Prediction of postoperative pulmonary complications in older patients undergoing lobectomy for lung cancer based on skeletal muscle mass
Highlight box
Key findings
• Independent risk factors for postoperative pulmonary complications (PPCs) after lobectomy for lung cancer were age, smoking history, diabetes mellitus, functional vital capacity (FVC) and skeletal muscle mass.
What is known and what is new?
• Preoperative pulmonary function tests (PFTs) are commonly used for the evaluation of preoperative PPC risk after lung cancer surgery.
• Previous studies have demonstrated that low skeletal muscle mass is associated with morbidity after major surgery.
• The present study shows that older patients with PPCs after lobectomy for lung cancer had significantly lower FVC and forced expiratory volume in 1 second (FEV1) values than those without PPCs. They also had a significantly lower skeletal muscle mass, represented by the erector spinae muscle.
What is the implication, and what should change now?
• Preoperative PFT and measurement of skeletal muscle mass are useful tools to predict PPCs in patients undergoing lobectomy for lung cancer.
Introduction
Despite continuous developments in the diagnosis and treatment of lung cancer, postoperative pulmonary complications (PPCs) are the major causes of morbidity and mortality following lung cancer surgery. There has been a great deal of effort to develop ways to prevent PPCs after lung cancer surgery (1,2). Preoperative risk stratification can help to predict the possibility of PPCs after lung cancer surgery and allow the implementation of strategies to prevent them. Preoperative pulmonary function tests (PFTs) are commonly used for the evaluation of preoperative PPC risk after lung cancer surgery.
Previous studies have demonstrated that low skeletal muscle mass is associated with morbidity after major surgery (3-5). Moreover, low skeletal muscle mass has been shown decreased lung function in patients with lung diseases (6,7). Low skeletal muscle mass, referred to as sarcopenia, is one of the most important causes of functional decline with a loss of independence in the elderly. Therefore, low skeletal muscle mass in older patients is expected to be associated with low pulmonary function and PPCs after lung cancer surgery. However, the relevant study has not been yet conducted.
We hypothesised that patients with PPCs after lung cancer surgery may have low preoperative PFT results and a low skeletal muscle mass compared to patients without PPCs after lung cancer surgery. This retrospective study was designed to evaluate preoperative PFT and skeletal muscle mass, represented by the erector spinae muscle (EM), as predictors of PPCs in older patients undergoing lobectomy for lung cancer. We present the following article in accordance with the STARD reporting checklist (available at https://jtd.amegroups.com/article/view/10.21037/jtd-22-1156/rc).
Methods
Study population
The study was conducted in accordance with the Declaration of Helsinki (as revised in 2013). This retrospective study was performed using the medical records of patients older than 65 years old undergoing lobectomy for lung cancer at Konkuk University Medical Center from January 2016 to December 2021 after receiving approval from the Institutional Review Board of Konkuk University Medical Center (approval No. KUMC 202207071) and registration at https://cris.nih.go.kr (registration number: KCT0007474). The patient data were obtained from chart reviews, and the requirement for written informed consent from the patients was waived. All data, extracted from chart reviews, were deidentified prior to analysis. The exclusion criteria were previous thoracic surgery, metastasis from other organs, concurrent other surgery, previous respiratory disease except lung cancer, history of heart failure, left or right ventricular ejection fraction below the normal range, sequelae of cerebrovascular disease and low renal function with elevated creatinine above the normal range (Figure 1).
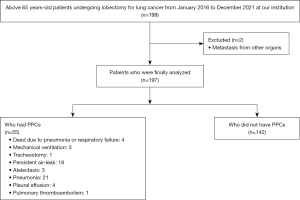
Preoperative PFTs
PFTs were performed within 1 week before lobectomy for lung cancer. Functional vital capacity (FVC), forced expiratory volume in 1 second (FEV1), forced expiratory flow (FEF), forced expiratory flow between 25% and 75% (FEF25–75%) and maximal voluntary ventilation (MVV) were measured according to institutional protocols.
Preoperative chest computed tomography (CT)
Chest CT was performed within 1 week before surgery for lung cancer. Images were obtained in steps of 2.5 mm according to our institutional protocol.
For skeletal muscle mass, the cross-sectional areas (CSAs) of the right and left EMs were measured. The contours of both EMs were identified at the level of the spinous process with the 12th thoracic vertebra. CSAs of the right and the left EMs were checked and the sum (CSABoth) was used as the skeletal muscle mass (Figure 2). All procedures were performed by a radiologist who was blinded to the study, using software, Centricity PACS Radiology RA1000 Workstation (GE Healthcare Inc., Chicago, IL, USA).
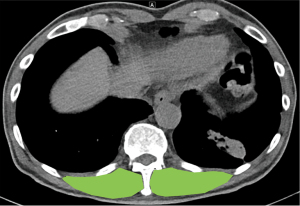
Perioperative care
Perioperative management was standardised and followed the institutional protocol. Lobectomy for lung cancer was performed using the video-assisted or open technique under general anaesthesia after discussion with the thoracic surgeon and attending anaesthesiologist. One-lung ventilation was secured using a double-lumen endotracheal tube or endobronchial blocker. Video-assisted thoracoscopic surgery (VATS) was performed through a 4-cm utility incision at anterior or middle axillary line of the fifth intercostal space (ICS) by using Alexis wound protector (Applied Medical, USA), a 12-mm trocar port at the eighth ICS and a 5-mm port at the subscapular area. Thoracotomy was performed through a posterolateral incision that spared the serratus anterior muscle. The chest cavity was entered through the fifth ICS with segmental resection of the sixth rib. A chest tube was inserted to check the drainage and air leakage after lobectomy under general anaesthesia. The chest tube was connected to an underwater seal chest drainage system. Postoperative pain was controlled with an intravenous patient-controlled analgesia pump. After emergence from general anaesthesia for lobectomy, the patient was transferred into the intensive care unit (ICU). Postoperative care was performed by the thoracic surgeon following our institutional protocol, including the decision of transfer from the ICU to the general ward. The chest tube was removed after confirmation that there was no drainage and no air leakage.
PPCs
The following PPCs within 30 days after lobectomy for lung cancer were evaluated: mortality, need for mechanical ventilation, tracheostomy, persistent air leakage, atelectasis, pneumonia, pleural effusion, haemothorax, chylothorax, pyothorax, pulmonary thromboembolism and nerve injury (e.g., phrenic nerve and recurrent laryngeal nerve).
PPCs were defined as above grade III in The Clavien-Dindo Classification (8).
Postoperative chest radiography was performed daily until discharge from the hospital. Any new-onset abnormal findings detected on postoperative chest radiography or pulmonary symptoms or signs that were not observed before lobectomy for lung cancer were checked, evaluated and managed by the thoracic surgeon using additional evaluation tools, including chest CT, if needed.
Continuous negative suction was applied to the bottle for the chest tube in patients with persistent air leakage. Pleurodesis was performed at the discretion of the thoracic surgeon in cases in which continuous negative suction was not effective.
Statistical analysis
Demographic data, including preoperative PFT, CSAs of the right and left EMs and CSABoth, were analysed according to the presence of PPCs.
Statistical analyses were conducted using SPSS for Windows (ver. 27.0; IBM Corp., Armonk, NY, USA). Categorical variables were analysed using the chi-square or Fisher’s exact test, and continuous variables were analysed using the independent t-test.
The correlation between preoperative PFT and CSABoth was confirmed using Pearson’s correlation coefficient. A univariate analysis was performed for each potential risk factor of PPCs using logistic regression. Factors with P<0.05 in univariate analysis were included in a multivariate conditional logistic regression model using the backward stepwise regression procedure. Odds ratios (ORs) with the 95% confidence interval (CI) were calculated based on the final model. To obtain cut-off values for preoperative PFT and CSABoth for the prediction of PPCs, the area under the curve (AUC) with receiver operating characteristic (ROC) curve analysis was determined with Youden’s index.
Data are expressed as the number of patients, mean [standard deviation (SD)]. In all analyses, P<0.05 was taken to indicate statistical significance.
Results
During the study period, 199 patients older than 65 years old underwent lobectomy for lung cancer at our centre. Two patients were excluded due to metastasis from other organs. Therefore, data from a total of 197 patients were included in the analyses (Figure 1). In total, 55 patients had PPCs: 4 died due to pneumonia or respiratory failure within 30 days after lobectomy, 3 required mechanical ventilation, 1 required tracheostomy, 18 had persistent air leakage, 3 had atelectasis, 21 had pneumonia, 4 had pleural effusion and 1 patient had pulmonary thromboembolism (Figure 1).
Preoperative PFT and chest CT were performed within 3 [3–4] and 3 [3–4] days, respectively.
The patients with PPCs were significantly older than those without PPCs (Table 1). The patients with PPCs significantly showed the higher rates of smoking history, including ex-smokers and active smokers (Table 1). The patients with PPCs had significantly higher rates of underlying diseases, such as hypertension (HTN) and diabetes mellitus (DM) (Table 1). The rates of medication with angiotensin-converting enzyme inhibitor (ACEi) or angiotensin receptor blocker (ARB) and hypoglycaemic agents were also significantly higher in patients with PPCs (Table 1). The patients with PPCs significantly showed the lower rate of pathological stage I and the higher rate of pathological stage II (Table 1).
Table 1
Variables | With PPCs (n=55) | Without PPCs (n=142) | P value |
---|---|---|---|
Sex (M/F) | 32/23 | 64/78 | 0.099 |
Age (years) | 75 (6.0) | 73 (5.0) | 0.004 |
Height (cm) | 158 (0.09) | 158 (0.07) | 0.666 |
Weight (kg) | 63.8 (10.8) | 64.3 (8.6) | 0.749 |
Smoking history | |||
Non-smoker | 16 | 94 | <0.001 |
Ex-smoker | 25 | 43 | 0.013 |
Active smoker | 14 | 5 | <0.001 |
Underlying disease | |||
HTN | 28 | 47 | 0.021 |
DM | 21 | 29 | 0.010 |
Cardiovascular disease | 7 | 10 | 0.066 |
CVA history | 1 | 6 | 0.413 |
Current medications | |||
ACEi or ARB | 17 | 21 | 0.010 |
β-blocker | 8 | 11 | 0.147 |
CCB | 6 | 11 | 0.478 |
Diuretic | 4 | 6 | 0.382 |
Hypoglycaemic agent | 19 | 21 | 0.002 |
Antiplatelet agents | 12 | 20 | 0.187 |
Location of lesion | |||
Right upper lobe | 21 | 43 | 0.720 |
Right middle lobe | 4 | 12 | 0.786 |
Right lower lobe | 6 | 29 | 0.117 |
Left upper lobe | 11 | 35 | 0.752 |
Left lower lobe | 13 | 23 | 0.225 |
VATS/thoracotomy | 47/8 | 117/25 | 0.606 |
Operation time (min) | 241 (53.0) | 234 (64.0) | 0.662 |
Type of lung cancer | |||
Adenocarcinoma | 37 | 111 | 0.112 |
Squamous cell carcinoma | 2 | 4 | 0.764 |
Large cell carcinoma | 16 | 26 | 0.097 |
Small cell carcinoma | 0 | 1 | 0.533 |
Pathological stage | |||
I | 35 | 122 | <0.001 |
II | 15 | 14 | 0.003 |
III | 5 | 6 | 0.059 |
Data are expressed as number of patients or mean (SD). M, male; F, female; HTN, hypertension; DM, diabetes mellitus; CVA history, history of cerebrovascular accident; ACEi, angiotensin-converting enzyme inhibitor; ARB, angiotensin receptor blocker; CCB, calcium channel blocker; VATS, video-assisted thoracoscopic surgery; PPCs, postoperative pulmonary complications; SD, standard deviation.
Patients with PPCs had significantly lower preoperative FVC [2.53 (0.45) vs. 3.00 (0.58) L, respectively; P=0.001] and FEV1 [1.79 (0.40) vs. 2.13 (0.42) L, respectively; P=0.001] values than those without PPCs (Table 2). However, there were no significant differences in FEV1/FVC, FEF, FEF25–75% or MVV between patients with and without PPCs (Table 2).
Table 2
Variables | With PPCs | Without PPCs | P value |
---|---|---|---|
Preoperative PFT | |||
FVC (L) | 2.53 (0.45) | 3.00 (0.58) | 0.001 |
FEV1 (L) | 1.79 (0.40) | 2.13 (0.42) | 0.001 |
FEV1/FVC | 70.77 (7.91) | 71.52 (8.10) | 0.554 |
FEF (L/s) | 6.90 (2.47) | 6.94 (1.92) | 0.900 |
FEF25–75% (L/s) | 1.38 (0.78) | 1.46 (0.65) | 0.521 |
MVV (L/min) | 94.21 (13.28) | 94.50 (11.43) | 0.369 |
CSABoth (mm2) | 26.91 (4.64) | 30.85 (5.88) | 0.001 |
Data are expressed as mean (SD). PFT, pulmonary function test; FVC, functional vital capacity; FEV1, forced expiratory volume in 1 second; FEF, forced expiratory flow; FEF25–75%, forced expiratory flow between 25% and 75%; MVV, maximal voluntary ventilation; PPCs, postoperative pulmonary complications.
The CSABoth was significantly lower in patients with than without PPCs [26.91 (4.64) vs. 30.85 (5.88) mm2, respectively; P=0.001] (Table 2).
The preoperative FVC and FEV1 were significantly positively correlated with CSABoth (r=0.526, P<0.001; r=0.404, P<0.001) (Figure 3).
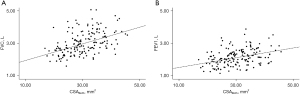
A univariate analysis identified age, smoking history, HTN, DM, ACEi or ARB, hypoglycaemic agents, pathological stage, FVC, FEV1 and CSABoth as risk factors for PPCs. However, multiple logistic regression analysis identified age (OR, 1.102; 95% CI, 1.001–1.213; P=0.048), ex-smokers (OR, 4.966; 95% CI, 1.988–12.406; P=0.001), active smokers (OR, 31.891; 95% CI, 7.152–142.195; P<0.001), DM (OR, 8.142; 95% CI, 2.608–25.417; P<0.001), FVC (OR, 0.295; 95% CI, 0.116–0.747; P=0.010) and CSABoth (OR, 0.878; 95% CI, 0.796–0.967; P=0.009) as independent risk factors for PPCs (Table 3). The AUCs for age, FVC and CSABoth were 0.620 (95% CI, 0.534–0.706; P<0.05), 0.727 (95% CI, 0.650–0.803; P<0.001) and 0.685 (95% CI, 0.608–0.762; P<0.001), respectively (Figure 4) (the AUC for DM was not analysed because DM was a categorical and not numerical variable). The optimal threshold values for age, FVC and CSABoth to predict PPCs based on ROC curve analysis were 73.5 years (sensitivity 58.2% and specificity 59.2%), 2.685 L (sensitivity 64.1% and specificity 61.8%) and 28.47 mm2 (sensitivity 62.0% and specificity 61.5%), respectively (Figure 4).
Table 3
Variables | Univariable | Multivariable | |||
---|---|---|---|---|---|
Odd ratio | P value | Odd ratio | P value | ||
Sex (M/F) | 0.590 (0.314–1.107) | 0.100 | |||
Age (years) | 1.095 (1.027–1.168) | 0.006 | 1.102 (1.001–1.213) | 0.048 | |
Height (cm) | 1.408 (0.018–107.56) | 0.877 | |||
Weight (kg) | 0.994 (0.960–1.028) | 0.712 | |||
Smoking history | |||||
Non-smoker | 0.186 (0.093–0.369) | <0.001 | |||
Ex-smoker | 3.935 (0.960–1.028) | <0.001 | 4.966 (1.988–12.406) | 0.001 | |
Active smoker | 16.293 (5.075–52.314) | <0.001 | 31.891 (7.152–142.195) | <0.001 | |
Underlying disease | |||||
HTN | 1.888 (1.003–3.555) | 0.047 | |||
DM | 2.407 (1.219–4.750) | 0.010 | 8.142 (2.608–25.417) | <0.001 | |
Cardiovascular disease | 0.519 (0.187–1.442) | 0.209 | |||
CVA history | 2.382 (0.280–20.256) | 0.427 | |||
Current medications | |||||
ACEi or ARB | 2.238 (1.069–4.683) | 0.033 | |||
β-blocker | 0.493 (0.187–1.301) | 0.153 | |||
CCB | 0.686 (0.241–1.955) | 0.480 | |||
Diuretic | 0.563 (0.152–2.075) | 0.388 | |||
Hypoglycaemic agent | 3.041 (1.475–6.270) | 0.003 | |||
Antiplatelet agents | 0.587 (0.265–1.302) | 0.190 | |||
Lesion of lung cancer* | |||||
Right upper lobe | Not applicable | – | |||
Right middle lobe | 0.733 (0.210–2.557) | 0.626 | |||
Right lower lobe | 0.455 (0.163–1.270) | 0.133 | |||
Left upper lobe | 0.776 (0.334–1.806) | 0.557 | |||
Left lower lobe | 1.243 (0.525–2.942) | 0.620 | |||
VATS/thoracotomy | 0.797 (0.335–1.892) | 0.606 | |||
Operation time | 1.002 (0.993–1.012) | 0.655 | |||
Type of lung cancer† | |||||
Adenocarcinoma | Not applicable | – | |||
Squamous cell carcinoma | 1.500 (0.264–8.526) | 0.647 | |||
Large cell carcinoma | 1.846 (0.894–3.814) | 0.098 | |||
Small cell carcinoma | 1.103 (0.202–2.944) | 0.843 | |||
Pathological stage | |||||
I | 0.270 (0.130–0.562) | <0.001 | |||
II | 3.735 (1.646–8.476) | 0.002 | |||
III | 2.905 (0.836–10.087) | 0.093 | |||
Preoperative PFT | |||||
FVC (L) | 0.173 (0.083–0.360) | 0.001 | 0.295 (0.116–0.747) | 0.010 | |
FEV1 (L) | 0.087 (0.034–0.226) | 0.001 | |||
FEV1/FVC | 0.964 (0.924–1.005) | 0.091 | |||
FEF (L/s) | 0.990 (0.852–1.151) | 0.900 | |||
FEF25–75% (L/s) | 0.845 (0.529–1.350) | 0.482 | |||
MVV (L/min) | 1.017 (0.986–1.038) | 0.367 | |||
CSABoth (mm2) | 0.876 (0.822–0.933) | 0.001 | 0.878 (0.796–0.967) | 0.009 |
Data are expressed as 95% CI. *, in the present study, most common lesion of lung cancer was right upper lobe. Odd ratio was obtained from right upper lobe as baseline. †, in the present study, most common type of lung cancer was adenocarcinoma. Odd ratio was obtained from adenocarcinoma as baseline. M, male; F, female; HTN, hypertension; DM, diabetes mellitus; CVA history, history of cerebrovascular accident; ACEi, angiotensin-converting enzyme inhibitor; ARB, angiotensin receptor blocker; CCB, calcium channel blocker; VATS, video-assisted thoracoscopic surgery; PFT, pulmonary function test; FEV1, forced expiratory volume in 1 second; FVC, functional vital capacity; FEF, forced expiratory flow; FEF25–75%, forced expiratory flow between 25% and 75%; MVV, maximal voluntary ventilation; CSABoth, sum of the cross-sectional areas of the right and left erector spinae muscles; CI, confidential interval.
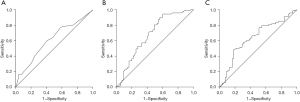
Discussion
The present study shows that older patients with PPCs after lobectomy for lung cancer had significantly lower FVC and FEV1 values than those without PPCs. They also had a significantly lower skeletal muscle mass, represented by the EM. Preoperative FVC and FEV1 showed significant positive correlations with CSABoth. Independent risk factors for PPCs after lobectomy for lung cancer were age, smoking history, DM, FVC and CSABoth.
The patients who developed PPCs after lung cancer surgery had poorer long-term outcomes with a reduction in overall survival (1). With regard to the surgical technique for lung cancer surgery, VATS has been shown to be associated with a lower incidence of PPCs in comparison with thoracotomy (9). However, PPCs remain a major issue that must be resolved for the improvement of both survival and quality of life. Old age, obesity, smoking, chronic obstructive pulmonary disease (COPD) and poor pulmonary function measured with the PFT were reported to be risk factors for PPCs after lung cancer surgery (10). However, the predictive capabilities of these factors were poor. Moreover, poor pulmonary function, as determined by the PFT, was not associated with low morbidity or mortality in patients undergoing VATS for lung cancer surgery (11,12). Berry et al. (13) concluded that preoperative PFT was associated with PPCs after lung cancer surgery only in thoracotomy, but not in VATS. As most recent cases of lung cancer surgery have been performed using VATS and not thoracotomy, a new objective means of predicting PPCs after lung cancer surgery via both thoracotomy and VATS is required. Most cases in the present study were treated by VATS, but preoperative FVC, one of the preoperative PFT parameters, was the most reliable predictor of PPCs after lobectomy for lung cancer. However, the process of PFT should be checked. For the precise evaluation of PFT, the patient’s effort is essential (14). Therefore, although PFT is an objective means of evaluating pulmonary function, it has the possibility of misinterpretation. Moreover, it is sometimes difficult to understand and perform the entire process of PFT, especially in older patients. Therefore, measurement of skeletal muscle mass may be an alternative method, without requiring any special effort by the patient, to predict PPCs in older patients undergoing lobectomy for lung cancer, regardless of the surgical approach, VATS, or thoracotomy. Considering the patients with PPCs were older than the patients without PPCs, measurement of skeletal muscle mass might be a useful and comfort tool for the elderly patients with lung cancer to predict PPCs after lung cancer surgery. Moreover, the evaluation for PPCs after lung cancer surgery was able to be simultaneously obtained at the first scanning for diagnosis of lung cancer, using CT. It meant that no additional assessment for PPCs after lung cancer surgery was needed.
Psoas muscle has been popularly chosen as a predictor in previous studies for the association between muscle mass and morbidity after major surgeries (15,16). However, psoas muscle is originated from T12 and inserted to femur. Scanning from chest to lower limbs is required for the exact evaluation of psoas muscle and muscle mass at the mid-level of psoas muscle, taken from abdominal scanning, has been used in the previous studies (17,18). Additionally, scanning increased the risk for the exposure of biohazard. Therefore, we thought that psoas muscle was not suitable in the patient undergoing lung cancer surgery, although the usefulness as predictor for morbidity after major surgeries has been demonstrated. We checked the EMs at the level of the spinous process with the 12th thoracic vertebra to measure skeletal muscle mass in the present study. Previous studies have demonstrated the usefulness of the EMs for the evaluation of sarcopenia. Nakano et al. (19) reported that CSA was a useful predictor of prognosis in patients with idiopathic pulmonary fibrosis. Oh et al. (20) reported that CSA was significantly associated with 30-day postoperative major complications in patients undergoing isolated tricuspid valve surgery. Poor clinical outcomes in a previous study were significantly associated with poor preoperative pulmonary function (19). Therefore, the measurement of skeletal muscle mass by examination of the EMs at the level of the spinous process with the 12th thoracic vertebra may be a useful proxy for preoperative pulmonary function.
PPCs after lobectomy for lung cancer are directly associated with respiratory muscles (21). Therefore, respiratory muscles may be better choices for the evaluation of skeletal muscle mass. However, it is necessary to take into account the technical difficulty of the evaluation. Respiratory muscles directly or indirectly affected by lung cancer surgery are associated with changes in respiratory function, which can result in decreases in chest wall compliance and increases in the work of breathing (22). PPCs are the clinical manifestations of functional changes in the respiratory system after lung cancer surgery, although VATS is associated with less damage to the respiratory muscles than thoracotomy. Therefore, preoperative training of respiratory muscles is important to prevent PPCs by increasing the strength of the inspiratory and expiratory muscles in patients undergoing lung cancer surgery (23,24). Using respiratory muscles as a surrogate for the measurement of skeletal muscle mass instead of EMs would be expected to better predict PPCs, but the evaluation would be more technically difficult. It is impossible for simple anatomical measurements of skeletal muscle mass to accurately represent the grade of muscle action or function. However, skeletal muscle mass is positively correlated with muscle strength in individuals above 50 years old, independent of age and sex (25). Therefore, the measurement of skeletal muscle mass based on the EMs in the present study was regarded as a valid surrogate for the physical activity of the patient.
Sarcopenia was defined as CSAs of the specific muscles indexed with body surfaced area less than the median, as in previous studies (20,26). These previous studies evaluated the outcomes after surgical procedures according to the presence or absence of sarcopenia and concluded that sarcopenia was a reliable predictor of clinical outcome after surgical procedures. However, sex and individual physical activity level were not considered in the determination of the cut-off, using the median value of CSAs of the specific muscles indexed to the body surface area in these previous studies. Moreover, a consensus definition of sarcopenia has not been established. The European consensus statement recommendations are based on healthy young adults (27), and were therefore not utilised in this study. However, the evaluation from larger population for association between sarcopenia defined by age, sex, and individual activity and PPCs after lung cancer surgery might be helpful.
In the present study, age, smoking, and DM were shown to be risk factors for PPCs after lobectomy. Age and smoking has been shown previously to be a predictive factor (28,29), but there has been some controversy regarding DM. Komatsu et al. (30) reported that DM did not influence postoperative recovery in the acute phase. Moreover, Wang et al. (31) reported that lung cancer patients with DM had significantly prolonged progression-free survival compared to patients without DM. Although DM led to lower postoperative survival rates in patients with non-small cell lung cancer (30,32,33), the control of glucose, not just binary division of positive or negative for DM diagnosis, may be more clinically meaningful. Therefore, the findings in the present study indicating that DM was a risk factor for PPCs should be re-evaluated in future studies using data regarding the control of glucose. However, we did not have such data in the present study.
Preoperative careful physiologic assessment is essential to identify the patient with risk of PPCs after lung cancer surgery and enable appropriate management immediately after the surgery. Preoperative physiologic assessment includes cardiovascular evaluation, PFT, diffusing capacity of lung for carbon monoxide (DLCO) and so on. The limitations of preoperative PFT were mentioned above, but predictive postoperative (ppo) values of PFT, especially FEV1 (ppoFEV1), or DLCO (ppoDLCO) has been traditionally regarded as the most reliable predictor for PPCs after lung cancer surgery (34,35). However, the process of ppoFEV1 or ppoDLCO is not simple or intuitive because the formula for ppoFEV1 or ppoDLCO requires the number of functional remained segments after lung cancer surgery or normal value of FEV1 or DLCO for individual patient. Although the association with skeletal muscle mass, and ppoFEV1 or ppoDLCO for predictability of PPCs was not evaluated in the present study, it might be helpful to confirm skeletal muscle as a predictor for PPCs.
The present study had several considerations. First, its retrospective study with relatively small sample size from a single centre had inevitable limitation. Important factor for PPCs after lung cancer surgery has the chance to be missed in the retrospective study. For evaluation of the exact association between preoperative PFT, and skeletal muscle mass and PPCs after lung cancer surgery, the patient with previous respiratory disease was excluded. However, it should be reminded that lung cancer had the common pathological mechanism with COPD (36). In the present study, the values of preoperative PFT were expressed by absolute values, not adjusted values by sex, age, height, weight and so on, following the guideline by European Respiratory Society and American Thoracic Society (37), although institutional protocol used equation for Korean population by Choi et al. (38). The guideline showed that the inappropriate selection of equation for reference values leaded misinterpretation and serious problems. Moreover, various equations, not universal equation, has been introduced for Korean population. Therefore, we described absolute values of PFT, not absolute value/reference value (%). Second, the interactions among the events might be existed, although the events of PPCs were defined and listed. Third, CSA of EM was measured by a radiologist and the data for interobserver agreement was not available, although the previous studies also did not have the data (39,40). Fourth, the median of CSABoth in the groups showed largely overlap and it seemed that the possibility of threshold to distinguish the two groups was low.
In conclusion, PPCs in older patients undergoing lobectomy for lung cancer were associated with a lower preoperative FVC, FEV1 and skeletal muscle mass. Skeletal muscle mass, represented by the EMs, was significantly correlated with preoperative PFT. Therefore, skeletal muscle mass may be a useful predictor of PPCs in patients undergoing lobectomy for lung cancer.
Acknowledgments
Funding: This research was supported by the Basic Science Research Program through the National Research Foundation of Korea (NRF) funded by the Ministry of Science, Information & Communication Technology (Grant No. 2021R1A2C1003360).
Footnote
Reporting Checklist: The authors have completed the STARD reporting checklist. Available at https://jtd.amegroups.com/article/view/10.21037/jtd-22-1156/rc
Data Sharing Statement: Available at https://jtd.amegroups.com/article/view/10.21037/jtd-22-1156/dss
Peer Review File: Available at https://jtd.amegroups.com/article/view/10.21037/jtd-22-1156/prf
Conflicts of Interest: All authors have completed the ICMJE uniform disclosure form (available at https://jtd.amegroups.com/article/view/10.21037/jtd-22-1156/coif). The authors have no conflicts of interest to declare.
Ethical Statement: The authors are accountable for all aspects of the work in ensuring that questions related to the accuracy or integrity of any part of the work are appropriately investigated and resolved. The study was conducted in accordance with the Declaration of Helsinki (as revised in 2013) and approved by the Institutional Review Board of Konkuk University Medical Center (approval No. KUMC 202207071). The patient data were obtained from chart reviews, and the requirement for written informed consent from the patients was waived.
Open Access Statement: This is an Open Access article distributed in accordance with the Creative Commons Attribution-NonCommercial-NoDerivs 4.0 International License (CC BY-NC-ND 4.0), which permits the non-commercial replication and distribution of the article with the strict proviso that no changes or edits are made and the original work is properly cited (including links to both the formal publication through the relevant DOI and the license). See: https://creativecommons.org/licenses/by-nc-nd/4.0/.
References
- Lugg ST, Agostini PJ, Tikka T, et al. Long-term impact of developing a postoperative pulmonary complication after lung surgery. Thorax 2016;71:171-6. [Crossref] [PubMed]
- Motono N, Ishikawa M, Iwai S, et al. Individualization of risk factors for postoperative complication after lung cancer surgery: a retrospective study. BMC Surg 2021;21:311. [Crossref] [PubMed]
- Silva de Paula N, de Aguiar Bruno K, Azevedo Aredes M, et al. Sarcopenia and Skeletal Muscle Quality as Predictors of Postoperative Complication and Early Mortality in Gynecologic Cancer. Int J Gynecol Cancer 2018;28:412-20. [Crossref] [PubMed]
- Sun X, Xu J, Chen X, et al. Sarcopenia in Patients With Normal Body Mass Index Is an Independent Predictor for Postoperative Complication and Long-Term Survival in Gastric Cancer. Clin Transl Sci 2021;14:837-46. [Crossref] [PubMed]
- Trejo-Avila M, Bozada-Gutiérrez K, Valenzuela-Salazar C, et al. Sarcopenia predicts worse postoperative outcomes and decreased survival rates in patients with colorectal cancer: a systematic review and meta-analysis. Int J Colorectal Dis 2021;36:1077-96. [Crossref] [PubMed]
- Park CH, Yi Y, Do JG, et al. Relationship between skeletal muscle mass and lung function in Korean adults without clinically apparent lung disease. Medicine (Baltimore) 2018;97:e12281. [Crossref] [PubMed]
- Park HK, Lee SH, Lee SY, et al. Relationships between lung function decline and skeletal muscle and fat mass changes: a longitudinal study in healthy individuals. J Cachexia Sarcopenia Muscle 2021;12:2145-53. [Crossref] [PubMed]
- Clavien PA, Barkun J, de Oliveira ML, et al. The Clavien-Dindo classification of surgical complications: five-year experience. Ann Surg 2009;250:187-96. [Crossref] [PubMed]
- Laursen LØ, Petersen RH, Hansen HJ, et al. Video-assisted thoracoscopic surgery lobectomy for lung cancer is associated with a lower 30-day morbidity compared with lobectomy by thoracotomy. Eur J Cardiothorac Surg 2016;49:870-5. [Crossref] [PubMed]
- Agostini PJ, Lugg ST, Adams K, et al. Risk factors and short-term outcomes of postoperative pulmonary complications after VATS lobectomy. J Cardiothorac Surg 2018;13:28. [Crossref] [PubMed]
- Garzon JC, Ng CS, Sihoe AD, et al. Video-assisted thoracic surgery pulmonary resection for lung cancer in patients with poor lung function. Ann Thorac Surg 2006;81:1996-2003. [Crossref] [PubMed]
- Almquist D, Khanal N, Smith L, et al. Preoperative Pulmonary Function Tests (PFTs) and Outcomes from Resected Early Stage Non-small Cell Lung Cancer (NSCLC). Anticancer Res 2018;38:2903-7. [PubMed]
- Berry MF, Villamizar-Ortiz NR, Tong BC, et al. Pulmonary function tests do not predict pulmonary complications after thoracoscopic lobectomy. Ann Thorac Surg 2010;89:1044-51; discussion 1051-2. [Crossref] [PubMed]
- Lange NE, Mulholland M, Kreider ME. Spirometry: don't blow it! Chest 2009;136:608-14. [Crossref] [PubMed]
- Hawkins RB, Mehaffey JH, Charles EJ, et al. Psoas muscle size predicts risk-adjusted outcomes after surgical aortic valve replacement. Ann Thorac Surg 2018;106:39-45. [Crossref] [PubMed]
- Uemura S, Shichinohe T, Kurashima Y, et al. Effects of preoperative psoas muscle index and body mass index on postoperative outcomes after video-assisted esophagectomy for esophageal cancer. Asian J Endosc Surg 2021;14:739-47. [Crossref] [PubMed]
- So SP, Lee BS, Kim JW. Psoas Muscle Volume as an Opportunistic Diagnostic Tool to Assess Sarcopenia in Patients with Hip Fractures: A Retrospective Cohort Study. J Pers Med 2021;11:1338. [Crossref] [PubMed]
- Yamada Y, Shimada Y, Makino Y, et al. Clinical utility of psoas muscle volume in assessment of sarcopenia in patients with early-stage non-small cell lung cancer. J Cancer Res Clin Oncol 2022; Epub ahead of print. [Crossref] [PubMed]
- Nakano A, Ohkubo H, Taniguchi H, et al. Early decrease in erector spinae muscle area and future risk of mortality in idiopathic pulmonary fibrosis. Sci Rep 2020;10:2312. [Crossref] [PubMed]
- Oh J, Song IK, Nam JS, et al. Sarcopenia as a prognostic factor for outcomes after isolated tricuspid valve surgery. J Cardiol 2020;76:585-92. [Crossref] [PubMed]
- Nomori H, Kobayashi R, Fuyuno G, et al. Preoperative respiratory muscle training. Assessment in thoracic surgery patients with special reference to postoperative pulmonary complications. Chest 1994;105:1782-8. [Crossref] [PubMed]
- Siafakas NM, Mitrouska I, Bouros D, et al. Surgery and the respiratory muscles. Thorax 1999;54:458-65. [Crossref] [PubMed]
- Laurent H, Aubreton S, Galvaing G, et al. Preoperative respiratory muscle endurance training improves ventilatory capacity and prevents pulmonary postoperative complications after lung surgery. Eur J Phys Rehabil Med 2020;56:73-81. [Crossref] [PubMed]
- Kendall F, Oliveira J, Peleteiro B, et al. Inspiratory muscle training is effective to reduce postoperative pulmonary complications and length of hospital stay: a systematic review and meta-analysis. Disabil Rehabil 2018;40:864-82. [Crossref] [PubMed]
- Chen L, Nelson DR, Zhao Y, et al. Relationship between muscle mass and muscle strength, and the impact of comorbidities: a population-based, cross-sectional study of older adults in the United States. BMC Geriatr 2013;13:74. [Crossref] [PubMed]
- Garg L, Agrawal S, Pew T, et al. Psoas Muscle Area as a Predictor of Outcomes in Transcatheter Aortic Valve Implantation. Am J Cardiol 2017;119:457-60. [Crossref] [PubMed]
- Cruz-Jentoft AJ, Bahat G, Bauer J, et al. Sarcopenia: revised European consensus on definition and diagnosis. Age Ageing 2019;48:16-31. [Crossref] [PubMed]
- Qiu B, Ji Y, Zhang F, et al. Outcomes and experience of anatomical partial lobectomy. J Thorac Cardiovasc Surg 2022;164:637-647.e1. [Crossref] [PubMed]
- de Angelis P, Tan KS, Chudgar NP, et al. Operative Time Is Associated with Postoperative Complications After Pulmonary Lobectomy. Ann Surg 2022; Epub ahead of print. [Crossref] [PubMed]
- Komatsu T, Chen-Yoshikawa TF, Ikeda M, et al. Impact of diabetes mellitus on postoperative outcomes in individuals with non-small-cell lung cancer: A retrospective cohort study. PLoS One 2020;15:e0241930. [Crossref] [PubMed]
- Wang NF, Tang HM, Liu FL, et al. Prolonged progression-free survival and overall survival are associated with diabetes mellitus but inversely associated with levels of blood glucose in patients with lung cancer. Chin Med J (Engl) 2020;133:786-91. [Crossref] [PubMed]
- Bi G, Yao G, Bian Y, et al. The Effect of Diabetes Mellitus on Prognosis of Patients with Non-Small-Cell Lung Cancer: A Systematic Review and Meta-Analysis. Ann Thorac Cardiovasc Surg 2020;26:1-12. [Crossref] [PubMed]
- Zhu L, Cao H, Zhang T, et al. The Effect of Diabetes Mellitus on Lung Cancer Prognosis: A PRISMA-compliant Meta-analysis of Cohort Studies. Medicine (Baltimore) 2016;95:e3528. [Crossref] [PubMed]
- Forster C, Hasenauer A, Perentes JY, et al. Is faster better? Impact of operative time on postoperative outcomes after VATS anatomical pulmonary resection. J Thorac Dis 2022;14:1980-9. [Crossref] [PubMed]
- Brunelli A. Preoperative functional workup for patients with advanced lung cancer. J Thorac Dis 2016;8:S840-8. [Crossref] [PubMed]
- Zhang S, Pang K, Feng X, et al. Transcriptomic data exploration of consensus genes and molecular mechanisms between chronic obstructive pulmonary disease and lung adenocarcinoma. Sci Rep 2022;12:13214. [Crossref] [PubMed]
- Stanojevic S, Kaminsky DA, Miller MR, et al. ERS/ATS technical standard on interpretive strategies for routine lung function tests. Eur Respir J 2022;60:2101499. [Crossref] [PubMed]
- Choi JK, Paek D, Lee JO. Normal Predictive values of spirometry in Korean Population. Tuberc Respir Dis (Seoul) 2005;58:230-42. [Crossref]
- Nakada T, Noda Y, Kato D, et al. Risk factors and cancer recurrence associated with postoperative complications after thoracoscopic lobectomy for clinical stage I non-small cell lung cancer. Thorac Cancer 2019;10:1945-52. [Crossref] [PubMed]
- Kawaguchi Y, Hanaoka J, Ohshio Y, et al. Sarcopenia predicts poor postoperative outcome in elderly patients with lung cancer. Gen Thorac Cardiovasc Surg 2019;67:949-54. [Crossref] [PubMed]