits Src activity in 3 NSCLC cell lines examined. Western blot analysis revealed that bufalin considerably reduced the phosphorylation of Src-Y416, an autophosphorylation tyrosine residue present in the activation loop, which in turn reflected Src activation (Figure 3D and Figure S2A,S2B). Accordingly, phosphorylation of STAT3 was inhibited by bufalin. Immunofluorescent staining NSCLC cells with antibodies against p-Src and p-STAT3 also showed the suppression of p-Src and p-STAT3 by bufalin (Figure 3E and Figure S2C,S2D).
Src mediates bufalin action in cell mobility and EMT
Our data revealed that Src was significantly inactivated by bufalin. In order to evaluate whether Src is a major factor in bufalin repression of cell mobility and EMT in NSCLC, Src was knocked down in A549 and H1299 cells; 2 pLKO.1-Src-shRNAs (shSrc-A and shSrc-B) lentiviruses were used. Depletion of Src significantly reduced levels of p-src416 and p-STAT3 in A549 cells (Figure 4A). As shown in Figure 4B, compared with the control group, depletion of Src significantly affected the effect of bufalin on EMT-related proteins in A549 cells. Notably, compared with the shSrc groups, bufalin treatment failed to repress expression of N-cadherin and vimentin, and weakly increased E-cadherin level and repressed the expression of Slug and Snail. This indicates that bufalin almost completely inhibits vimentin and N-cadherin through Src, but only partially affects Snail, Slug, and E-cadherin (Figure 4B). Moreover, depletion of Src largely abrogated bufalin-inhibited cell migration and invasion (Figures S3,S4).
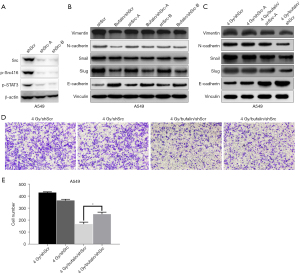
We also examined whether knockdown of Src affects the radiosensitivity enhanced by bufalin. Although depletion of Src had little effect on the occurrence of EMT by simple irradiation, it obviously abrogated the effect of inhibiting the expression of vimentin and N-cadherin when bufalin is combined with radiotherapy, thereby significantly reducing the radiosensitization effect of bufalin (Figures 4C-4E).
To sum up, these findings indicate that bufalin inhibition of cell mobility and EMT, and enhancement of radiosensitivity are largely mediated by targeting Src and its signaling pathways.
Discussion
Radiotherapy is commonly used to treat inoperable NSCLC. Nevertheless, radiation resistance remains a major obstacle for successful treatment (28,29). Multiple factors contribute to radiation resistance, including radiation-induced EMT.
As a broad-spectrum anti-tumor agent, cinobufosin can inhibit cell proliferation and angiogenesis and induce cell apoptosis (16,30,31). Bufalin, a single extract of cinobufagin, can act as a potential radiosensitizer. In this research, we explored the inhibitory effect of bufalin on the migration and invasion of NSCLC cells and its effect on radiotherapy sensitivity.
The aberrant expression of Src is responsible for the disintegration of tight junctions and adhesion junctions and connected with the decrease of epithelial markers and the increase of mesenchymal markers in tumors (32-34). Consistently, our data revealed that an alteration of EMT marker expression induced by radiation could be partially or completely reversed by bufalin in NSCLC cells, and bufalin notably reduces the expressions of Src and downstream proteins, which indicates that bufalin inhibits the initiation of EMT by regulating the activities of Src and STAT3, thus achieving the radiosensitization effect.
Our results also manifest that knockdown of Src reverses the expressions of N-cadherin and vimentin, but does not completely reverse the levels of all EMT markers. Hence, we speculate that inhibition of EMT is only one aspect of bufalin against tumor migration. Several studies have shown that H1299 cells have mesenchymal characteristics (35,36) and that A549 cells have more interstitial properties than epithelial features (37). Moreover, compared with complete EMT, partial EMT is more related to the invasive progression of cancer cells (38). In view of these discoveries, it might be of crucial importance to investigate whether bufalin thoroughly interrupts EMT to generate an epithelial phenotype or it merely inhibits EMT to produce a mixed E/M phenotype in part. The interaction between Src and STAT3 in the pharmacological process of bufalin has not yet been clarified. The STAT3 signaling activation relies on the phosphorylation at tyrosine 705 (Y705) triggered by a number of upstream tyrosine-specific kinases, including receptor tyrosine kinases (RTKs) and cytoplasmic tyrosine kinases (CTKs) containing Src (39,40). Based on previous studies, we elucidated that the inhibitory effect of bufalin on STAT3 activation can be enhanced by the inhibition of bufalin on Src. However, the interaction of Src and STAT3 with bufalin should be addressed by future research.
A recent study has pointed out that the root bark of Morus alba affects cancer cell migration and EMT markers by inhibiting STAT3 (41). Flavonoids compounds luteolin and quercetin can repress the migration and invasion of squamous cell carcinoma by reducing the Src/STAT3/S100A7 pathway (42). Although some natural products have been shown to be relatively effective inhibitors of EMT both in vivo and in vitro, meticulous studies are still warranted to verify their effects in clinical trials. These natural products have huge therapeutic potential in traditional chemotherapy and combined radiotherapy and chemotherapy.
Conclusions
To sum up, our findings provide novel insights into the mechanism of bufalin inhibiting NSCLC cell migration and invasion. We demonstrated that EMT mediated by Src and STAT3 has a vital role in the pharmacological activity of bufalin. Further investigations are warranted to assess the efficacy of bufalin in preclinical and clinical context and we prudently indicate that bufalin may be employed as a radiosensitizer in clinical practice.
Acknowledgments
Funding: This work was partially supported by the Natural Science Foundation of Shandong Province (No. ZR2019MH058).
Footnote
Reporting Checklist: The authors have completed the MDAR reporting checklist. Available at https://jtd.amegroups.com/article/view/10.21037/jtd-22-1859/rc
Data Sharing Statement: Available at https://jtd.amegroups.com/article/view/10.21037/jtd-22-1859/dss
Conflicts of Interest: All authors have completed the ICMJE uniform disclosure form (available at https://jtd.amegroups.com/article/view/10.21037/jtd-22-1859/coif). The authors have no conflicts of interest to declare.
Ethical Statement: The authors are accountable for all aspects of the work in ensuring that questions related to the accuracy or integrity of any part of the work are appropriately investigated and resolved.
Open Access Statement: This is an Open Access article distributed in accordance with the Creative Commons Attribution-NonCommercial-NoDerivs 4.0 International License (CC BY-NC-ND 4.0), which permits the non-commercial replication and distribution of the article with the strict proviso that no changes or edits are made and the original work is properly cited (including links to both the formal publication through the relevant DOI and the license). See: https://creativecommons.org/licenses/by-nc-nd/4.0/.
References
- Palomar-Abril V, Soria-Comes T, Campos ST, et al. Dynamic evaluation of neutrophil-to-lymphocyte ratio as prognostic factor in stage III non-small cell lung cancer treated with chemoradiotherapy. Clin Transl Oncol 2020;22:2333-40. [Crossref] [PubMed]
- Prise KM, Schettino G, Folkard M, et al. New insights on cell death from radiation exposure. Lancet Oncol 2005;6:520-8. [Crossref] [PubMed]
- Zhou YC, Liu JY, Li J, et al. Ionizing radiation promotes migration and invasion of cancer cells through transforming growth factor-beta-mediated epithelial-mesenchymal transition. Int J Radiat Oncol Biol Phys 2011;81:1530-7. [Crossref] [PubMed]
- El Bezawy R, Cominetti D, Fenderico N, et al. miR-875-5p counteracts epithelial-to-mesenchymal transition and enhances radiation response in prostate cancer through repression of the EGFR-ZEB1 axis. Cancer Lett 2017;395:53-62. [Crossref] [PubMed]
- Andarawewa KL, Erickson AC, Chou WS, et al. Ionizing radiation predisposes nonmalignant human mammary epithelial cells to undergo transforming growth factor beta induced epithelial to mesenchymal transition. Cancer Res 2007;67:8662-70. [Crossref] [PubMed]
- Strong MS, Vaughan CW, Kayne HL, et al. A randomized trial of preoperative radiotherapy in cancer of the oropharynx and hypopharynx. Am J Surg 1978;136:494-500. [Crossref] [PubMed]
- Kamran MZ, Patil P, Gude RP. Role of STAT3 in cancer metastasis and translational advances. Biomed Res Int 2013;2013:421821. [Crossref] [PubMed]
- Jung JW, Hwang SY, Hwang JS, et al. Ionising radiation induces changes associated with epithelial-mesenchymal transdifferentiation and increased cell motility of A549 lung epithelial cells. Eur J Cancer 2007;43:1214-24. [Crossref] [PubMed]
- Wang H, Wang Z, Li Y, et al. Silencing Snail Reverses Epithelial-Mesenchymal Transition and Increases Radiosensitivity in Hypopharyngeal Carcinoma. Onco Targets Ther 2020;13:497-511. [Crossref] [PubMed]
- Calderón-Montaño JM, Burgos-Morón E, Orta ML, et al. Evaluating the cancer therapeutic potential of cardiac glycosides. Biomed Res Int 2014;2014:794930. [Crossref] [PubMed]
- Datta P, Dasgupta A. Interactions between drugs and Asian medicine: displacement of digitoxin from protein binding site by bufalin, the constituent of Chinese medicines Chan Su and Lu-Shen-Wan. Ther Drug Monit 2000;22:155-9. [Crossref] [PubMed]
- Dasgupta A, Emerson L. Neutralization of cardiac toxins oleandrin, oleandrigenin, bufalin, and cinobufotalin by digibind: monitoring the effect by measuring free digitoxin concentrations. Life Sci 1998;63:781-8. [Crossref] [PubMed]
- Zhang Z, Fan Y, Xie F, et al. Breast cancer metastasis suppressor OTUD1 deubiquitinates SMAD7. Nat Commun 2017;8:2116. [Crossref] [PubMed]
- Qi F, Li A, Inagaki Y, et al. Antitumor activity of extracts and compounds from the skin of the toad Bufo bufo gargarizans Cantor. Int Immunopharmacol 2011;11:342-9. [Crossref] [PubMed]
- Zhang L, Pan YM, Wang LY, et al. Therapeutic Targets of Bufalin on Renal Carcinoma and Mechanisms: Experimental Validation of Network Pharmacology Analysis. Evid Based Complement Alternat Med 2022;2022:5469795. [Crossref] [PubMed]
- Wang H, Zhang C, Xu L, et al. Bufalin suppresses hepatocellular carcinoma invasion and metastasis by targeting HIF-1α via the PI3K/AKT/mTOR pathway. Oncotarget 2016;7:20193-208. [Crossref] [PubMed]
- Sheng X, Zhu P, Zhao Y, et al. Effect of PI3K/AKT/mTOR Signaling Pathway on Regulating and Controlling the Anti-Invasion and Metastasis of Hepatoma Cells by Bufalin. Recent Pat Anticancer Drug Discov 2021;16:54-65. [Crossref] [PubMed]
- Kim NY, Suh YA, Kim S, et al. Bufalin down-regulates Axl expression to inhibit cell proliferation and induce apoptosis in non-small-cell lung cancer cells. Biosci Rep 2020;40:BSR20193959. [Crossref] [PubMed]
- Jiang Y, Zhang Y, Luan J, et al. Effects of bufalin on the proliferation of human lung cancer cells and its molecular mechanisms of action. Cytotechnology 2010;62:573-83. [Crossref] [PubMed]
- Liu M, Feng LX, Sun P, et al. A Novel Bufalin Derivative Exhibited Stronger Apoptosis-Inducing Effect than Bufalin in A549 Lung Cancer Cells and Lower Acute Toxicity in Mice. PLoS One 2016;11:e0159789. [Crossref] [PubMed]
- Kang XH, Xu ZY, Gong YB, et al. Bufalin Reverses HGF-Induced Resistance to EGFR-TKIs in EGFR Mutant Lung Cancer Cells via Blockage of Met/PI3k/Akt Pathway and Induction of Apoptosis. Evid Based Complement Alternat Med 2013;2013:243859. [Crossref] [PubMed]
- Gu W, Liu L, Fang FF, et al. Reversal effect of bufalin on multidrug resistance in human hepatocellular carcinoma BEL-7402/5-FU cells. Oncol Rep 2014;31:216-22. [Crossref] [PubMed]
- Yang SS, Yu DY, Du YT, et al. Inhibition of Delta-like Ligand 4 enhances the radiosensitivity and inhibits migration in cervical cancer via the reversion of epithelial-mesenchymal transition. Cancer Cell Int 2020;20:344. [Crossref] [PubMed]
- Zhang S, Yu D. Targeting Src family kinases in anti-cancer therapies: turning promise into triumph. Trends Pharmacol Sci 2012;33:122-8. [Crossref] [PubMed]
- Yang L, Zhou F, Zhuang Y, et al. Acetyl-bufalin shows potent efficacy against non-small-cell lung cancer by targeting the CDK9/STAT3 signalling pathway. Br J Cancer 2021;124:645-57. [Crossref] [PubMed]
- Fang K, Zhan Y, Zhu R, et al. Bufalin suppresses tumour microenvironment-mediated angiogenesis by inhibiting the STAT3 signalling pathway. J Transl Med 2021;19:383. [Crossref] [PubMed]
- Roskoski R Jr. Src protein-tyrosine kinase structure, mechanism, and small molecule inhibitors. Pharmacol Res 2015;94:9-25. [Crossref] [PubMed]
- Fukusumi T, Ishii H, Konno M, et al. CD10 as a novel marker of therapeutic resistance and cancer stem cells in head and neck squamous cell carcinoma. Br J Cancer 2014;111:506-14. [Crossref] [PubMed]
- Wang S, Song M, Zhang B. Trichostatin A enhances radiosensitivity and radiation-induced DNA damage of esophageal cancer cells. J Gastrointest Oncol 2021;12:1985-95. [Crossref] [PubMed]
- Zhao L, Liu S, Che X, et al. Bufalin inhibits TGF-β-induced epithelial-to-mesenchymal transition and migration in human lung cancer A549 cells by downregulating TGF-β receptors. Int J Mol Med 2015;36:645-52. [Crossref] [PubMed]
- Gai JQ, Sheng X, Qin JM, et al. The effect and mechanism of bufalin on regulating hepatocellular carcinoma cell invasion and metastasis via Wnt/β-catenin signaling pathway. Int J Oncol 2016;48:338-48. [Crossref] [PubMed]
- Patel A, Sabbineni H, Clarke A, et al. Novel roles of Src in cancer cell epithelial-to-mesenchymal transition, vascular permeability, microinvasion and metastasis. Life Sci 2016;157:52-61. [Crossref] [PubMed]
- Liu X, Feng R. Inhibition of epithelial to mesenchymal transition in metastatic breast carcinoma cells by c-Src suppression. Acta Biochim Biophys Sin (Shanghai) 2010;42:496-501. [Crossref] [PubMed]
- Yuan Z, Liu C, Sun Y, et al. Bufalin exacerbates Photodynamic therapy of colorectal cancer by targeting SRC-3/HIF-1α pathway. Int J Pharm 2022;624:122018. [Crossref] [PubMed]
- Biswas K, Jolly MK, Ghosh A. Stability and mean residence times for hybrid epithelial/mesenchymal phenotype. Phys Biol 2019;16:025003. [Crossref] [PubMed]
- Jolly MK, Tripathi SC, Jia D, et al. Stability of the hybrid epithelial/mesenchymal phenotype. Oncotarget 2016;7:27067-84. [Crossref] [PubMed]
- Roche J, Gemmill RM, Drabkin HA. Epigenetic Regulation of the Epithelial to Mesenchymal Transition in Lung Cancer. Cancers (Basel) 2017;9:72. [Crossref] [PubMed]
- Nieto MA, Huang RY, Jackson RA, et al. EMT: 2016. Cell 2016;166:21-45. [Crossref] [PubMed]
- Levy DE, Darnell JE Jr. Stats: transcriptional control and biological impact. Nat Rev Mol Cell Biol 2002;3:651-62. [Crossref] [PubMed]
- Schindler C, Levy DE, Decker T. JAK-STAT signaling: from interferons to cytokines. J Biol Chem 2007;282:20059-63. [Crossref] [PubMed]
- Min TR, Park HJ, Park MN, et al. The Root Bark of Morus alba L. Suppressed the Migration of Human Non-Small-Cell Lung Cancer Cells through Inhibition of Epithelial-Mesenchymal Transition Mediated by STAT3 and Src. Int J Mol Sci 2019;20:2244. [Crossref] [PubMed]
- Fan JJ, Hsu WH, Lee KH, et al. Dietary Flavonoids Luteolin and Quercetin Inhibit Migration and Invasion of Squamous Carcinoma through Reduction of Src/Stat3/S100A7 Signaling. Antioxidants (Basel) 2019;8:557. [Crossref] [PubMed]
(English Language Editor: J. Jones)