Lipopolysaccharide and lipoteichoic acid regulate the PI3K/AKT pathway through osteopontin/integrin β3 to promote malignant progression of non-small cell lung cancer
Highlight box
Key findings
• We demonstrated that lipoteichoic acid (LTA) and lipopolysaccharide (LPS) increased osteopontin/integrin β3 expression and activated the phosphatidylinositol 3-kinase (PI3K)/AKT pathway to promote malignant progression of non-small cell lung cancer (NSCLC) through chronic inflammation mechanisms.
What is known and what is new?
• Previous studies found that the lung microbiota may be different in NSCLC patients with different clinical and pathological characteristics, and this may play an important role in the development of NSCLC.
• This current research investigated the molecular mechanisms of LTA and LPS in promoting the malignant progression of lung cancer in terms of inflammation.
What is the implication, and what should change now?
• Our research provided a theoretical basis for further exploring the influence of lung microbiota on the progress of NSCLC and the optimization of LC therapy in the future.
Introduction
Lung cancer (LC) is one of the most fatal cancers in the world, and non-small cell lung cancer (NSCLC) is the main type of LC. Despite recent progress in the treatment of NSCLC, the overall survival rate remains low. While our understanding of the genome is expanding, there remains a paucity of data regarding the external factors controlling NSCLC progression. The lung is a mucosal tissue with a large surface area, that is continuously exposed to certain airborne bacteria. Therefore, the relationship between lung flora and the development of LC has attracted increasing attention. In addition to the composition of the lung flora, there are many other factors that may affect the microenvironment of lung tumors, such as structural changes after LC surgery, local atelectasis caused by tumor tissue blocking the trachea and bronchus, tumor rupture and bleeding in the airway, bronchiectasis caused by tumor pulling the bronchus, low immunity caused by chemotherapy and disease development itself, bone marrow suppression, radiation induced airway mucosal damage, the decline of ciliary clearance ability, and the use of antibiotics. The lung resident immune system plays an important part in maintaining stable balance of lung tissue and monitoring the invasion of pathogens. Chronic inflammation is an important factor in the development of LC. In our previous study, we screened 37 patients with stage III or IV NSCLC who were treated in our hospital from May 2017 to April 2019, and collected tumor tissue, as well as plasma and bronchoalveolar lavage fluid (BALF) samples for pathogen targeting sequencing or 16S rDNA sequencing. We confirmed that there were 49 different types of gram-negative bacteria and gram-positive bacteria in LC tumor tissue, including Haemophilus parainfluenzae, Enterococcus, Staphylococcus haemolyticus, Peptostreptococcus, and defective anatrophic bacteria (1). Lipopolysaccharide (LPS) and lipoteichoic acid (LTA) are the main cell lysis components of gram-negative and gram-positive bacteria respectively. Studies suggested that LPS and LTA can stimulate cancer cells to release proinflammatory factors, induce local inflammation, and promote cancer progression (2). Osteopontin (OPN) interacts with integrin, CD44 and other receptors to regulate inflammation, immunity, bone metabolism, tumor and other physiological and pathological processes, and participates in multiple cancer metastasis (3). This study discussed that some specific bacterial components or products of respiratory tract microbiota can induce osteopontin expression through inflammatory mechanism and promote the malignant progression of lung cancer cells. We present the following article in accordance with the MDAR and ARRIVE reporting checklists (available at https://jtd.amegroups.com/article/view/10.21037/jtd-22-1825/rc).
Methods
Cell grouping
Prepare cell culture medium contains 0, 0.01, 0.1, and 1 µL/mL LPS (from Escherichia coli, Sigma Aldrich, USA), and containing 0, 5, 10, and 30 µL/mL LTA (from Staphylococcus aureus, Sigma Aldrich, USA) respectively. When the cells grow to 60–70% confluence, the prepared culture medium was used to stimulate the cells for 24 hours. The expression of inflammatory factors was analyzed by ELISA, and the PC9 and H1299 cell lines were selected to act on by LTA and LPS at appropriate doses for subsequent experiments.
Determination of LTA and LPS concentration by enzyme-linked immunosorbent assay (ELISA)
Add standard solution and diluent of standard solution respectively into 10 holes on the enzyme label coating plate, as a result of 50 µL samples each hole, then mix well. The concentration of standard LTA was 300, 200, 100, 50 and 25 ng/mL, and the concentration of LPS standard was 360, 240, 120, 60, and 30 ng/mL, respectively. Add sample diluent and sample to be tested into the sample hole to be tested. Blank hole were set. After sealing the plate with the sealing film, place it at 37 °C and incubate for 30 minutes. Remove the sealing film, discard the liquid, shake it dry, add detergent, and let it stand for 30 seconds. Repeat for 5 times, and pat it dry. Add 50 µL enzyme labeled reagent except for blank holes. Warm cultivate and wash. Add 50 µL developer A first. Then add 50 µL developer B. Color rendering in dark at 37 °C for 15 minutes. Add 50 µL stop liquid to stop reaction. Measure the absorbance (OD value) of each hole in sequence at 450 nm wavelength.
Cell viability determination
The Cell Counting Kit 8 (CCK-8) was used to measure the number of cells to observe the effect of LPA or LTA on cell proliferation. The Transwell assay was performed to measure the migration ability of cells. Apoptosis was assessed using flow cytometry. Each experiment was repeated 3 times.
qRT-PCR
Total RNA was extracted from the cells using Trizol reagent (TaKaRa (Dalian) Co., Ltd). The EasyScript First Strand cDNA Synthesis Super Mix kit was used for cDNA synthesis. The expression level of each gene was detected using SYBRgreen. Specific primers for each gene were synthesized by Sangon Biotech (Shanghai) Co., Ltd. (Table 1). The PCR reaction conditions were as follows: 94 °C pre denaturation, 240 s; 65 °C, 30 s; 72 °C, 60 s; 30 cycles; 72 °C, 300 s. Fluorescence of each PCR extension phase was collected. The cycle threshold (Ct) value was analyzed using SDS 2.2 software. Glyceraldehyde 3-phosphate dehydrogenase (GAPDH) was selected as the internal reference. The 2-∆∆Ct method was used for the analysis of the relative expression of target genes. Apoptosis was observed by flow cytometry. Each experiment was repeated 3 times. All data were used for statistical analysis.
Table 1
Primer name | Sequence |
---|---|
IL-1β | F (5'-3'): TTTGAGTCTGCCCAGTTCCC |
R (5'-3'): GTTATATCCTGGCCGCCTT | |
IL-6 | F (5'-3'): CACAGACAGCCACTCACCT |
R (5'-3'): GCCTCTTTGCTGCTTTCACAC | |
TNF-α | F (5'-3'): GCTCTTCTGCCTGCTGCACTT |
R (5'-3'): ACATGGGCTACAGGCTTGTCAC | |
CXCL-1 | F (5'-3'): CCAAAGTGTGAACGTGAAGTCCC |
R (5'-3'): CTTTCCGCCCATTCTTGAGTGT | |
CXCL-6 | F (5'-3'): TGGTAAACTGCAGGTGTTCCCC |
R (5'-3'): CCAGACAAACTTGCTTCCCGTTC | |
SPP1 | F (5'-3'): TTTCACTCCAGTTGTCCCC |
R (5'-3'): GTGATGTCCTCGTCTGTAGC | |
TLR2 | F (5'-3'): CTGGAGAACTTCAATCCCC |
R (5'-3'): TTTGTGGCTCTTTTCA | |
TLR4 | F (5'-3'): GTATTTATTGCACAGACTTGCG |
R (5'-3'): AGCCTAATTTCTTTAAATGCAC | |
Caspase-3 | F (5'-3'): ATGACATCTCGGTCTGGTA |
R (5'-3'): CTTTAGAAACATCACGCATC | |
Caspase-9 | F (5'-3'): GTGAACTTCTGCCGTGAGTCC |
R (5'-3'): AGCACCATTTTCTTGGCAGTCA | |
PI3K | F (5'-3'): TTATAAACGAGAACGTGTG |
R (5'-3'): AATAGCTAGATAAGCC | |
AKT | F (5'-3'): CAGCATCGCTTCTTTGCCGGTA |
R (5'-3'): CCTGGTGTCAGTCTCCGACGTGA | |
ERK | F (5'-3'): ACAGGGCTCCAGAAATTATGTTG |
R (5'-3'): AGAATGCAGCCTACAGACCAA |
IL, interleukin; TNF, tumor necrosis factor; CXCL, C-X-C motif ligand; SPP1, secreted phosphoprotein 1; TLR, toll-like receptor; PI3K, Phosphatidylinositol 3-kinase; AKT, rac-alpha serine/threonine-protein kinase; ERK, extracellular signal-regulated kinase.
Western blot
Cells were lysed with RIPA buffer and the total protein concentration was quantified using the BCA protein quantitative kit. Protein samples (50 µg) were separated via 10% sodium dodecyl sulfate/polyacrylamide (SDS-PAGE) electrophoresis, transferred to polyvinylidene fluoride (PVDF) membranes, and sealed at room temperature for 2 hours with 5% degreased protein. Membranes were then incubated with antibodies against SUZ12 (1:1,000 dilution) and GAPDH (1:10,000 dilution) at 4 °C overnight. After 3 washes with 0.1% PBS Tween (PBST), membranes were incubated with a horseradish peroxidase labeled secondary antibody (1:10,000 dilution) at room temperature for 1 hour. Following another 3 washes with PBST, the ECL-PLUS chemiluminescence kit was used for detection of protein bands. Each experiment was repeated 3 times.
Tumor transplantation in mice
To assess the role of pathogenic bacteria in the progression of LC, a tumor transplantation test was performed in mice. All animal experiments were carried out in Kangtai Medical Laboratory Services Hebei Co. Ltd. (SYXK (Ji) 2021-006). BALB/C nude mice (female, 5 weeks old) were purchased from Shanghai Institute of Biological Sciences, Chinese Academy of Sciences. A total of 18 nude mice, weighing 50 g each, were divided into the following three groups (n=6): 1 group was injected with cells that had been transfected with a negative control small interfering RNA (siNC); 1 group was injected with cells that had been transfected with integrin β3 siRNA; and the control group did not undergo any operation. After 4 weeks, the mice were sacrificed and the weight and volume of the transplanted tumor was measured. The expression of the PI3K/AKT/ERK protein was detected by Western blot analysis. Animal experiments were performed under a project license (No. MDL2021-09-20-01) granted by Laboratory Animal Ethics Committee of Kangtai Medical Laboratory Services Hebei Co. Ltd., in compliance with institutional guidelines for the care and use of animals. A protocol was prepared before the study without registration.
The overall experimental design is shown in Figure 1.
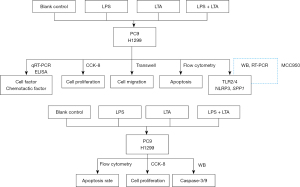
Statistical analyses
The SPSS23.0 software was used for all statistical analyses and Graphpad Prism7.0 was used to generate graphs. The measurement data was expressed as mean ± standard deviation (mean ± SD) and the counting data was expressed as a percentage. The measurement data from two independent samples were compared using T test. The counting data were compared using Chi square test. Analysis of variance was used for comparison between groups. The tests were repeated three times. A P value <0.05 was considered statistically significant.
Results
The effects of LPS and LTA on the expression of inflammatory factors and inflammatory chemokines in NSCLC cells
Different concentrations of LPS and LTA were used to treat PC9 cells and H1299 cells. Three inflammatory factors were analyzed. Enzyme linked immunosorbent assay (ELISA) showed that the expression of inflammatory factors in both cell lines was significantly increased after treatment with 1 µg/mL LPS and 30 µg/mL LTA (Figure 2). Combined with previous study (4), these concentrations of LPS and LTA were chosen as the optimum concentration for subsequent tests.
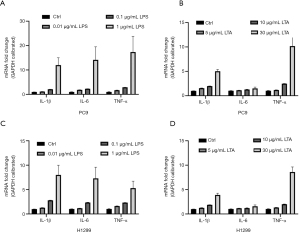
The results of qRT-PCR analysis showed that in the PC9 cell line, the expression of both CXCL1 and CXCL6 were significantly higher after treatment with LPS, LTA, and LPS + LTA compared to the control group, and the expression of CXCL1 and CXCL6 in the LPS + LTA group was significantly higher than that in either the LPS or LTA group alone (P<0.001; Figure 3A-3D). ELISA demonstrated that LPS and LTA promoted the expression of TNF-α, IL-1β, and IL-6, and the expression of these inflammatory factors was significantly higher in the LPS + LTA group compared to the single treatment groups (P<0.001). Furthermore, the proinflammatory effects of LPS was greater than that of LTA (P<0.001; Figure 3E-3J). A similar trend was observed in H1299 cells.
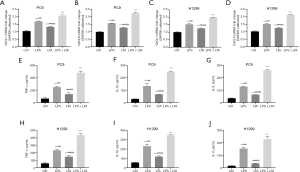
The effects of LPS and LTA on proliferation, apoptosis, and migration of NSCLC cells
The CCK-8 assay demonstrated that LPS and LTA caused a time-dependent inhibition of proliferation in PC9 and H1299 cells (Figure 4A,4B). After 96 hours, LPS combined with LTA had the greatest inhibitory effect on the proliferation of lung cancer cells. LPS + LTA also significantly increased cell migration and decreased apoptosis in PC9 cells compared with either treatment alone, as shown by Transwell assays and flow cytometry, respectively (P<0.001; Figure 4C,4D). H1299 cells showed a similar trend (Figure 4E,4F). These results suggested that LPS and LTA may play a crucial role in promoting NSCLC progression.
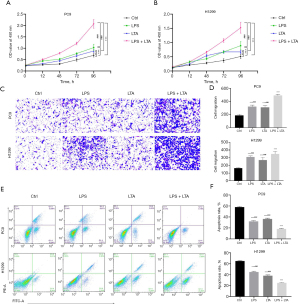
The effects of LPS and LTA on inflammatory mechanisms in NSCLC cells
LPS and LTA activate NOD like receptor thermal protein domain associated protein 3 (NLRP3) in a TLR2/4 dependent manner to promote the expression of SPP1. A study has shown that gram-negative bacteria containing LPS can activate TLR4, while gram-positive bacteria containing LTA can activate TLR2 (5). Western blot was applied to analyze the expression of TLR2/4, NLRP3, and SPP1 protein levels in PC9 and H1299 cells. The results showed that LPS significantly activated TLR4 expression (P<0.001) and LTA significantly activated TLR2 expression (P<0.001) in PC9 lung cancer cells. All cells treated with LPS and/or LTA significantly upregulated the protein expression of NLRP3 and SPPI in lung cancer cells, and the elevated expression was more significant in the combined treatment group compared to LPS or LTA alone (Figure 5A-5Q). Treatment of cells with the NLRP3 inhibitor MCC950 significantly reduced the mRNA expression of NLRP3 in the LPS + LTA group (P<0.001), as well as the expression of SPP1 (P<0.001; Figure 5R,5S). The above results suggested that LPS and LTA activate TLR4 and TLR2, respectively, which in turn upregulates the expression of SPP1 by promoting the expression of NLRP3 in inflammatory bodies.
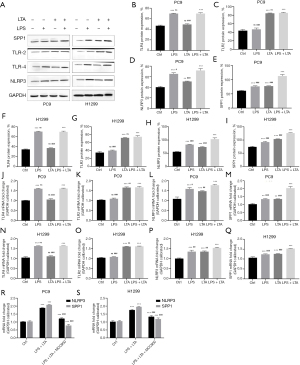
The effects of LPS and LTA on cisplatin sensitivity in lung cancer cells
Cisplatin is a first-line anti-cancer drug. We analyzed the influence of LPS and LTA on the therapeutic effect of cisplatin on lung cancer cells. CCK-8 analysis showed that combined treatment with LPS + LTA + cisplatin significantly reduced the inhibitory effects of LPS on cell proliferation (P<0.001; Figure 6A,6B). Flow cytometry revealed that the LPS + LTA + cisplatin group showed substantially reduced apoptosis rate compared to cells treated with cisplatin alone (P<0.001; Figure 6C-6E). These results suggested that LPS combined with LTA could significantly reduce cisplatin sensitivity, promote cell proliferation, and reduce apoptosis.
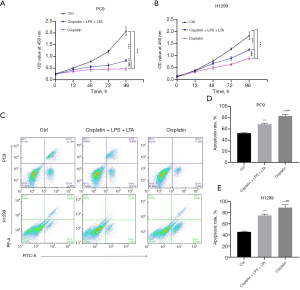
The effects of LPS and LTA on caspase-3 and caspase-9
Since caspase-3 and caspase-9 participate in cisplatin-induced apoptosis (6), the effect of LPS and LTA on the expression of these apoptotic proteins was assessed by Western blot. As shown in Figure 7A-7C, in PC9 cell lines, both the cisplatin and LPS + LTA + cisplatin groups showed elevated caspase-3 and caspase-9 protein expression compared with the control group (P<0.001). However, the LPS + LTA + cisplatin group showed significantly reduced levels of caspase-3 and caspase-9 compared to cisplatin alone (P<0.001). A similar trend was observed with the H1299 cells (Figure 7D,7E). This data demonstrated that cisplatin induced upregulation of caspase-3 and caspase-9, while lung microbiota (rich in LPS and LTA) decreased caspase-3 and -9 expression, inhibited cell apoptosis, and reduced cisplatin sensitivity.
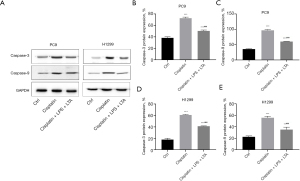
LPS and LTA regulated the expression of integrin β3 through OPN
To determine the mechanisms of LPS and LTA, the expression of OPN was silenced in PC9 and H1299 cells. Cells were then transfected with siNC or SPP1 siRNA, and treated with LPS + LTA or blank control. The expression of SPP1 and integrin β3 was analyzed by qRT-PCR. LPS + LTA treatment in the siNC group significantly increased SPP1 and integrin β3 expression (P<0.001; Figure 8). However, cells in which SPP1 was silenced (SPP1 siRNA) showed dramatically reduced SPP1 and integrin β3 expression even after LPS + LTA treatment (P<0.001). These results suggested that LPS and LTA regulated the expression of integrin β3 through upregulation of SPP1 expression.
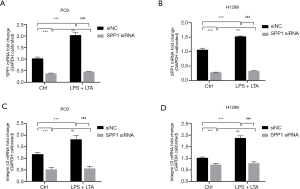
Regulation of integrin β3 by LPS and LTA affects the bioactivity of NSCLC cells
The study found that LPS and LTA, the main components of lung microorganisms, can regulate the expression of integrin β3 by activating OPN protein. The biological activity of lung cancer cells was analyzed after silencing integrinβ3. CCK-8 analysis showed that the proliferation activity of siNC cells treated with LPS + LTA was the highest, followed by integrin β3 siRNA cells treated with LPS + LTA (Figure 9A,9B). Silencing the expression of integrin β3 in lung cancer cells reduced the proliferative effects of the microbial toxins. Flow cytometry analysis showed that the apoptosis rate of LPS + LTA-treated siNC transfected cells was lower than that of integrin β3 siRNA cells treated with LPS + LTA (P<0.001; Figure 9C-9E), and indeed, the apoptosis rate of LSP + LTA treated lung cancer cells increased after knocking out integrin β3. LPS + LTA treatment increased the migration distance of lung cancer cells (P<0.001) in the PC9 cell line, while silencing integrin β3 significantly reduced lung cancer cell migration distance (P<0.01) compared with the siNC group. A similar trend as observed in H1299 cells (Figure 9F-9H).
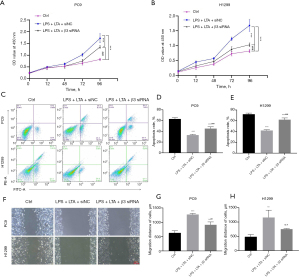
The above results demonstrated that silencing integrin β3 can reverse the influence of LPS + LTA on the bioactivity of lung cancer cells. LPS + LTA promotes the proliferation and migration of lung cancer cells and inhibits apoptosis, and this mechanism is related to integrin β3.
LPS + LTA can activate integrin β3 and regulate the PI3K/AKT/ERK pathway
PC9 and H1299 lung cancer cells were transfected with siNC or integrin β3 siRNA and treated with LPS + LTA. qRT-PCR results showed that LPS + LTA promoted the mRNA expression levels of PI3K, AKT, and ERK in lung cancer cells, which were significantly decreased after silencing integrin β3 (P<0.01; Figure 10A-10F). Western blot analysis demonstrated that phosphorylated (p)-PI3K, p-AKT, and p-ERK protein expression were significantly increased in lung cancer cells after LPS + LTA treatment compared with the control group (P<0.001), and significantly decreased after silencing integrin β3 (P<0.001). There were no significant changes in PI3K, AKT, nor ERK protein expression regardless of silencing integrin β3 (P>0.05; Figure 10G-10S). The results in the H1299 cell line were consistent with those in the PC9 cell line. These data suggested that LPS + LTA may activate the phosphorylation levels of the PI3K/AKT/ERK pathway in lung cancer cells, and promote the expression of the PI3K/AKT/ERK pathway via integrin β3.
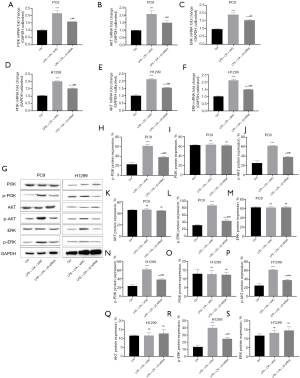
LPS + LTA regulates the PI3K/AKT/ERK pathway by activating integrin β3 in tumorigenesis in nude mice
The PC9 lung cancer cell line transfected with siNC or integrin β3 siRNA were injected into the back of the nude mice for 4 weeks. After the nude mice were sacrificed, the weight and volume of the transplanted tumor were assessed. Tumors from cells treated with LPS + LTA were significantly bigger than tumors from control cells (P<0.001). However, the weight and volume of transplanted tumors from cells transfected with integrin β3 siRNA were significantly smaller than that of the LPS + LTA mice (P<0.001; Figure 11A,11B). Western blot results showed that p-PI3K, p-AKT, and p-ERK protein expression levels were significantly increased in the xenografts in the LPS+LTA-treated group (P<0.01, P<0.05, and P<0.001, respectively), and their expressions were significantly inhibited after silencing integrin β3 (Figure 11C-11I).
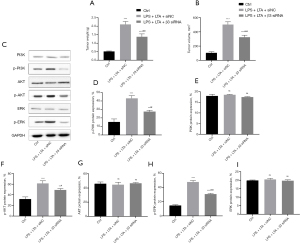
In summary, the animal transplantation tumor experiment confirmed that LPS and LTA could activate the phosphorylation levels of PI3K/AKT/ERK in lung cancer cells via integrin β3, thereby promoting the expression of the PI3K/AKT/ERK pathway.
Discussion
In the process of cancer occurrence, the microecology in the lung microenvironment is out of balance, gradually forming a microenvironment that promotes cancer progress, thus inducing a series of chain reactions, and finally causing cancer. The imbalance of lung microecology may affect the carcinogenesis of lung cancer through inflammation, immunity, metabolism and genotoxicity. Chronic inflammation is a key risk factor for lung cancer It can form proinflammatory cytokines, adhesion molecules and growth factors to provide a favorable microenvironment for tumor cell survival and proliferation. Some microorganisms are important pathogens that induce inflammation in cancer (7). Nejman et al. (8) and Poore et al. (9) proposed that there are different intratumoral microbiological compositions in more than 30 cancers. These latter studies provided imaging evidence of the intratumoral spatial distribution and intracellular localization of these microorganisms in seven types of cancers, performed typical histological tests for 6 cancers, and demonstrated using immunohistochemistry of that LPS and LTA are the main components of cell lysis. It was further proposed that LPS and LTA can stimulate cancer cells to release proinflammatory factors, inducing local inflammation and promoting cancer progression (2). However, there is a paucity of data regarding the mechanisms of the malignant bioactivity of LPS and LTA in lung cancer cells. To the best of our knowledge, this current study is the first to explore the molecular mechanism of LPS and LTA in stimulating the malignant progression of lung cancer cells.
Of the LPS components, only the lipid A group has endotoxin activity. However, the induction of LTA on inflammatory reaction is relatively weak, but in the presence of peptidoglycan and other bacterial components, the inflammatory reaction will be moderately amplified (10). Arabzadeh et al. reported that the ovarian cancer cell line was stimulated by 10 µg/mL LPS and 30 µg/mL LTA, and TLR-2, TLR-4, and IL-6 receptors were activated, thus starting NF-κB and STAT3 to up regulate Wnt5A-ROR2 to promote tumor cell growth and migration, revealing the role of inflammatory mediators in the growth and migration of ovarian cancer cells (4). In lung cancer, the role of LTA in promoting the proliferation of cancer cells is not limited to A549 cells, but also significantly promotes the NSCLC cell line originated from squamous cells (11). Moreover, some studies have shown that the proliferation promotion effect of pathogenic bacteria on cancer cells can be solely due to LTA, which inhibits the activation of LPS induced TLR4 cells, and the effect of LTA on the proliferation of NSCLC cell lines can still be seen (12). Our study found that compared with LPS and LTA treatment alone, combined LPS and LTA treatment can significantly promote the release of inflammatory factors from lung cancer cells. LPS and LTA activate TLR4 and TLR2, respectively, and then promote the malignant biological activity of cancer cells by enhancing the expression of NLRP3, promoting the release of cancer cell chemokines and inflammatory factors, and upregulating the expression of SPP1. The combination of LPS and LTA can significantly promote the proliferation and migration of lung cancer cells, inhibit the apoptosis of cancer cells, and play a cancer-promoting role. This current study examined the mechanisms by which LPS and LTA promote the malignant progression of lung cancer cells. Lipid A with different structures can produce different kinds of cytokines in the body, causing different immune responses, and thus the results have some limitations, In the future, we will further distinguish the different structures of lipid A of different strains, conduct in-depth research and comparison, and further examine the impact of different strains on LC.
SPP1 can bind to the extracellular matrix through transglutaminase and mediate the adhesion process of tumor cells. It was first found in malignant epithelial cells (13). The study found that SPP1 is a cancer promoting gene, which facilitates the malignant progression of LC (14). SPP1 is also a driving factor for epithelial to mesenchymal transition (EMT) (15). However, the effects of LC microorganisms on the expression of SPP1 have not been previously reported. This study found that LPS and LTA induced the upregulation of TLR4/2 expression in lung cancer cell lines PC9 and H1299, activated NLRP3, and further enhanced SPP1 expression. The upregulation of SPP1 expression can significantly reduce the cisplatin sensitivity of PC9 and H1299 cell lines, which is consistent with previous research conclusions. LPS combined with LTA treatment of lung cancer cells can promote cell proliferation and reduce the rate of apoptosis while reducing the sensitivity of cisplatin. Cisplatin can induce the upregulated expression of apoptotic proteins caspase-3 and caspase-9 in lung cancer cells, while lung microbiota (rich in LPS and LTA) can inhibit the expression of caspase-3 and caspase-9, inhibit cell apoptosis, and reduce the sensitivity of cisplatin. All these data provide new references for tumor treatment.
Researches have confirmed that progression of LC is related to the interaction between OPN and integrin β3. OPN (SPP1) is a glycosylated phosphoprotein with the ability to bind calcium and integrin. It can be alternatively spliced to produce a variety of local functions, such as tumor growth, invasion, metastasis, angiogenesis, drug resistance, and immunosuppression. It is widely expressed in bone, kidney, breast, thymus, the immune system, and the brain (16). The most versatile subtype in tumor tissue is β3 (17), which is related to growth, invasion, metastasis, and survival. It is also one of the indicators of increased lymph node or bone metastasis and decreased survival rate of patients in some cancers (18). Integrin β3 affects tumor cell initiation, survival, and stemness by regulating cytokines, thereby promoting carcinogenesis and tumor growth (19). In this study, we preliminarily analyzed the interaction between OPN and integrin β3 in the malignant bioactivity of lung cancer cells, which was similar to previous literature. We found that LPS combined with LTA can significantly activate SPP1, and then promoted the expression of integrin β3 to further exert their effects. Silencing the expression of integrin β3 reversed the influence of LPS and LTA on the bioactivity of lung cancer cells. Therefore, LPS + LTA plays a role in promoting the proliferation and migration of lung cancer cells, and inhibiting apoptosis of lung cancer cells.
Phosphatidylinositol 3-kinase (PI3K) consists of a catalytic subunit of 110 KD and a subunit of 85 KD. Many studies have shown that PI3K is widespread in most tissues and has carcinogenic potential (20). Research showed that SPP1 maintains tumor cell angiogenesis by activating the PI3K/AKT pathway (21). Integrin adhesion also activates RAS-MAPK and PI3K-AKT signaling pathways, thereby activating GTPase RAP1A on the plasma membrane, which has an influence on promoting cell migration and invasion (22). Fortin et al. found that the PI3K/AKT signaling pathway was activated in LC. In contrast, AKT phosphorylation of multiple targets can control cell proliferation (23). The above results are similar to those of our study. Integrin β3 plays a role in promoting the progress of LC by activating the PI3K/AKT pathway. Furthermore, the in vitro experiment showed that the lung microbiota can induce integrin β3 activation by promoting the expression of SPP1. Integrin β3 can activate the phosphorylation levels of PI3K/AKT/ERK, thereby promoting the expression of PI3K/AKT/ERK pathway. These results were confirmed by in vivo transplantation experiments in mice. The results demonstrated that the lung microbiota can activate the phosphorylation level of PI3K/AKT/ERK, which in turn promotes the expression of the PI3K/AKT/ERK pathway via integrin β3.
Conclusions
In this study, we analyzed the distribution characteristics of non-infectious NSCLC lung pathogens obtained from a variety of specimens in clinical practice. We examined the main virulence factors LPS and LTA. The experimental data demonstrated that OPN/integrin β3 regulates the PI3K/AKT pathway to promote the malignant progression of LC. These results lay the preliminary experimental foundations for further detailed research on the impact of lung microbiota on the NSCLC microenvironment and the optimization of LC treatment.
Acknowledgments
Funding: None.
Footnote
Reporting Checklist: The authors have completed the MDAR and ARRIVE reporting checklists. Available at https://jtd.amegroups.com/article/view/10.21037/jtd-22-1825/rc
Data Sharing Statement: Available at https://jtd.amegroups.com/article/view/10.21037/jtd-22-1825/dss
Conflicts of Interest: All authors have completed the ICMJE uniform disclosure form (available at https://jtd.amegroups.com/article/view/10.21037/jtd-22-1825/coif). ZG is from Beijing Goldstandard Medicine Independent Clinical Laboratory Co. Ltd. The other authors have no conflicts of interest to declare.
Ethical Statement: The authors are accountable for all aspects of the work in ensuring that questions related to the accuracy or integrity of any part of the work are appropriately investigated and resolved. Animal experiments were performed under a project license (No. MDL2021-09-20-01) granted by Laboratory Animal Ethics Committee of Kangtai Medical Laboratory Services Hebei Co. Ltd., in compliance with institutional guidelines for the care and use of animals.
Open Access Statement: This is an Open Access article distributed in accordance with the Creative Commons Attribution-NonCommercial-NoDerivs 4.0 International License (CC BY-NC-ND 4.0), which permits the non-commercial replication and distribution of the article with the strict proviso that no changes or edits are made and the original work is properly cited (including links to both the formal publication through the relevant DOI and the license). See: https://creativecommons.org/licenses/by-nc-nd/4.0/.
References
- Zhang M, Zhang Y, Han Y, et al. Lung microbiota features of stage III and IV non-small cell lung cancer patients without lung infection. Transl Cancer Res 2022;11:426-34. [Crossref] [PubMed]
- Xu T, Deng R, Li X, et al. RNA-seq analysis of different inflammatory reactions induced by lipopolysaccharide and lipoteichoic acid in bovine mammary epithelial cells. Microb Pathog 2019;130:169-77. [Crossref] [PubMed]
- Butti R, Kumar TVS, Nimma R, et al. Osteopontin Signaling in Shaping Tumor Microenvironment Conducive to Malignant Progression. Adv Exp Med Biol 2021;1329:419-41. Erratum in: Adv Exp Med Biol 2021;1329:C1-C2. [Crossref] [PubMed]
- Arabzadeh S, Hossein G, Salehi-Dulabi Z, et al. WNT5A-ROR2 is induced by inflammatory mediators and is involved in the migration of human ovarian cancer cell line SKOV-3. Cell Mol Biol Lett 2016;21:9. [Crossref] [PubMed]
- Kuo YJ, Chung CH, Huang TF. From Discovery of Snake Venom Disintegrins to A Safer Therapeutic Antithrombotic Agent. Toxins (Basel) 2019;11:372. [Crossref] [PubMed]
- Wu Z, Min L, Chen D, et al. Overexpression of BMI-1 promotes cell growth and resistance to cisplatin treatment in osteosarcoma. PLoS One 2011;6:e14648. [Crossref] [PubMed]
- Wang Y, Yang H, Xu H. Age-specific microbiota in altering host inflammatory and metabolic signaling and metabolome based on sex. Hepatobiliary Surg Nutr 2022;11:305-7. [Crossref] [PubMed]
- Nejman D, Livyatan I, Fuks G, et al. The human tumor microbiome is composed of tumor type-specific intracellular bacteria. Science 2020;368:973-80. [Crossref] [PubMed]
- Poore GD, Kopylova E, Zhu Q, et al. Microbiome analyses of blood and tissues suggest cancer diagnostic approach. Nature 2020;579:567-74. [Crossref] [PubMed]
- Hulina A, Grdić Rajković M, Jakšić Despot D, et al. Extracellular Hsp70 induces inflammation and modulates LPS/LTA-stimulated inflammatory response in THP-1 cells. Cell Stress Chaperones 2018;23:373-84. [Crossref] [PubMed]
- Hattar K, Reinert CP, Sibelius U, et al. Lipoteichoic acids from Staphylococcus aureus stimulate proliferation of human non-small-cell lung cancer cells in vitro. Cancer Immunol Immunother 2017;66:799-809. [Crossref] [PubMed]
- Hattar K, Savai R, Subtil FS, et al. Endotoxin induces proliferation of NSCLC in vitro and in vivo: role of COX-2 and EGFR activation. Cancer Immunol Immunother 2013;62:309-20. [Crossref] [PubMed]
- Fong YC, Liu SC, Huang CY, et al. Osteopontin increases lung cancer cells migration via activation of the alphavbeta3 integrin/FAK/Akt and NF-kappaB-dependent pathway. Lung Cancer 2009;64:263-70. [Crossref] [PubMed]
- Tang H, Chen J, Han X, et al. Upregulation of SPP1 Is a Marker for Poor Lung Cancer Prognosis and Contributes to Cancer Progression and Cisplatin Resistance. Front Cell Dev Biol 2021;9:646390. [Crossref] [PubMed]
- Kaufhold S, Garbán H, Bonavida B. Yin Yang 1 is associated with cancer stem cell transcription factors (SOX2, OCT4, BMI1) and clinical implication. J Exp Clin Cancer Res 2016;35:84. [Crossref] [PubMed]
- Ali MN, Mori M, Mertens TCJ, et al. Osteopontin Expression in Small Airway Epithelium in Copd is Dependent on Differentiation and Confined to Subsets of Cells. Sci Rep 2019;9:15566. [Crossref] [PubMed]
- Cobb DA, de Rossi J, Liu L, et al. Targeting of the alphav beta3 integrin complex by CAR-T cells leads to rapid regression of diffuse intrinsic pontine glioma and glioblastoma. J Immunother Cancer 2022;10:e003816. [Crossref] [PubMed]
- Surapaneni SK, Patel N, Sun L, et al. Anticancer and chemosensitization effects of cannabidiol in 2D and 3D cultures of TNBC: involvement of GADD45α, integrin-α5, -β5, -β1, and autophagy. Drug Deliv Transl Res 2022;12:2762-77. [Crossref] [PubMed]
- Huynh TT, Sreekumar S, Mpoy C, et al. Therapeutic Efficacy of 177Lu-Labeled A20FMDV2 Peptides Targeting ανβ6. Pharmaceuticals (Basel) 2022;15:229. [Crossref] [PubMed]
- Sun F, Mu C, Kwok HF, et al. Capivasertib restricts SARS-CoV-2 cellular entry: a potential clinical application for COVID-19. Int J Biol Sci 2021;17:2348-55. [Crossref] [PubMed]
- Ji X, Liu Y, Mei F, et al. SPP1 overexpression is associated with poor outcomes in ALK fusion lung cancer patients without receiving targeted therapy. Sci Rep 2021;11:14031. [Crossref] [PubMed]
- Hamidi H, Ivaska J. Every step of the way: integrins in cancer progression and metastasis. Nat Rev Cancer 2018;18:533-48. [Crossref] [PubMed]
- Fortin J, Mak TW. Targeting PI3K Signaling in Cancer: A Cautionary Tale of Two AKTs. Cancer Cell 2016;29:429-31. [Crossref] [PubMed]
(English Language Editor: J. Teoh)