This article has an erratum available at: http://dx.doi.org/10.21037/jtd-2023-21 the article has been update on 2023-12-28 at here.
LncRNA HOXD-AS2 regulates miR-3681-5p/DCP1A axis to promote the progression of non-small cell lung cancer
Highlight box
Key findings
• HOXD-AS2 is significantly heightened in NSCLC and it modulates the miR-3681-5p/DCP1A axis to boost the proliferation, migration, and invasion activities of NSCLC cells.
What is known and what is new?
• NSCLC is the most common malignancy in lung cancer, with a low survival rate and unfavorable prognosis. HOXD-AS2 is a demonstrated oncogenic lncRNA in carcinomas.
• The expression profile and molecular mechanism underlying HOXD-AS2 in NSCLC was first uncovered by the present paper.
What is the implication, and what should change now?
• These findings found the basis of HOXD-AS2 as a new diagnostic biomarker and molecular target for NSCLC therapy.
Introduction
Globally, lung cancer is the most common malignancy and the leading cause of cancer mortality, with a low 5-year survival rate of 6–13% (1,2). It can be divided into small cell lung cancer (SCLC; 15%) and non-small cell lung cancer (NSCLC; 85%), NSCLC can be subdivided into large cell lung cancer, lung adenocarcinoma (LUAD) and lung squamous cell carcinoma (LUSC) (3,4). Surgery, immunotherapy, chemotherapy, radiotherapy, and molecular targeted therapy are main treatment approaches for NSCLC (5). Despite recent advances in treatment strategies of NSCLC, its incidence rate remains relatively high (6). Thus, it is imperative to seek the functional molecular mechanisms in NSCLC to determine new therapeutic targets.
Non-coding RNAs (ncRNAs), constituting the majority of the human transcribed genome, are RNA transcripts that functionally mediate cellular processes, containing transcription, chromatin remodelling, and post-transcriptional modifications (7,8). They can be classified into long ncRNAs (lncRNAs), circular RNAs (circRNAs), microRNAs (miRNAs), small nuclear RNAs (snRNAs), and so on (9). LncRNAs, with a length over 200 nt and no protein-coding potential, are differentially expressed and exert pivotal roles in modulating cellular activities in diverse carcinomas (10-12). For examples, lncRNA OXCT1-AS1 could boost proliferation of NSCLC cells through targeting miR-195/CCNE1 axis (13); lncRNA B4GALT1-AS1 accelerates NSCLC growth via elevating ZEB1 level via sponging miR-144-3p (14). Among these lncRNAs, HOXD cluster antisense RNA 2 (HOXD-AS2) is a demonstrated oncogenic lncRNA in human carcinomas. In glioma, HOXD-AS2 is up-regulated and promotes cancer process, which is due to the transcription activation by TFE3 and cytoplasmic degradation by miR-661 (15). HOXD-AS2 also negatively affects the miR-3681-5p level to augment MALT1 expression in glioblastoma (16). Thus, the function and related functional mechanisms underlying HOXD-AS2 in NSCLC still await investigation.
MiRNAs are small ncRNAs post-transcriptionally controlling gene expression upon targeting 3' untranslated regions (3'UTRs) of relative messenger RNAs (mRNAs) (17). Different to lncRNAs, the length of miRNAs is about 20–22 nt (18). MiRNAs display abnormal expression profiles and functions in human diseases, including cancer (19). MiR-106b-5p has been shown to provoke the lung metastasis of breast cancer through targeted inhibition of CNN1 to activate the Rho/ROCK1 pathway (20). In prostate cancer, miR-19a binds with CUL5 to hasten migratory, invasive, and epithelial-mesenchymal transition (EMT) capabilities (21). In this research, miR-3681-5p is shown to be negatively affected by HOXD-AS2, thus augmenting MALT1 expression for glioblastoma aggressiveness (16). However, no studies on the role of miR-3681-5p in NSCLC were conducted.
In the competing endogenous RNA (ceRNA) molecular mechanism, mRNAs commonly function as the downstream targets of miRNAs to influence cellular activities of tumor cells (22,23). Among these genes, mRNA-decapping enzyme 1A (DCP1A) is in close association with cancer progression. DCP1A is highly expressed and related to poor prognosis, downregulation of which can reduce proliferation, migration, and chemotherapeutic resistance (24). Also, overexpression of DCP1A decreases the survival rate of colorectal carcinoma patients (25). In gastric cancer, DCP1A is mediated by the LINC00339/miR-377-3p axis and accelerates tumor progression (26). Similarly, no studies on the role of DCP1A in NSCLC have been reported.
In this paper, we aimed to investigate the role of HOXD-AS2 in NSCLC in another way. We analyzed the expression of HOXD-AS2 in cancer tissues and cells and dissected the relationship between its expression and overall survival (OS) of patients. Functional experiments were conducted to examine the effect of HOXD-AS2 on cell viability, invasion, migration, and apoptosis in NSCLC cells. Thereafter, the binding between miR-3681-5p and HOXD-AS2 or DCP1A was explored via luciferase reporter assay. The regulatory function of HOXD-AS2 on miR-3681-5p or that of miR-3681-5p on DCP1A in NSCLC was measured through rescue experiments. We present the following article in accordance with the ARRIVE and MDAR reporting checklists (available at https://jtd.amegroups.com/article/view/10.21037/jtd-23-153/rc).
Methods
Clinical tissue samples
We collected 5 paired cancerous and adjacent non-tumor tissues from patients pathologically diagnosed with NSCLC at Binhai County People’s Hospital. Tissues were stored at −80 ℃ for subsequent analysis. None of the included patients underwent chemotherapy or radiotherapy prior to tissue collection and all patients provided their informed consents before taking part. The research was approved by the Ethics Committee of Binhai County People’s Hospital (No. 2021BYKYLL006) and conducted in accordance with the Declaration of Helsinki (as revised in 2013).
Cell culture
The cells used in assays were obtained from American Type Culture Collection (ATCC; Manassas, VA, USA) and cultivated in a humid environment with 5% CO2 at 37 ℃. Dulbecco’s modified Eagle medium (DMEM; Gibco, Grand Island, NY, USA) supplemented with 100 µg/mL streptomycin, 10% fetal bovine serum (FBS), and 100 U/mL penicillin (Gibco, USA) was employed for culture of human cancerous H1975 (cat. No. CRL-5908), A549 (cat. No. CCL-185), and H1299 (cat. No. CRL-5803) cells, and the normal lung epithelial cell line BEAS-2B (cat. No. CRL-9609) was used as a control.
Quantitative real-time polymerase chain reaction (qRT-PCR)
RNA extraction was implemented in tissues and cells through application of TRIzol (Invitrogen, Carlsbad, CA, USA), followed by complementary DNA (cDNA) acquisition by a PrimeScript RT reagent Kit with gDNA Eraser (Takara, Dalian, China). qRT-PCR was performed with SYBR Green Master PCR mix (Applied Biosystems, Foster City, CA, USA) on the ABI 7900 system (Applied Biosystems). Expression of HOXD-AS2, CCR1, DCP1A, and PPP3R1 or miR-3681-5p, calculated by 2−ΔΔCt method was normalized to that of GAPDH or U6. Primers for this assay were as follows: HOXD-AS2 forward 5'-AGGAACTGCTCTGGTGAACTCC-3' and reverse 5'-TGGGCATCTCTTTCAGGAAGGT-3'; miR-3681-5p forward 5'-TAGTGGATGATGCACTCTGT-3' and reverse 5'-GAACATGTCTGCGTATCTC-3'; CCR1 forward 5'-GCCTTCTGGTTTTATGGG-3' and reverse 5'-CTCCTAGACACTTTTCCTC-3'; DCP1A forward 5'-CACCCCGGTGCTAATCACTC-3' and reverse 5'-GCTCAACGGGATTGTGTAGGTT-3'; PPP3R1 forward 5'-GAGGGCGTCTCTCAGTTCAG-3' and reverse 5'-GCTGGACGTCTTGAGCAGAT-3'; GAPDH forward 5'-CGCTCTCTGCTCCTCCTGTTC-3' and reverse 5'-ATCCGTTGACTCCGACCTTCAC-3'; U6 forward 5'-CTCGCTTCGGCAGCACA-3' and reverse 5'-ACGCTTCACGAATTTGCGT-3'.
Cell transfection
H1975 and A549 cells were cultured in the incubator at 37 ℃ in a humidified 5% CO2 atmosphere for cell transfection. When cell confluency reached 60–80%, cells were subjected to transfection. All plasmids were from GenePharma (Shanghai, China). HOXD-AS2 downregulation in tumor cells was achieved through treatment with small interfering RNAs (siRNAs) targeting HOXD-AS2 (si-HOXD-AS2), in comparison with control and negative control (si-NC) groups. To explore the role of HOXD-AS2 in vivo, short hairpin RNAs (shRNAs) targeting HOXD-AS2 (sh-HOXD-AS2) and sh-NC lentivirus (LV) were transfected into H1975 cells. Expression of miR-3681-5p was affected by transfection of miR-3681-5p mimic and anti-miR-3681-5p, compared with relative NCs. The sequence of DCP1A was amplified and cloned into a pcDNA3.1 vector to construct DCP1A overexpression vectors (oe-DCP1A), with blank vector as NC. This assay was conducted by Lipofectamine 2000 (Invitrogen) according to the manufacturer’s protocols.
Bioinformatics analysis
A volcano plot was made according to Gene Expression Omnibus (GEO) database GSE138172 to identify differentially expressed genes (DEGs) in NSCLC and non-tumor tissues. Interaction between HOXD-AS1 and miR-3681-5p was predicted through ENCORI (https://starbase.sysu.edu.cn/index.php). Predicted targets of miR-3681-5p by miRDB (https://mirdb.org/), TargetScan (https://www.targetscan.org/vert_80/), and DIANA (http://diana.imis.athena-innovation.gr/DianaTools/index.php) were analyzed to construct a Venn diagram on Venny 2.1.0 (https://bioinfogp.cnb.csic.es/tools/venny/index.html).
3-(4,5-dimethylthiazol-2-yl)-2,5-diphenyltetrazolium bromide (MTT) assay
MTT (Sigma Aldrich, St. Louis, MO, USA) was employed to test cell viability. Cells (4×103 in each well) were seeded into a 96-well culture dish 24 hours post transfection. After removal of the supernatant, 10 µL MTT was added to the well at 0, 24, 48, and 72 hours, followed by 4 hours of incubation. Dimethyl sulfoxide (DMSO) was added and shaken to sufficiently dissolve the purple crystals, and the optical density (OD) value was measured at 490 nm upon a microplate reader (Olympus, Tokyo, Japan).
Flow cytometry analysis
Cell apoptosis of tumor cells was assessed through flow cytometry with assistance of Annexin V-fluorescein isothiocyanate (FITC)/propidium iodide (PI) apoptosis kit (eBioscience; Thermo Fisher Scientific, Waltham, MA, USA) as per the guidance of producers. Collected cells from 6 well plates were centrifuged for 5 minutes and washed with phosphate-buffered saline (PBS), followed by incubation with 5 µL Annexin V-FITC and 5 µL PI for 15 minutes at room temperature. Thereafter, cells were dissected utilizing the BD FACScalibur flow cytometry [Becton, Dickinson, and Co. (BD) Biosciences, Franklin Lakes, NJ, USA].
Transwell experiments
Cell migration and invasion capabilities were explored via transwell experiments through usage of a transwell insert (8-µm pore size, Corning Costar, Corning, NY, USA) without or with Matrigel (Millipore, Bedford, MA, USA). For cell migration, serum-free DMEM was employed for obtaining cell suspension, which was thereafter put in the upper chamber. Medium with 10% FBS was put in the lower chamber. Cells were wiped from the lower surface of the upper chamber with a cotton swab and taken for fixation by 70% methanol and staining with 0.25% crystal violet for 20 minutes at room temperature. An optical microscope (Olympus, Tokyo, Japan) was applied to observe and count cells in 5 random fields. For cell invasion, the insert with Matrigel was employed.
Luciferase reporter assay
To assess the effect of miR-3681-5p on HOXD-AS2, H1975 and A549 cells were co-transfected with NC or miR-3681-5p mimic and the pmirGLO vector (Promega, Madison, WI, USA) with wild type (WT) or mutant type (MUT) sequences of HOXD-AS2. To assess the effect of miR-3681-5p on DCP1A, H1975 and A549 cells were co-transfected with NC or miR-3681-5p mimic and the pmirGLO vector with WT or MUT sequences of DCP1A. After 48 hours of transfection by Lipofectamine (Invitrogen, USA), luciferase activity was estimated utilizing the dual-luciferase reporter assay system (Promega, USA), with Renilla activity as normalization.
Xenograft tumor models
To interrogate the function of HOXD-AS2 in NSCLC in vivo, animal models were established. A total of 10 4-week-old healthy male BALB/c nude mice (D000521) were obtained from Jiangsu Jicui Yaokang Biotechnology Co., Ltd. (GemPharmatech Co. Ltd., Nanjing, China) and kept in specific-pathogen-free conditions with free food and water. After subcutaneous injection of H1975 cells with LV-sh-NC or LV-sh-HOXD-AS2 into the right flank, mice were randomly divided into sh-NC and sh-HOXD-AS2 groups (n=5 in each group). Tumor growth was measured every 3 days using calipers, which was calculated as V = 0.5 × L × W2 (L: length; W: width). Twenty-four days later, mice were euthanized through carbon dioxide (CO2) inhalation and the tumors were removed for measuring weights and taking pictures. Experiments were performed in the laboratory animal center of Nanjing Agricultural University under a project license (No. 2022021) granted by the Ethics Committee of Nanjing Agricultural University, in compliance with institutional guidelines for the care and use of animals. A protocol was prepared before the study without registration.
Western blot
To examine protein expression, isolated specimens were lysed in radioimmunoprecipitation assay (RIPA) lysis buffer (Thermo Fisher Scientific, USA) and its concentration was estimated by bicinchoninic acid (BCA) kit (Thermo Fisher Scientific). A total of 20 µg of protein samples were thereafter isolated on 10% sodium dodecyl sulfate polyacrylamide gel electrophoresis (SDS-PAGE), followed by transferring onto polyvinylidene fluoride (PVDF) membranes (Millipore, Bedford, MA, USA). The membranes were subjected to blockade by 5% nonfat milk and cultivated overnight at 4 ℃. Primary antibodies against DCP1A (22373-1-AP; 1:2,000; Proteintech, Rosemont, IL, USA) and GAPDH (ab8245; 1:500; Abcam, Cambridge, MA, USA) were employed. Thereafter, horseradish peroxidase (HRP)-tagged secondary antibody was applied to culture the membranes for another 1 hour. Blots were viewed with an enhanced chemiluminescence (ECL) kit (Pierce, Rockford, IL, USA), normalized to the internal reference GAPDH.
Hematoxylin and eosin (HE) staining
Obtained lung tissues were fixed by formalin, embedded in paraffin, and sectioned as 4 µm. Subsequently, sections were stained utilizing HE solution after deparaffinage. Histopathological characteristics were viewed using a light microscope (Leica Microsystems, Wetzlar, Germany).
Immunohistochemistry (IHC)
We soaked tissues in ethanol and rinsed them using PBS. Thereafter, samples were cultivated with a permeable solution with 3% H2O2 for another 10 minutes. We prepared 5% goat serum blocking solution for incubation for nearly 20 minutes. Antibody against Ki-67 (ab92742; 1:1,000; Abcam) was utilized for dilution and added to the sample at 4 ℃ overnight. After that, HRP-labelled anti-rabbit secondary antibody was applied for culture for 1 hour. Slides were developed with 3,3'-diaminobenzidine (DAB) and dyed utilizing hematoxylin, followed by observation under a microscope.
Statistical analysis
Data gained from triplicated tests were dissected with SPSS 20.0 (IBM Corp., Armonk, NY, USA) and presented as mean ± standard deviation (SD) with a 95% confidence interval (CI). A P value below 0.05 was considered statistically significant. Differences of two or multiple groups were compared using Student’s t-test and one-way analysis of variance (ANOVA). Kaplan-Meier analysis was used to measure the correlation between HOXD-AS2 expression and OS of cancer patients.
Results
HOXD-AS2 was upregulated in NSCLC and predicted poor prognosis
The GSE138172 dataset was analyzed via volcano plots to identify DEGs in NSCLC (Figure 1A). Among these genes, lncRNA HOXD-AS2 is significantly overexpressed in NSCLC. Then, we tested its expression in 5 pairs of NSCLC and non-tumor tissues. The results of qRT-PCR demonstrated that HOXD-AS2 exhibited obviously high expression in NSCLC tissues (Figure 1B). Kaplan-Meier analysis was performed for exploring the relation between HOXD-AS2 expression and OS, which revealed that high HOXD-AS2 expression predicted low OS rate (Figure 1C). The qRT-PCR assay indicated that HOXD-AS2 expression was relatively heightened in NSCLC cells containing H1975, A549, and H1299, compared with normal pulmonary epithelial BEAS-2B cells (Figure 1D). The H1975 and A549 cells with higher expression of HOXD-AS2 were chosen for subsequent assays. Here, it was clear that HOXD-AS2 exhibited high expression in NSCLC tissues and cells and predicted worse prognosis.
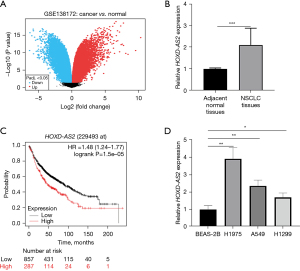
HOXD-AS2 silencing impeded proliferation, migration, and invasion but induced apoptosis in NSCLC
To assess the function of HOXD-AS2 in NSCLC, we applied si-HOXD-AS2 to downregulate HOXD-AS2 expression. The results of qRT-PCR disclosed that in H1975 and A549 cells, HOXD-AS2 expression was dramatically silenced by si-HOXD-AS2 transfection (Figure 2A). Through MTT assay, we observed that downregulation of HOXD-AS2 significantly repressed cell viability in comparison with control and si-NC groups (Figure 2B). The results of transwell assays presented that cell migration and invasion were distinctly inhibited when HOXD-AS2 expression was downregulated (Figure 2C). Moreover, flow cytometry exhibited that cell apoptosis was overtly activated under transfection of si-HOXD-AS2 in H1975 and A549 cells (Figure 2D). Taken together, inhibition of HOXD-AS2 hindered proliferation, migration, and invasion but activated apoptosis in NSCLC cells.
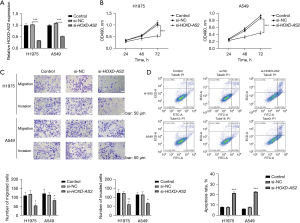
HOXD-AS2 sponged miR-3681-5p to regulate cellular activities in NSCLC cells
To explore the molecular mechanism under HOXD-AS2 in NSCLC, we browsed starBase and discovered the binding between HOXD-AS2 and miR-3681-5p. The binding sequences were presented in Figure 3A. Then, luciferase reporter assay was utilized to affirm the interaction, in which an obvious decrease in luciferase activity of HOXD-AS2-WT was observed (Figure 3B). In NSCLC tissues, miR-3681-5p expression was distinctly decreased relative to normal tissues, as estimated by qRT-PCR (Figure 3C). Significantly low expression of miR-3681-5p was observed in H1975 and A549 cells, compared with BEAS-2B cells (Figure 3D). The qRT-PCR assay presented that HOXD-AS2 silencing could upregulate miR-3681-5p expression, which was reduced under transfection of anti-miR-3681-5p (Figure 3E). The results of MTT assay demonstrated that cell viability was significantly repressed when HOXD-AS2 was downregulated, which was strengthened when miR-3681-5p expression was inhibited (Figure 3F). Transwell experiments also represented that HOXD-AS2 repression overtly hindered cell migration and invasion and the inhibition was restored when miR-3681-5p expression was reduced (Figure 3G and Figure S1A). In flow cytometry, HOXD-AS2 decrease-activated cell apoptosis was distinctly restricted under miR-3681-5p reduction (Figure 3H and Figure S1B). In summary, HOXD-AS2 sponged miR-3681-5p to mediate cellular activities in NSCLC cells.
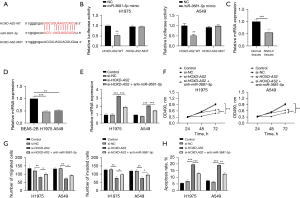
MiR-3681-5p targeted DCP1A to boost NSCLC proliferation, migration, and invasion
A Venn diagram was drawn to identify the downstream targets of miR-3681-5p. Data from miRDB, TargetScan, and DIANA were analyzed and eventually, 3 targets including CCR1, DCP1A, and PPP3R1 were screened (Figure 4A). After overexpression of miR-3681-5p, only DCP1A exhibited dramatically reduced expression in H1975 and A549 cells (Figure 4B). Thus, DCP1A was the predicted target of miR-3681-5p in NSCLC. The predicted binding sites between miR-3681-5p and DCP1A were shown in Figure 4C. Subsequent luciferase reporter experiments showed that miR-3681-5p bound to DCP1A (Figure 4D). Functional assays were also performed in H1975 and A549 cells to explore the function of miR-3681-5p/DCP1A axis in NSCLC. The transfection efficiency of oe-DCP1A was affirmed through qRT-PCR (Figure 4E). In the MTT assay, cell viability was significantly restricted by miR-3681-5p upregulation, which was partly recovered by increase of DCP1A (Figure 4F). Transwell experiments uncovered that miR-3681-5p elevation-restrained migration and invasion was rescued when DCP1A expression was obviously increased (Figure 4G and Figure S2A). Overexpression of miR-3681-5p obviously promoted apoptosis and the phenomenon was significantly suppressed with increase of DCP1A (Figure 4H and Figure S2B). Hence, miR-3681-5p targeted DCP1A, thus facilitating NSCLC proliferation, migration, and invasion.
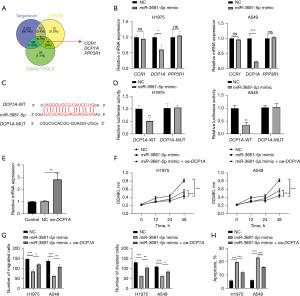
Inhibition of HOXD-AS2 hampered NSCLC tumor growth in vivo
Animal models were established to investigate the role of HOXD-AS2 in vivo. H1975 cells subjected to lentiviral transfection with sh-NC or sh-HOXD-AS2 were injected subcutaneously into nude mice. The recorded tumor volumes were displayed in Figure 5A, which indicated the inhibitory effect of HOXD-AS2 silencing on tumor volume. Tumor weights and sizes were also decreased when HOXD-AS2 was downregulated (Figure 5B,5C). We also used qRT-PCR and Western blot to test miR-3681-5p and DCP1A in tumor tissues and the results illustrated that miR-3681-5p was dramatically elevated and DCP1A protein expression was significantly decreased when HOXD-AS2 was reduced (Figure 5D,5E). As exhibited in Figure 5F, HE staining results indicated decreased tissue damage under HOXD-AS2 downregulation. According to the results of IHC, Ki-67 expression was obviously reduced with HOXD-AS2 downregulation. Thus, HOXD-AS2 silencing could inhibit NSCLC tumor growth in vivo.
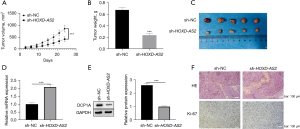
Discussion
In ceRNA networks, lncRNAs competitively bind to miRNAs to cross-modulate each other, hence mediating downstream target genes of miRNAs at post-transcription level (27,28). In this regulatory mechanism, lncRNAs act as miRNA sponges via miRNA response elements (MREs) in the cytoplasm (29). Previously, lncRNAs-based ceRNA networks have been illustrated in different tumors. For examples, lncRNA DANCR accelerates proliferation and metastasis of pancreatic cancer cells via modulation of miR-33b/matrix metalloproteinase 16 (MMP16) expression (30); lncRNA SNHG16 increases vascular endothelial growth factor (VEGF) levels in lung cancer upon its interaction with miR-520, and enhances the migration ability (31); in gallbladder cancer, PVT1 mediates HK2 expression via competitive binding with miR-143 (32). In this paper, we observed the dramatically high expression of HOXD-AS2 in NSCLC tissues compared with normal tissues based on GSE138172 results. In view of the tumor-promoting role of HOXD-AS2 in glioma (15,33), we hypothesized that HOXD-AS2 may also facilitate the progression of NSCLC. Hence, HOXD-AS2 was chosen for research. Consistent with previous results, HOXD-AS2 expression in NSCLC tissues and cells was relatively higher than that in normal tissues and cells. Moreover, NSCLC patients with high expression of HOXD-AS2 exhibited short OS rate. Functional assays also affirmed that HOXD-AS2 silencing significantly repressed cell viability, migration, invasion, and activated apoptosis of H1975 and A549 cells.
To clarify the molecular regulation mechanism of HOXD-AS2 in NSCLC in detail, we browsed online and searched for the potential miRNAs binding with HOXD-AS2, among which miR-3681-5p attracted our attention. Previously, miR-3681-5p has only been demonstrated to interact with and be negatively mediated by HOXD-AS2 to elevate MALT1 expression in glioblastoma (16). Hence, we supposed that miR-3681-5p might involve in the regulation of HOXD-AS2 on NSCLC. Our findings validated that HOXD-AS2 bound with miR-3681-5p. The expression of miR-3681-5p was distinctly downregulated in NSCLC tissues and cells, and was negatively related with HOXD-AS2 level. The results of rescue assays unveiled that miR-3681-5p downregulation could abrogate the inhibitory effect of HOXD-AS2 repression on cellular activities of H1975 and A549 cells. Herein, miR-3681-5p and its involvement in HOXD-AS2 regulation were firstly explored in NSCLC.
Subsequently, we applied the TargetScan, miRDB, and DIANA websites to analyze the predicted targets of miR-3681-5p. The Venn diagram displayed 3 common targets, among which merely DCP1A exhibited an obvious decrease under miR-3681-5p overexpression. Ruan et al. has demonstrated that heightened DCP1A is associated with poor prognosis, and its downregulation decreases proliferation, migration, and chemotherapy resistance (24). In gastric cancer, DCP1A has been affirmed to be controlled by the LINC00339/miR-377-3p pathway and to accelerate tumor progression (26). In this study, DCP1A was affirmed to be targeted by miR-3681-5p and its overexpression could reverse the suppressed viability, migration, and invasion of NSCLC cells caused by miR-3681-5p promotion. The role of DCP1A and its related upstream mechanism in NSCLC was revealed for the first time.
In summary, these results indicated that HOXD-AS2 exerts a promoting effect on proliferation, migration, and invasion through regulation of the miR-3681-5p/DCP1A axis in NSCLC, hinting the role of HOXD-AS2 as an optional molecular target and diagnostic marker for further treatment and prognosis of NSCLC patients. However, the limitations of this study cannot be ignored. The diagnostic marker role of HOXD-AS2 in NSCLC needs to be verified with more clinical samples. Functional experiments on other cellular activities were not performed. In future research, we will attempt to deeply study the potential relationships between HOXD-AS2 and EMT, radioresistance, chemoresistance and ultimately recurrence, and its potential as a biomarker for patient risk stratification and local regional metastasis in NSCLC. For clinical studies, more NSCLC specimens and characteristics of NSCLC patients should be collected for analyzing the clinical application of lncRNA. For animal models, OS and the relationship with studied genes will also be explored.
Conclusions
HOXD-AS2 was upregulated in NSCLC tissues and cells, and its high expression predicted shorter OS rate. In NSCLC, HOXD-AS2, exerting its function via targeting miR-3681-5p to modulate DCP1A, could regulate the proliferation, migration, and invasion abilities of cells in vitro and in vivo.
Acknowledgments
Funding: None.
Footnote
Reporting Checklist: The authors have completed the ARRIVE and MDAR reporting checklists. Available at https://jtd.amegroups.com/article/view/10.21037/jtd-23-153/rc
Data Sharing Statement: Available at https://jtd.amegroups.com/article/view/10.21037/jtd-23-153/dss
Peer Review File: Available at https://jtd.amegroups.com/article/view/10.21037/jtd-23-153/prf
Conflicts of Interest: Both authors have completed the ICMJE uniform disclosure form (available at https://jtd.amegroups.com/article/view/10.21037/jtd-23-153/coif). The authors have no conflicts of interest to declare.
Ethical Statement: The authors are accountable for all aspects of the work in ensuring that questions related to the accuracy or integrity of any part of the work are appropriately investigated and resolved. Animal experiments were performed under a project license (No. 2022021) granted by the Ethics Committee of Nanjing Agricultural University, in compliance with institutional guidelines for the care and use of animals. Experiments involving human participants who provided signed informed consent before taking part were approved by the Ethics Committee of Binhai County People’s Hospital (No. 2021BYKYLL006) and were conducted in accordance with the Declaration of Helsinki (as revised in 2013).
Open Access Statement: This is an Open Access article distributed in accordance with the Creative Commons Attribution-NonCommercial-NoDerivs 4.0 International License (CC BY-NC-ND 4.0), which permits the non-commercial replication and distribution of the article with the strict proviso that no changes or edits are made and the original work is properly cited (including links to both the formal publication through the relevant DOI and the license). See: https://creativecommons.org/licenses/by-nc-nd/4.0/.
References
- Baran K, Brzeziańska-Lasota E. Proteomic biomarkers of non-small cell lung cancer patients. Adv Respir Med 2021;89:419-26. [Crossref] [PubMed]
- He T, Cao J, Xu J, et al. Minimally Invasive Therapies for Early Stage Non-small Cell Lung Cancer. Zhongguo Fei Ai Za Zhi 2020;23:479-86. [PubMed]
- Smolle E, Leithner K, Olschewski H. Oncogene addiction and tumor mutational burden in non-small-cell lung cancer: Clinical significance and limitations. Thorac Cancer 2020;11:205-15. [Crossref] [PubMed]
- Travis WD, Brambilla E, Nicholson AG, et al. The 2015 World Health Organization Classification of Lung Tumors: Impact of Genetic, Clinical and Radiologic Advances Since the 2004 Classification. J Thorac Oncol 2015;10:1243-60. [Crossref] [PubMed]
- Alexander M, Kim SY, Cheng H. Update 2020: Management of Non-Small Cell Lung Cancer. Lung 2020;198:897-907. [Crossref] [PubMed]
- Miller KD, Siegel RL, Lin CC, et al. Cancer treatment and survivorship statistics, 2016. CA Cancer J Clin 2016;66:271-89. [Crossref] [PubMed]
- Anastasiadou E, Jacob LS, Slack FJ. Non-coding RNA networks in cancer. Nat Rev Cancer 2018;18:5-18. [Crossref] [PubMed]
- Chan JJ, Tay Y. Noncoding RNA:RNA Regulatory Networks in Cancer. Int J Mol Sci 2018;19:1310. [Crossref] [PubMed]
- Wilkes MC, Repellin CE, Sakamoto KM. Beyond mRNA: The role of non-coding RNAs in normal and aberrant hematopoiesis. Mol Genet Metab 2017;122:28-38. [Crossref] [PubMed]
- Bhan A, Soleimani M, Mandal SS. Long Noncoding RNA and Cancer: A New Paradigm. Cancer Res 2017;77:3965-81. [Crossref] [PubMed]
- Zhang W, Wang Q, Yang Y, et al. The role of exosomal lncRNAs in cancer biology and clinical management. Exp Mol Med 2021;53:1669-73. [Crossref] [PubMed]
- Gupta S, Hashimoto RF. Dynamical Analysis of a Boolean Network Model of the Oncogene Role of lncRNA ANRIL and lncRNA UFC1 in Non-Small Cell Lung Cancer. Biomolecules 2022;12:420. [Crossref] [PubMed]
- Wang D, Chen Y, Song X, et al. LncRNA OXCT1-AS1 promotes the proliferation of non-small cell lung cancer cells by targeting the miR-195/CCNE1 axis. Transl Cancer Res 2022;11:1255-68. [Crossref] [PubMed]
- Liu SW, Yang P, Li FN, et al. LncRNA B4GALT1-AS1 promotes non-small cell lung cancer cell growth via increasing ZEB1 level by sponging miR-144-3p. Transl Cancer Res 2022;11:538-47. [Crossref] [PubMed]
- Qin Y, Qi Y, Zhang X, et al. Production and Stabilization of Specific Upregulated Long Noncoding RNA HOXD-AS2 in Glioblastomas Are Mediated by TFE3 and miR-661, Respectively. Int J Mol Sci 2022;23:2828. [Crossref] [PubMed]
- Zhong X, Cai Y. Long non-coding RNA (lncRNA) HOXD-AS2 promotes glioblastoma cell proliferation, migration and invasion by regulating the miR-3681-5p/MALT1 signaling pathway. Bioengineered 2021;12:9113-27. [Crossref] [PubMed]
- Hussen BM, Hidayat HJ, Salihi A, et al. MicroRNA: A signature for cancer progression. Biomed Pharmacother 2021;138:111528. [Crossref] [PubMed]
- Braicu C, Cojocneanu-Petric R, Chira S, et al. Clinical and pathological implications of miRNA in bladder cancer. Int J Nanomedicine 2015;10:791-800. [Crossref] [PubMed]
- Li M, Li J, Ding X, et al. microRNA and cancer. AAPS J 2010;12:309-17. [Crossref] [PubMed]
- Wang Z, Li TE, Chen M, et al. miR-106b-5p contributes to the lung metastasis of breast cancer via targeting CNN1 and regulating Rho/ROCK1 pathway. Aging (Albany NY) 2020;12:1867-87. [Crossref] [PubMed]
- Wang Y, Hu J, Qi G, et al. miR-19a promotes the metastasis and EMT through CUL5 in prostate cancer cell line PC3. J BUON 2020;25:2028-35. [PubMed]
- Zhao Y, Xu L, Wang X, et al. A novel prognostic mRNA/miRNA signature for esophageal cancer and its immune landscape in cancer progression. Mol Oncol 2021;15:1088-109. [Crossref] [PubMed]
- Wang W, Lou W, Ding B, et al. A novel mRNA-miRNA-lncRNA competing endogenous RNA triple sub-network associated with prognosis of pancreatic cancer. Aging (Albany NY) 2019;11:2610-27. [Crossref] [PubMed]
- Ruan T, Zhang Y, Liu W, et al. Expression of DCP1a in gastric cancer and its biological function and mechanism in chemotherapy resistance in gastric cancer cells. Dig Liver Dis 2020;52:1351-8. [Crossref] [PubMed]
- Wu C, Liu W, Ruan T, et al. Overexpression of mRNA-decapping enzyme 1a affects survival rate in colorectal carcinoma. Oncol Lett 2018;16:1095-100. [Crossref] [PubMed]
- Shi C, Liu T, Chi J, et al. LINC00339 promotes gastric cancer progression by elevating DCP1A expression via inhibiting miR-377-3p. J Cell Physiol 2019;234:23667-74. [Crossref] [PubMed]
- Karreth FA, Pandolfi PP. ceRNA cross-talk in cancer: when ce-bling rivalries go awry. Cancer Discov 2013;3:1113-21. [Crossref] [PubMed]
- Braga EA, Fridman MV, Moscovtsev AA, et al. LncRNAs in Ovarian Cancer Progression, Metastasis, and Main Pathways: ceRNA and Alternative Mechanisms. Int J Mol Sci 2020;21:8855. [Crossref] [PubMed]
- Das S, Ghosal S, Sen R, et al. lnCeDB: database of human long noncoding RNA acting as competing endogenous RNA. PLoS One 2014;9:e98965. [Crossref] [PubMed]
- Luo Y, Wang Q, Teng L, et al. LncRNA DANCR promotes proliferation and metastasis in pancreatic cancer by regulating miRNA-33b. FEBS Open Bio 2020;10:18-27. [Crossref] [PubMed]
- Chen L, Qiu CH, Chen Y, et al. LncRNA SNHG16 drives proliferation, migration, and invasion of lung cancer cell through modulation of miR-520/VEGF axis. Eur Rev Med Pharmacol Sci 2020;24:9522-31. [PubMed]
- Chen J, Yu Y, Li H, et al. Long non-coding RNA PVT1 promotes tumor progression by regulating the miR-143/HK2 axis in gallbladder cancer. Mol Cancer 2019;18:33. [Crossref] [PubMed]
- Nie E, Jin X, Miao F, et al. TGF-β1 modulates temozolomide resistance in glioblastoma via altered microRNA processing and elevated MGMT. Neuro Oncol 2021;23:435-46. [Crossref] [PubMed]
(English Language Editor: J. Jones)